, where V = volume, n = number of moles, R = gas constant, T = temperature, and P = pressure.
Consider the liquid state of water: according to the Ideal Gas law, one gram of water at atmospheric pressure and room temperature should occupy 1358 cubic centimeters (1.358 L); i.e., if P = 1 atm, n = (1 g)(1 mol water/18 g water) = 0.05555 moles of water, R = 0.082 L.atm.mole–1deg–1, T = 298 K (25°C), and V = 1.358 L (1358 cm3).
Yet we know this to be untrue. One gram of water under these conditions occupies approximately one cubic centimeter at room temperature. This type of deviation occurs with many liquids. Why is there such deviation from the Ideal Gas law? For water, it can be explained by understanding the strong intermolecular forces that exist between water molecules and their intramolecular origins. The presence or absence of intermolecular interactions affects not only water, but also all of the liquid substances important for pharmaceuticals. A better way to phrase the last statement is: the magnitude of intermolecular interactions affects not only water, but also all of the liquid substances important for pharmaceuticals, because intermolecular interactions always exist.
Adhesive and cohesive interactions
The fact that water is soluble in itself perhaps is not surprising, as may be the abilities of some other liquids to freely mix – or be miscible with – water. Without thinking further, the maxim ‘like dissolves like’ could suffice as an explanation. For example, many alcohols and water spontaneously combine to form homogeneous mixtures. But why do some liquids, such as oils, generally not mix with – or are immiscible with – water? Mixing mineral oil with water results in the separation of the two liquids, with the oil floating on top of the water. Many liquids spontaneously mix with water, while others spontaneously separate from it. The tendency for two liquids to be miscible is determined by the relative magnitude of cohesive and adhesive attractions among similar and dissimilar molecules. Cohesive interactions are those between molecules of the same kind (such as water with water). Adhesive forces are those between molecules of different kinds (such as ethanol and water). The net result of these forces, for all the involved molecules, dictates the extent to which the two liquids will interact and mix (Figure 1.1).
Cohesive forces are those between like molecules, such as water with water, ethanol with ethanol, and mineral oil with mineral oil (Figure 1.2).
Key Point
Cohesive forces are those between like molecules. Adhesive forces are those between different types of molecules.
Figure 1.2 Examples of materials that interact via cohesive forces
Adhesive forces are those between different kinds of molecules (Figure 1.3).
Figure 1.3 Examples of materials that interact via adhesive forces
If two liquids are mixed together and the sum of the cohesive forces is greater than the sum of their adhesive forces, the liquids will tend to stay in separate phases (such as oil floating on water). If the adhesive forces are stronger than the cohesive forces, the two liquids will tend to mix, to some degree. This ‘degree’ is a measurement known as solubility. Can solubility be predicted? The answer is yes. There are reference tables, such as Table 1.1, for many combinations to obviate the need for many calculations and provide information without needing to experiment.
When a solute dissolves in a solvent, adhesive forces have, at least to some degree, overcome cohesive forces (Figure 1.4).
Water is represented by squares, mineral oil by circles, and ethanol by triangles. In the first frame (A), the cohesive intermolecular interactions (between mineral oil molecules) are stronger than the adhesive interactions (between mineral oil and water). These liquids will be relatively insoluble in each other. In the second frame (B), adhesive intermolecular interactions (between ethanol and water) are in the same range as the cohesive interactions (ethanol–ethanol and water–water). These liquids will tend to be soluble in each other. In the third frame (C), if all three were capable of overcoming cohesion, the three could be soluble in one another, and the intermolecular interactions could be visualized as indicated.
Figure 1.4 Illustration of solubility – adhesive forces overcoming cohesive forces
This is a conceptual illustration of cohesive and adhesive forces among three different liquids. An example of solubility using mineral oil (squares), ethanol (triangles) and water (circles). If either two, or all three, were able to dissolve in one another, this would indicate adhesive forces (solid arrows) were greater than cohesive forces (dashed lines). (A) Water and mineral oil molecules naturally attract to themselves (cohesive interactions), but not to each other (adhesive interactions), and so do not readily mix. (B) Ethanol and mineral oil have cohesive attraction, but also enough adhesive attraction that the two liquids will mix. (C) The three separate entities have some degree of solubility in each other.
An important intermolecular property is polarity, or the tendency to form dipole moments. Recall that the dipole moment of a molecule (µ) is the net polarity, or charge separation, within the molecule, and is represented by the equation µ = Q × r, where µ is the molecule’s polarity, Q is the magnitude of the charge (+ or −) at either end of the molecule, and r is the distance separating the charge centers. When the average positions of an atom’s or molecule’s positive and negative centers coincide, the atom or molecule is neutral, or nonpolar as illustrated in Figure 1.5.
Figure 1.5 Polarity of the atom versus the molecule
(A) When considered at close range, atoms are composed of regions that differ with respect to charge. (B) The nucleus is positively charged, while electrons are negatively charged. When considered as a single entity, the atom overall is neutrally charged, with equal amounts of positive and negative charge ‘cancelling’ each other. Under certain conditions, most commonly when atoms are grouped and covalently bound as molecules, atoms may be ionized. More subtly, however, is the ability of molecules to form regions of charge. Though the molecule as a whole may remain neutral, if considered alone, a region may be neutral, or charged, and thus possess polar and nonpolar regions.
Key Point
An atom or molecule is polar when the average position of its electrons is asymmetrically distributed.
Nonpolar molecules have no net dipole moment. Unless influenced by other forces, neutral molecules are incapable of substantial intermolecular interactions. Nonpolar molecules have symmetry with regard to their charge dispersion. Polar molecules have a permanent asymmetry with regard to their charge dispersion (Figure 1.6). However, nonpolar molecules can have their charge symmetry disrupted through the influences of surrounding molecules. Therefore, the key to molecular interactions is a separation of the positive and negative centers – a dipole – even if ever so briefly. Though it is relative, it should be noted that the charge that is mobile is the negative charge (electrons), and so dipole moments are the result of the movement of electrons.
Key Point
The key to molecular interactions is the separation of positive and negative centers of charge to create a dipole.
Figure 1.6 Net dipole moment
Within this conceptual molecule are two atoms (X and Y). Y is more electronegative than X, and so electrons preferentially occupy the region around Y more than around X, creating a dipole moment.
The presence of a dipole may be a native condition of molecules, or a dipole may occur in an otherwise nonpolar molecule. The latter is fittingly called induction. A permanent dipole arises when the atoms of the molecule do not equally share the electrons of a covalently bonded molecule. This condition occurs when there are differences in electronegativity of the component atoms.
From Table 1.2, it can be observed that when comparing the electronegativities of some selected atoms from higher to lower values, we obtain F (4.1) > O (3.5) > Cl (3.0), N (3.0) > C (2.5) and H (2.2) in order of decreasing electronegativity. Recall that, moving toward the right in the Periodic Table of the Elements, atomic radii decrease, ionization energies increase, and electronegativities increase. The electronegativity of component atoms of a molecule influences the polarity of molecules. When molecules have component atoms with disparate electronegativity values, they are often polar (Figure 1.7). An exception can occur if a molecule has three-dimensional symmetry, with a net dipole moment of zero. Molecules such as hydrochloric acid have permanent dipoles and are therefore ‘polar.’
Table 1.2 Approximate electronegativity of selected elements
Figure 1.7 Polar elements and polar molecules
When considering molecules, as they are collections of atoms, charge can be symmetrically distributed, or it can be unbalanced. Unbalancing occurs either when two or more atoms of differing electronegativity are bonded, or it can occur when an otherwise neutral molecule has its charge induced to move to an asymmetric conformation. The charge that is actually movable is, of course, the negative charge.
Molecules of nitrogen have no difference in electronegativity, no permanent dipole, and so are considered ‘nonpolar.’ Why is water polar? If drawn in a linear fashion, it would appear symmetrical (H–O–H) and that it should have no net dipole moment. First, hydrogen and oxygen differ in electronegativity. This alone would dictate water should have a net dipole moment of zero though – if it were indeed linear. However, the bond angle between the two hydrogens in water molecules is 104.5°, creating nonlinear molecules. Since water molecules are not linear, and there is electrostatic disparity between oxygens and hydrogens, they are able to possess a net dipole moment (Figure 1.8).
Carbon tetrachloride is also symmetrical when drawn in a linear fashion. However, in its three-dimensional conformation it truly is symmetrical, has no net dipole moment, and so is nonpolar, even though carbon and chlorine differ in electronegativity. It is the lack of an overall (net) dipole moment that causes carbon tetrachloride to be nonpolar. Nonpolar molecules do have the capacity to be polarized, perhaps briefly, by the external influences of neighboring molecules. This phenomenon is called an induced dipole, or polarization, and will be discussed below.
Figure 1.8 Bond angle and polarity
Since water takes on a 104.5° angle among the three atoms, a molecule that would otherwise have no net dipole moment possesses one. Carbon tetrachloride has no three-dimensional asymmetry, and so no net dipole moment.
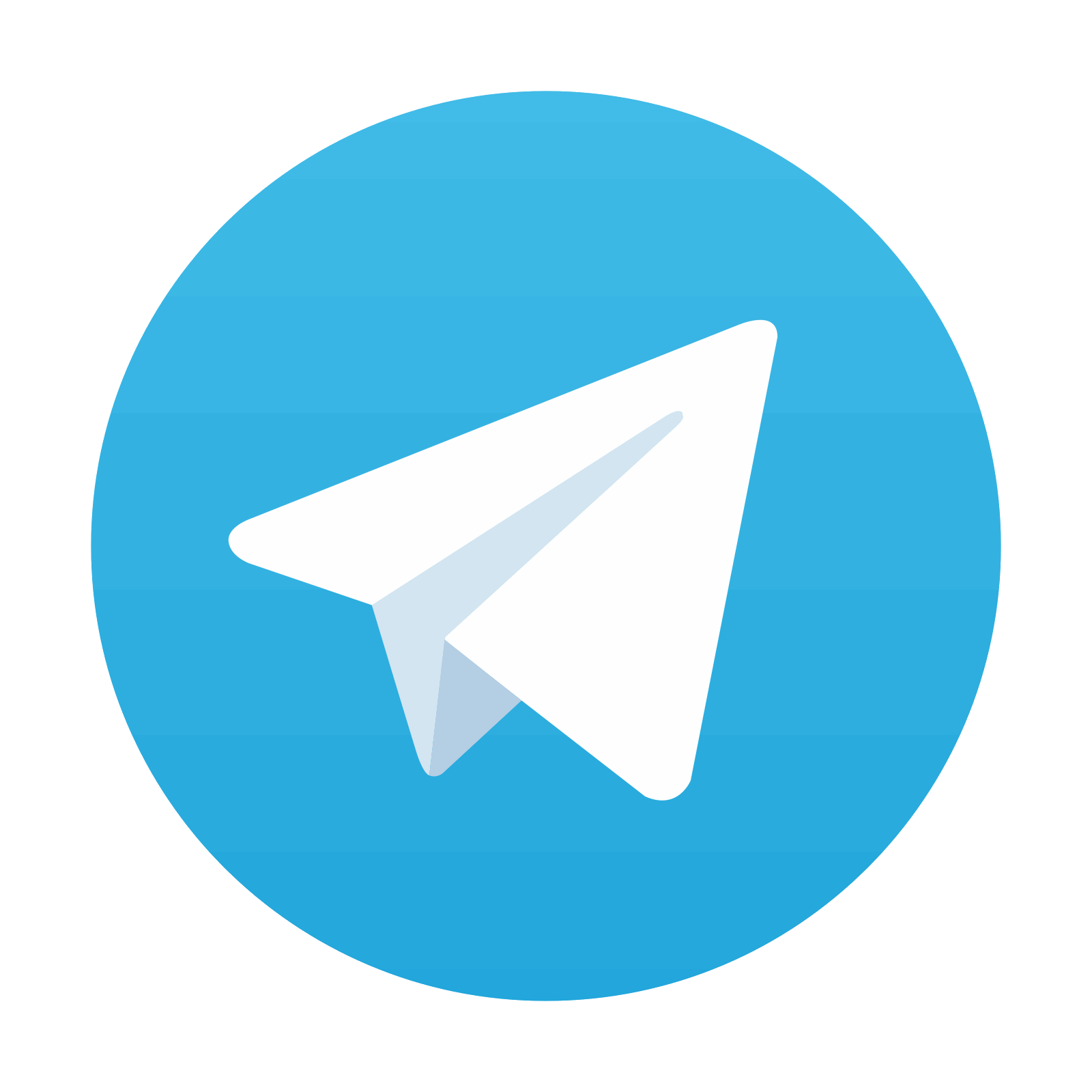
Stay updated, free articles. Join our Telegram channel

Full access? Get Clinical Tree
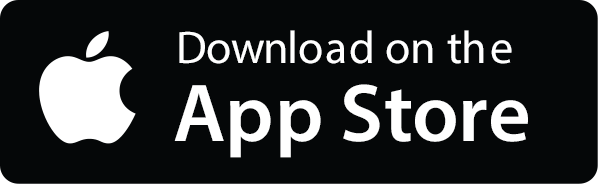
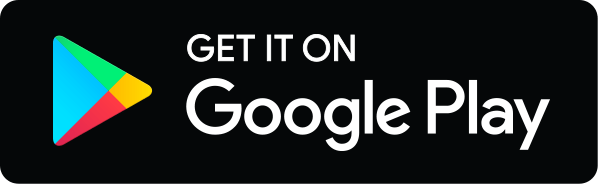