Inflammation and Immunity
Jacquelyn L. Banasik
Key Questions
• What are the major organs and cellular components of the body’s defense against foreign antigens?
• How do immune cells communicate through cell-to-cell interactions and through secreted cytokines?
• How do innate and adaptive immune mechanisms differ?
• What is the role of MHC class I and II proteins in cell- mediated immunity?
http://evolve.elsevier.com/Copstead/
The immune system is a complex network of cells and tissues that work together to protect the body against foreign invaders. The wide variety of potential pathogens requires a defense system that is diversified and adaptable. Several types of white blood cells (WBCs) are of primary importance in localizing, recognizing, and eliminating foreign substances. These immune cells are strategically situated in diverse locations so that pathogens may be detected quickly. The dispersed nature of these defensive cells necessitates a complex system of intercellular communication to effectively mobilize reinforcements to areas of need. A tremendous amount of information has accumulated about how immune cells communicate and the processes that enable them to migrate to particular locations. The impact of this research goes far beyond the traditional immune disorders such as immunodeficiency diseases and hypersensitivity reactions. The immune system has been implicated in the pathogenesis of disorders as diverse as atherosclerosis, myocardial infarction, shock, diabetes, and stroke. Therefore, an understanding of immune function is fundamental to the study of a wide variety of diseases. This chapter describes the organs and cells that constitute the immune system, the mechanisms of action of innate and adaptive defenses, and the communication processes whereby immune cells achieve a coordinated response. Underreactions and overreactions of the immune system, immune system malignancies, and human immunodeficiency virus disease are described in Chapters 10, 11, and 12, respectively.
Components of the Immune System
The structures of the immune system include (1) skin and mucous membranes; (2) the mononuclear phagocyte system; (3) the lymphoid system, including spleen, thymus gland, and lymph nodes; and (4) bone marrow. All these structures are inhabited by different types of WBCs (leukocytes) that mediate inflammation and immunity. Leukocytes are responsible for locating and eliminating pathogens and foreign molecules. They are aided in their task of bodily defense by a number of chemical mediators, including complement, kinins, clotting factors, cytokines, and chemokines.
Components of the immune system are often categorized into specific or innate defenses according to the mechanisms whereby antigens are recognized. Innate defenses require no previous exposure to mount an effective response against an antigen, and a wide variety of different antigens are recognized. Natural killer (NK) cells and phagocytic cells such as neutrophils and macrophages are mediators of innate defenses. In contrast, specific defenses respond more effectively on second exposure to an antigen (adaptive) and are highly selective in the ability to recognize antigens. B lymphocytes (B cells) and T lymphocytes (T cells) are the agents of specific immunity.
Although separating immune components into specific and innate systems is helpful for studying inflammation and immunity, it is an artificial division because they function in a highly integrated manner. The approach used in this chapter is to first describe the major components of the immune system, discuss innate and specific adaptive defenses separately, and then summarize the integrated function of the entire system and its regulation.
Epithelial Barriers
The skin and mucous membranes are sometimes called the “first line of defense” because they are frequently the initial sites of microbial invasion. Intact epithelia in skin and mucous membranes provide mechanical and chemical barriers that prevent microorganisms from gaining access to the body’s tissues. The skin epithelium produces antimicrobial peptides called defensins that can kill a wide variety of bacteria and fungi. The intestinal epithelium produces another form of bactericidal peptide called cryptocidins that prevent bacteria from colonizing the intestinal wall.1 Resident microorganisms may aid in providing this line of defense by making conditions inhospitable for pathogens (see Chapter 8). Disruption of the normal epithelial barriers increases the likelihood that pathogens will successfully establish an infection. Physical trauma (e.g., burns, lacerations, erosions) and biochemical alterations (e.g., pH changes, increased glucose concentration, decreased enzyme production) predispose to infection. Pathogens that breach the skin or mucous membranes are generally first detected by cells of the mononuclear phagocyte system. These cells are thought to originate from monocytes produced in the bone marrow. Specialized antibody-secreting cells also locate to the mucous membranes where they produce antibodies of the immunoglobulin A (IgA) class. IgA antibodies bind antigens on the mucosal surface and prevent them from entering more deeply into the tissues.
Mononuclear Phagocyte System
The mononuclear phagocyte system (previously called the reticuloendothelial system) is composed of dendritic cells, monocytes, and macrophages that are widely distributed throughout the body. Monocytes from the circulating blood migrate to organs and tissues to become macrophages. Macrophages are found throughout the body and are assigned various names according to the tissues in which they are located, such as alveolar macrophages in the lungs, microglial cells in the brain, Kupffer cells in the liver, and histiocytes in connective tissue (Figure 9-1). Dendritic cells are a monocyte-derived cell type that specializes in capturing and presenting antigens to T cells. Dendritic cells are strategically located in subcutaneous and submucosal tissues.
Macrophages and dendritic cells are often the first immune system cells to encounter a pathogen or foreign antigen after it has entered the body, and they are instrumental in communicating news of the invasion to other immune cells. This communication is accomplished through secretion of chemical signaling molecules called cytokines and by presentation of captured antigen to the specific, adaptive immune cells. Dendritic cells specialize in antigen presentation and are able to migrate quickly to lymphoid tissues when they have captured antigens. Macrophages have many other roles in the immune response in addition to their sentry function. Macrophages are powerful phagocytes, each capable of ingesting numerous microbes. Macrophages are called on to clean up the area in which dead neutrophils and inflammatory debris have accumulated after an inflammatory reaction, and they have a role in wound healing.
Lymphoid System
The primary lymphoid organs are the bone marrow and thymus gland, which are the structures where lymphocytes develop. All types of lymphocytes are produced from stem cells in the bone marrow (Figure 9-2). T lymphocytes then migrate to the thymus for development, whereas B lymphocytes and NK cells stay in the marrow to develop. NK cells are a population of lymphocytes that lack both T-cell and B-cell markers. NK cells are produced and released from the bone marrow and function in innate immune responses. NK cells are found mainly in the circulation and spleen. Once mature, T and B lymphocytes migrate to the secondary lymphoid organs where they await activation by antigens. Secondary lymphoid organs include the tonsils, spleen, lymph nodes, and Peyer patches (Figure 9-3).
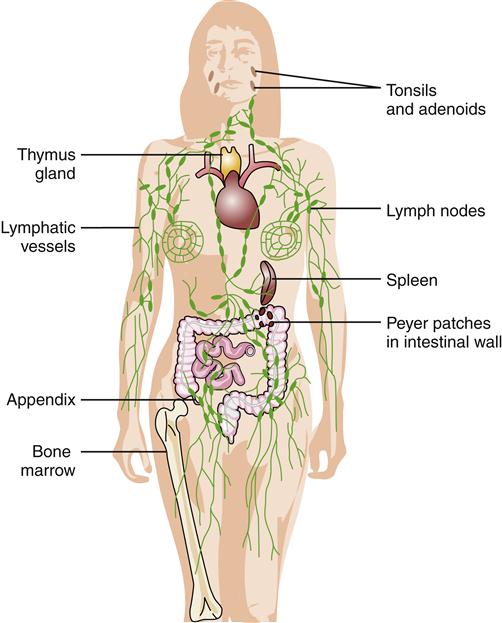
Primary Lymphoid Organs
Bone marrow is contained in all the bones of the body. The primary function of bone marrow is hematopoiesis, or the formation of blood cells. There are two kinds of bone marrow: red and yellow. Hematopoiesis is carried out by red (functioning) marrow. By adulthood, red marrow is confined to the pelvis, sternum, ribs, cranium, ends of the long bones, and vertebral spine. Yellow or fatty bone marrow is found in the remaining bones. It normally does not contribute to hematopoiesis in the adult, but can be recruited to become red marrow again under conditions of increased need for hematopoiesis.2
B lymphocytes (B cells) are produced and develop in the bone marrow. B cells migrate from the outer edges toward the center of the bone marrow as they develop. Pre-B cells are subjected to a highly selective quality control process, and less than 25% of the developing B cells are allowed to survive. During migration through the bone marrow, immature B cells are exposed to self antigens. B cells that do not bind to any antigens continue to develop into mature B cells. If immature B cells encounter antigens to which they bind while still in the bone marrow, a series of events is triggered to induce self-tolerance. The immature B cell is stimulated to reactivate the genetic recombination machinery in an attempt to produce a new B-cell receptor (BCR) that does not bind to self antigens. If this receptor editing attempt fails to alter binding sufficiently, the immature B cell will undergo apoptosis in the bone marrow.3 Mature B cells that leave the bone marrow to colonize secondary lymphoid organs are called naive B cells because they have not yet encountered antigen.
T lymphocytes (T cells) develop in the thymus, which is located in the anterior mediastinum overlying the heart. Pre-T cells initially enter the outer aspect (cortex) of the thymus lobules, and many die while they migrate to the center (medulla) of the thymus. The selection process for T cells is even more rigorous than that for B cells; only about 5% of the cells entering the thymus survive to reenter the circulation and colonize secondary lymphoid organs. The thymus is relatively large at birth and steadily atrophies after puberty.4 The thymus produces interleukin-7 (IL-7), a cytokine that promotes T cell proliferation.
Secondary Lymphoid Organs
Once mature, lymphocytes leave their primary lymphoid organs and travel through the blood to localize in peripheral, or secondary, lymphoid tissues, including lymph nodes, spleen, tonsils, and Peyer patches in the intestine. These naive T cells and B cells express specific receptor proteins on their cell surfaces that allow them to migrate or “home” to specific locations in lymph tissue. Most mature T lymphocytes are in constant circulation through lymphatic tissues and the bloodstream. It has been estimated that a lymphocyte makes a circuit from the blood to tissues to lymphatics and back to the bloodstream once or twice per day.3 Antigens can be carried to the naive cells in the lymph nodes by the specialized antigen-presenting dendritic cells. When exposed to an appropriate antigen, T cells and B cells migrate toward each other within the lymph nodes and begin to proliferate. Activated T cells may then migrate to lymph vessels and travel to the bloodstream, where they are dispersed throughout the system. The majority of B cells stay in the lymph node, where they mature into antibody-secreting plasma cells. Lymphocyte recirculation and homing is regulated by binding interactions between various types of cell adhesion molecules (CAMs) including selectins, integrins, and addressins.3
Tonsils
Tonsils are aggregates of lymphoid tissue located in the mouth and pharynx. The tonsils are strategically located at the entrance to the digestive and respiratory tracts, where they are likely to encounter microorganisms. Unlike lymph nodes, tonsils have no afferent (incoming) lymphatic vessels. They do have efferent lymphatic drainage so that activated lymphocytes from the tonsils can migrate to other lymphoid organs. Tonsils normally make an important contribution to immune function; however, they may occasionally become chronically infected, and surgical removal (tonsillectomy) is then helpful.
Spleen
The spleen is located under the diaphragm on the left side of the body. It measures about 12 cm in length, which makes it the largest of the lymphoid organs. The spleen provides an important filtering function for blood. The tissue structure of the spleen is similar to that of lymph nodes. It is surrounded by a capsule of connective tissue and filled with a meshwork of red pulp and localized masses of lymphocytes called white pulp. Within the red pulp are many blood-filled sinuses lined with macrophages. Macrophages filter out foreign substances and old red blood cells. Lymphocytes located in the white pulp are in a strategic position to come in contact with blood-borne antigens. Lymphocytes thus activated in the spleen can migrate to other lymphoid organs via efferent lymphatics. Like the tonsils, the spleen does not have afferent lymphatic vessels.
Lymph Nodes and Lymphatics
The lymphatic vessels begin with small, closed-ended lymphatic capillaries in direct contact with the interstitial fluid surrounding cells and tissues. Lymphatics pick up fluid and proteins that escape the bloodstream and return them to the circulation by way of the right lymphatic and thoracic ducts. Along the way from lymphatic capillaries to the thoracic ducts, lymph flows through specialized structures called lymph nodes. Lymph nodes are found primarily in the neck, axilla, thorax, abdomen, and groin. They often become tender and palpable when responding to foreign invaders. Projections of connective tissue called trabeculae divide the interior of the lymph node into compartments (Figure 9-4). Lymph nodes contain large numbers of B and T lymphocytes and macrophages. B cells are the predominant cell type in the cortical follicles, whereas T cells predominate in the area just under the cortex called the paracortex. The central region, or medulla, is populated by macrophages, B cells, and plasma cells (antibody-secreting B cells). Lymph fluid flows through the nodes in a way that allows these immune cells to filter, detect, and react to foreign material.
Peyer Patches
Aggregates of lymphoid tissue can be found scattered throughout the body, particularly in the gastrointestinal, respiratory, and urogenital tracts. These structures are analogous to lymph nodes, but they are not encapsulated and contain primarily B cells. Because of their location, these structures have been termed mucosa-associated lymphoid tissue (MALT) or gut-associated lymphoid tissue (GALT). These structures, also called Peyer patches, are of particular importance in producing antibodies to microorganisms that tend to invade mucosal tissue.
Leukocytes
Leukocytes, or WBCs, are the primary effector cells of the immune system. Each of the different types of leukocytes found in blood has a special job to perform. All leukocytes, as well as red blood cells and platelets, are formed from stem cells in the bone marrow. Stem cells can produce daughter cells that differentiate along several different pathways to become mature cell types (see Figure 9-2). The first major differentiation step produces either a lymphoid stem cell or a myeloid stem cell. Lymphoid stem cells further differentiate to form B and T lymphocytes and NK cells. Myeloid stem cells can produce a variety of cell types, including red blood cells, platelets, monocytes, dendritic cells, and granulocytes. Monocytes that migrate from the blood into tissues are called macrophages. Granulocytes are further divided into neutrophils, eosinophils, and basophils. Basophils are precursors of the mast cells located in tissues.
Development of these cell types is influenced by hormonal signaling molecules called cytokines. Cytokines are produced locally in the bone marrow and by various other cells. Certain cytokines stimulate stem cell growth, proliferation, and differentiation into particular cell types. The WBC count and differential are commonly measured laboratory tests used to evaluate white blood cell production. A normal WBC count and differential are shown in Table 9-1. The general features of each of the WBC types are summarized in the following sections.
TABLE 9-1
LEUKOCYTE PROPORTIONS AND FUNCTIONS
TYPE | PERCENTAGE∗ | ROLE IN INFLAMMATION |
Neutrophils | 60-80 | First to appear after injury, phagocytosis |
Lymphocytes | 20-30 | Immune response |
Monocytes (macrophages) | 3-8 | Phagocytosis |
Eosinophils | 1-6 | Allergic reactions, parasite infection |
Basophils | 0-2 | Contain histamine, mediate type I allergic reactions, initiate inflammation |
Neutrophils
Neutrophils are circulating granulocytes that are also known as polymorphonuclear leukocytes (polys or PMNs). They account for 60% to 80% of the total WBC count. Neutrophils normally have two to five nuclear lobes and coarse, clumped chromatin. Neutrophils arise from bone marrow stem cells and undergo several stages of maturation. As illustrated in Figure 9-2, these stages, from least to most mature, are myeloblast, promyelocyte, metamyelocyte, band cell, and mature segmented neutrophil.
Neutrophils stored in the bone marrow outnumber, by about 10-fold, the quantity of circulating neutrophils. An adult produces more than 1 × 1011 neutrophils each day.1 These stored neutrophils are released into the circulation, where they have a half-life of 4 to 10 hours. Neutrophils that are not recruited into tissues within about 6 hours undergo programmed cell death (apoptosis). Neutrophils are early responders to an acute bacterial infection and arrive in large numbers very quickly. They are phagocytes that engulf and degrade microorganisms. Circulating neutrophils have receptors on their cell surfaces that enable them to bind to endothelial cells in areas of inflammation. These receptors, called L-selectins, allow neutrophils to adhere and roll along the capillary surface.5 Other interactions between neutrophil integrin receptors and extracellular matrix then facilitate movement of neutrophils through the capillary wall and into the tissue. Neutrophils are attracted to areas of inflammation and bacterial products by chemotactic factors such as complement fragments and cytokines. This process is discussed in more depth in the section titled Inflammation.
Initially during an acute infection, neutrophilia, or an increase in the number of circulating neutrophils, occurs as the bone marrow releases stored neutrophils. As neutrophils are consumed and demand exceeds production, an increase in the number of immature (band) neutrophils occurs. Bands are identified by their lack of nuclear segmentation. This increase in band cells is referred to as a “shift to the left of normal” (Figure 9-5). Traditionally, the band count has been used to differentiate bacterial from viral infections, and a greater shift to the left is viewed as a more severe infection. The utility of using the band count for these purposes has been called into question because the specificity is poor.6
Neutrophils produce potent chemical mediators that enable them to destroy microorganisms. Numerous toxins released by neutrophils have been identified, including oxidizing free radicals, defensins, and proteolytic enzymes, such as elastase.7 Because of the ability to generate free radicals and release enzymes, neutrophils can cause extensive damage to normal tissue during their inflammatory response.
Eosinophils
Eosinophils are circulating granulocytes that have two nuclear lobes and stain brilliant red-orange with eosin. They constitute 1% to 6% of the total WBC count. Eosinophils mature in the bone marrow (3 to 6 days) and circulate in the blood for about 30 minutes. They have a half-life of 12 days in tissue. Eosinophils arise from myeloid stem cells and undergo a maturation process similar to that of neutrophils.
Eosinophils are particularly associated with an increase in number during allergic reactions and infection by intestinal parasites. The role of eosinophils in allergic reactions is less well characterized than that of mast cells. Eosinophils are recruited into areas of inflammation by the chemokine eotaxin, which is produced by epithelial cells.3 Eosinophils release inflammatory chemicals, such as lysosomal enzymes, peroxidase, major basic protein, and cationic protein. The primary function of eosinophils is to kill parasitic helminths (worms). Helminths are too large to be phagocytosed by neutrophils or macrophages, and their exterior is resistant to attack by complement or mast cell products. Eosinophils produce specialized molecules such as major basic protein and eosinophil cationic protein, which may be more effective against helminths.1 Eosinophils recognize helminths that have been opsonized (coated) with IgE antibody. They bind to the IgE and then release their stored chemicals onto the surface of the opsonized helminth. Parasitic infections are a significant problem in much of the world, with one third of the population being affected.
Basophils and Mast Cells
Basophils are granulocytes characterized by granules that stain blue with basophilic dyes. Basophils account for 0% to 2% of the total leukocyte count. Basophils are structurally similar to mast cells. Mature basophils circulate in the vascular system, whereas mast cells are found in connective tissue, especially around blood vessels and under mucosal surfaces. When stimulated by cytokines, mature basophils can migrate to connective tissue, but once in the tissue, basophils (then called mast cells) do not reenter the circulation.
The average basophil life span is measured in days, whereas mast cells can live for weeks to months. Mast cells and basophils have IgE receptors that allow them to bind and display IgE antibodies on their cell surfaces. When an appropriate stimulus occurs, such as antigen binding to the IgE antibodies, mast cells and basophils release granules (degranulate) containing proinflammatory chemicals.
Mast cell and basophil granules contain histamine, platelet-activating factor, and other vasoactive amines that are important mediators of immediate hypersensitivity responses (Figure 9-6). Degranulation of mast cells and basophils begins the inflammatory response that is characteristically associated with allergic reactions. Mast cells and basophils are also involved in wound healing and chronic inflammatory conditions (see Chapter 10).
Monocytes and Macrophages
Monocytes and macrophages, like granulocytes, originate from bone marrow stem cells of the myeloid lineage. Monocytes are immature macrophages and account for about 5% of the total WBC count. Monocytes circulate in the bloodstream for about 3 days before they enter tissue to become macrophages. As described earlier, macrophages are found in widespread locations as part of the mononuclear phagocyte system.
Phagocytosis by macrophages is similar to that by neutrophils except that neutrophils are short-lived and die in the process of fighting infection. Macrophages, in contrast, may live for months to years and can migrate in and out of tissue. Macrophages are more efficient phagocytes than neutrophils and can ingest several times as many microorganisms (Figure 9-7). Macrophages are capable of cell division and may proliferate at the site of inflammation.
Macrophages are covered with a variety of receptor proteins on their cell surface (Figure 9-8). Some of these receptors help macrophages locate antigens that have been coated by antibodies. These receptors are called Fc receptors because they bind to the part of an antibody called the constant fragment, or Fc. Macrophages also have receptors for the complement component C3b. Complement, like antibodies, can coat an antigen and make it more recognizable to macrophages. Coating of antigen by antibodies or by complement is called opsonization. Macrophages have receptors that help them recognize bacteria directly. These innate pattern-recognition receptors bind to particular molecules prevalent in the bacterial cell wall. For example, mannose receptors and numerous Toll-like receptors on macrophages allow them to recognize common microbial structures (see Figure 9-8). Other receptors, called selectins and integrins, help macrophages adhere to capillary walls, and enter and move through tissue. Integrin receptors bind to proteins in the extracellular matrix and help macrophages target or “home” to certain areas.
In addition to their phagocytic function, macrophages have important secretory function. Some of the substances secreted by macrophages are cytokines, which help to coordinate the activities of other immune cells (Figure 9-9). Macrophage cytokines include IL-1, IL-6, IL-12, and tumor necrosis factor-α (TNF-α). These cytokines promote inflammation, as well as the activity of other WBCs, including neutrophils and lymphocytes (see the section titled Cytokines and Chemokines).
Macrophages secrete a number of proteins that are important in wound healing. Some of these proteins are enzymes that degrade tissue (e.g., collagenase, elastase, plasminogen activator), whereas others stimulate the growth of new granulation tissue (e.g., fibroblast growth factor, angiogenic factors).
A third function of macrophages, in addition to phagocytosis and secretion, is antigen presentation. For T cells to recognize antigens, these antigens must first be processed and presented on the surface of an antigen-presenting cell such as dendritic cells, macrophages, or B cells. Macrophages accomplish this task by first engulfing the antigen, then processing it into smaller pieces, and finally combining the antigen fragments with special membrane proteins. The antigen complexes are then displayed on the macrophage cell surface, where T lymphocytes (T helper cells) can recognize and become activated by them. Antigen presentation is explored in more detail in the section titled Specific Adaptive Immunity.
Dendritic Cells
Dendritic cells are derived in the bone marrow from the same progenitor cells that produce monocytes and macrophages (Figure 9-10) and are structurally and functionally similar to macrophages (Figure 9-11). Dendritic cells derive their name from an unusual shape that has extensive projections from the surface. Dendritic cells are located throughout the body as part of the mononuclear phagocyte system. They function primarily as antigen-presenting cells, capturing antigen in tissues and then migrating to lymphoid areas to present antigen to T cells. Some types of dendritic cells produce chemical messengers called type I interferon (IFN-α and IFN-β) in response to viral infections. Type I interferons suppress the viral replication machinery in nearby cells and help stop the local spread of the virus.
Lymphocytes
The three major types of lymphocytes are NK cells, T cells, and B cells. NK cells function in innate immunity, whereas B and T lymphocytes are the cells responsible for specific, adaptive immunity. B and T cells have the capacity to proliferate into “memory cells,” which provide long-lasting immunity against specific antigens. NK, T, and B cells are derived from a common lymphoid stem cell in the bone marrow that is stimulated to proliferate by bone marrow–derived cytokines including IL-7. T cells then migrate to the thymus, where they mature. B cells remain in the bone marrow during their maturation phase. NK cells are released into the circulation. Together NK, B, and T lymphocytes compose approximately 20% of the total WBC count. Mature NK cells circulate and populate the spleen, whereas T and B cells migrate to secondary lymphoid organs.
Structurally, lymphocytes are small, round cells with a large, round nucleus. Despite their relatively uniform appearance, lymphocytes can be sorted into a number of subpopulations based on characteristic surface proteins called cluster of differentiation (CD) markers. More than 350 different CD markers have been identified thus far, with different immune cell types displaying different combinations on their cell surfaces. Lymphocytes have many complex and differentiated functions, and only the major lymphocyte subtypes are discussed in this chapter.
Natural Killer Cells
NK cells have no B- or T-cell markers and are not dependent on the thymus for development. NK cells are considered to be innate immune cells because they can effectively kill tumor cells and virally infected cells without previous exposure. NK cells kill their target cells by a mechanism similar to that used by cytotoxic T cells. Unlike T and B cells, NK cells can respond to a variety of antigens and are therefore not specific for a particular antigen. Like neutrophils and macrophages, NK cells recognize antibody-coated target cells with their Fc receptors. This process is called antibody-dependent cell-mediated cytotoxicity (ADCC). NK cells also target virally infected cells and tumor cells. They are thought to be able to recognize virally infected cells through innate pattern-recognition receptors; however, only those cells that lack certain normal self proteins on their cell surface (major histocompatibility complex I, or MHC I, proteins) are targeted for killing.3 Cells that display normal MHC I on their cell surfaces are protected from NK cell cytotoxicity, but will be susceptible to killing by cytotoxic T cells that recognize viral antigen displayed on the MHC I proteins.
T Lymphocytes
Two major classes of T lymphocytes can be differentiated by the presence or absence of CD4 and CD8 surface proteins (Figure 9-12). T cells that possess CD4 proteins (CD4+) are called T helper cells. T helper cells interact with antigens presented on the surface of specialized antigen-presenting cells such as dendritic cells, macrophages, and B cells. T helper cells can be further divided into subclasses called TH1, TH2, and TH17 based on the types of cytokines that they secrete (Figure 9-13). The TH1 subset of T helper cells develops in response to IL-12 from macrophages and, when activated, secretes cytokines that activate other T cells (IL-2) and macrophages (interferon-γ [IFN-γ]). TH2 cells develop in response to IL-4 from activated T helper cells and secrete cytokines that stimulate B-cell proliferation and antibody production (e.g., IL-4, IL-5, IL-10, IL-13).8 The TH17 subclass, as its name implies, secretes IL-17, which is a proinflammatory cytokine.
The presence of CD8 protein (CD8+) on a T lymphocyte characterizes it as a cytotoxic T cell. Cytotoxic T cells recognize antigen presented in association with surface proteins that can be found on all nucleated cells of the body (MHC I). When a CD8+ T cell recognizes a foreign antigen on a cell, the antigen-presenting cell is killed, thus the name cytotoxic T cell. CD8+ cells are particularly effective at destroying virally infected cells, foreign cells, and mutant cells (Figure 9-14). Proliferation of activated cytotoxic T cells is enhanced by T helper cell cytokines, particularly IL-2.
B Lymphocytes
B cells are distinguished from other lymphocytes by their ability to produce antibodies and by the presence of antibody-like receptors (B-cell receptors [BCRs]) on their cell surfaces. Each B cell carries many copies (100,000) of identical BCRs and is able to respond to only 1 antigen epitope9 (Figure 9-15). B cells require “help” from T helper cells to respond efficiently to protein antigens. B cells bind and internalize the protein antigen, and then process and present it to T helper cells. T cells that recognize the presented peptides bind to and are activated by the B cell. T-cell help is provided to the B cell by physical cell-to-cell contact through coreceptor binding, as well as through secreted cytokines. Some B cells can respond to nonprotein antigens such as bacterial carbohydrate and lipid molecules. B-cell responses to nonprotein antigens are T-cell independent because T cells respond only to peptide antigens. Exposure to antigens stimulates B cells to mature into antibody-secreting plasma cells and memory cells. B-cell memory cells form a reserve of cells that can quickly mount an immune response on subsequent exposure to the same antigen. Memory cells are able to survive for months to years, whereas most antibody-secreting plasma cells live for only a few days. Plasma cells are able to secrete antibodies at a rate of about 2000 per second per cell.9 Memory B cells and plasma cells develop in germinal centers located in secondary lymphoid organs, including the lymph nodes and spleen. A few long-lived plasma cells inhabit the bone marrow and continue to produce a low level of antibody, which provides immediate protection on second exposure to the same antigen. The mechanisms of specific adaptive immunity are explored later in the chapter.
Chemical Mediators Of Immune Function
Complement
The complement system consists of about 20 plasma proteins that interact to enhance inflammation, chemotaxis, and lysis of target cells. Complement proteins are synthesized in the liver and by macrophages and neutrophils. They circulate in the blood in an inactive form. Activation of the complement cascade occurs via three different pathways: classical, alternative, and lectin. In all three pathways, the inactive complement proteins are converted to their active form in a sequence of reactions. Major actions of complement proteins include cell lysis, facilitation of phagocytosis by opsonization, inflammation, and chemotaxis (Figure 9-16).
The classical pathway is usually triggered by IgG or IgM antibody-antigen complexes. The alternative pathway can be initiated on first exposure to an antigen. Lipopolysaccharide, in bacterial cell walls, and bacterial endotoxin are effective triggers of the alternative pathway. In the classical pathway, an antibody hooked onto an antigen combines with C1, the first of the complement proteins. This step sets in motion a domino effect called the complement cascade (Figure 9-17). The alternative pathway begins with the activation of C3. The alternative pathway can be activated on first exposure and is part of the innate immune response.10 The lectin pathway also can be triggered on first exposure. Lectin is a circulating biomolecule that binds mannose on bacterial cell walls and triggers complement activation at C2 and C4. C3 spontaneously degrades into active C3b fragments in plasma. If microbial cell surfaces are present, the C3b fragment can bind directly to the microbe. Two other complement proteins, factors B and D, combine with C3b to initiate the alternative pathway. C3 is the most important and plentiful of the complement proteins. C3 divides into two fragments called C3a and C3b. C3a is a proinflammatory protein that causes histamine release from mast cells, contraction of smooth muscle, and increased endothelial cell permeability. C3b initiates the next step in the cascade by cleaving C5 into its active fragments C5a and C5b. Complement protein fragment C5a is both a powerful inflammatory chemical and a potent chemotactic agent. C5a chemotaxis stimulates neutrophils and monocytes to migrate to the inflamed tissue. C5a also activates neutrophils by triggering their oxidative activity and increasing their glucose uptake.
The C5b fragment combines with C6, C7, C8, and multiple units of C9 to form a large porelike structure (C5b6789) called the membrane attack complex. The membrane attack complex has a direct cytotoxic effect by attacking cell membranes and disrupting the lipid bilayer. This action allows free movement of sodium and water into the target cell, which causes it to rupture (see Figure 9-17). The complement system is a potent inflammatory and cytotoxic system that is carefully regulated by eight known inhibitory factors.3 Normal host cells produce membrane and inhibitory plasma proteins that prevent complement binding to their surface (e.g., C1 inhibitor, protein S).
Kinins
Bradykinin and kallidin are two of the many kinins present in the body. Kinins are small polypeptides that cause powerful vasodilation. They are especially active in the inflammatory process. The kinin system is linked to the clotting system via the Hageman factor (XII) and is activated with the activation of clotting.1 The first step in this process is the conversion of factor XII to factor XIIa (Figure 9-18). Factor XIIa converts a substance known as prekallikrein to kallikrein. Kallikrein converts precursor substances known as kininogens to kinins. The most prevalent is kallidin, which is then converted to bradykinin. Activated kinins cause increased vascular permeability, vasodilation, and smooth muscle contraction. Kinins are also responsible for pain, which is one of the classic signs of inflammation.
Clotting Factors
The blood coagulation cascade’s major purpose is to stop bleeding. It is also intimately involved in inflammation and triggering of the kinin system. The key linkage between the inflammatory response and clotting system is activated factor XII (Hageman factor) (see Figure 9-18). (The blood coagulation cascade is discussed in detail in Chapter 14.) Activation of the coagulation cascade results in the formation of insoluble fibrin strands, which provide an effective barrier to the spread of infection. Clot formation also activates the fibrinolytic cascade, which splits fibrin proteins. Some of the fibrin degradation products are chemotactic signals for neutrophils.
Cytokines and Chemokines
Cytokines are polypeptide signaling molecules that affect the function of other cells by stimulating surface receptors. Cytokines function in a complex intercellular communication network. WBC cytokines have previously had many names, including monokines, lymphokines, and interleukins, depending on their cell of origin. The number of known cytokines is large and growing; they can be grouped according to their source and function (Table 9-2).
TABLE 9-2
SELECTED IMMUNE CYTOKINES AND THEIR FUNCTIONS
CYTOKINE | ORIGIN | FUNCTION |
IFN-α | Macrophages and induced by RNA or DNA viruses and by single- or double-stranded polyribonucleotides | Inhibits virus replication, toxic to cancer cells, stimulates leukocytes, facilitates NK cell activity, produces fever, increases B- and T-cell activity |
IFN-β | Fibroblasts and induced by RNA or DNA viruses and by single- or double-stranded polyribonucleotides | Inhibits virus replication, toxic to cancer cells, facilitates NK cell activity, produces fever |
IFN-γ | T cells (TH1 and CD8+) and NK cells | Inhibits virus replication, promotes antigen expression, activates macrophages, inhibits cell growth, induces myeloid cell lines, promotes B cell switch to IgG |
IL-1 | Mononuclear phagocyte | Stimulates T cells and macrophages, induces acute phase reaction of inflammation, produces fever; similar to TNF and endogenous pyrogen |
IL-2 | T helper cells (TH1) | Promotes growth of T cells, enhances function of NK cells, assists T-cell maturation in thymus and B-cell proliferation |
IL-3 | T cells, endothelial cells, fibroblasts, other cells | Induces proliferation and differentiation of other lymphocytes, pluripotent stem cells, mast cells, and granulocytes |
IL-4 | T helper cells (TH2) | Promotes T-cell/B-cell interactions, promotes synthesis of IgE by B-cell and TH2 cell growth, promotes mast cell and hematopoietic cell growth |
IL-5 | T helper cells (TH2) | Promotes growth and differentiation of B cells to secrete IgA, induces differentiation of eosinophils |
IL-6 | Mononuclear phagocytes, T helper cells (TH2), tumors, and nonlymphoid cells (e.g., endothelium) | Promotes immunoglobulin secretion by B cells, induces fever, promotes release of inflammation factors from liver cells, promotes differentiation of hematopoietic stem cells and nerve cells |
IL-7 | Stromal cells in bone marrow | Stimulates immature lymphocytes to divide to produce B and T cells |
IL-8 | Macrophages | Enhances inflammation and chemotaxis (CXCL chemokine) |
IL-9 | TH2 cells | Enhances growth of T helper cells |
IL-10 | T cells and macrophages | Inhibits activation of macrophages and dendritic cells, inhibits IL-12 production |
IL-11 | Stromal cells in bone marrow | Stimulates platelet production |
IL-12 | Macrophages, dendritic cells | Enhances TH1 cell activities and release of IFN-γ by T cells and NK cells |
IL-13 | TH2 cells | Stimulates B-cell growth and IgE production, suppresses macrophages |
IL-14 | T cells | Induces B-cell proliferation |
IL-15 | Macrophages (esp. viral infection) | Similar actions to IL-2, enhances proliferation of T cells (CD8) and NK cells |
IL-16 | CD8+ T cells | CD4+ cell chemotaxis, suppresses viral replication of HIV |
IL-17 | CD4+ T cells | Stimulates production of colony-stimulating factors and chemokines |
IL-18 | Macrophages in response to microbes | Increases NK cell proliferation and secretion of IFN-γ by TH1 |
IL-19 | Macrophages | Stimulates macrophage IL-1 secretion |
IL-20 | Monocytes | Stimulates hematopoietic stem cells |
IL-21 | TH2 and TH17 cells | Activates B cells, stimulates production of NK cells |
IL-22 | TH17 cells | Epithelial cells, increased barrier function, defensin production |
IL-23 | Macrophages and dendritic cells | Similar to IL-12, stimulates cell-mediated immunity |
IL-24 | Monocytes, T cells | Monocyte inflammatory cytokine production |
IL-25 | TH2 cells | Stimulates production of cytokines by TH2 cells |
IL-26 | T cells, monocytes | Uncertain |
IL-27 | Macrophages and dendritic cells | Inhibits TH1 cells |
TNF-α | Macrophages | Induces leukocytosis, fever, weight loss, inflammation, necrosis of some tumors; stimulates lymphokine synthesis; activates macrophages; toxic to viruses and tumor cells |
TNF-β | T cells | Inhibits B-cell and T-cell proliferation |
G-CSF, M-CSF, GM-CSF | Macrophages, T cells, fibroblasts | Stimulates granulocyte and monocyte production in bone marrow |
TGF-β | T cells, macrophages | Inhibits T cells, B cells, and macrophages |
Chemokines | ||
CXCL 1-16 | Macrophages and various cells in tissues | Recruitment of neutrophils, macrophages, lymphocytes |
CCL 1-28 | Macrophages and various cells in tissues | Recruitment of neutrophils, macrophages, lymphocytes |
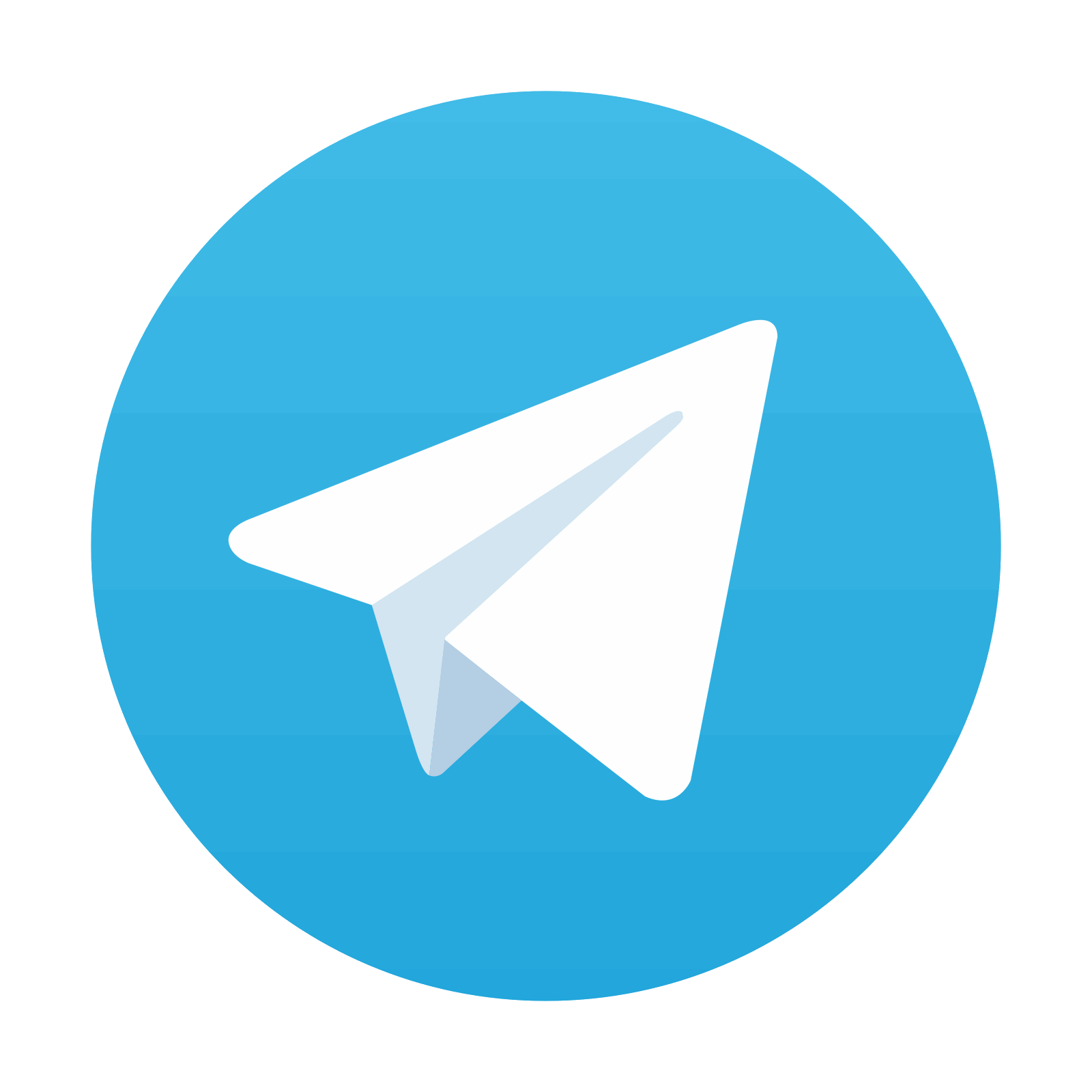
Stay updated, free articles. Join our Telegram channel

Full access? Get Clinical Tree
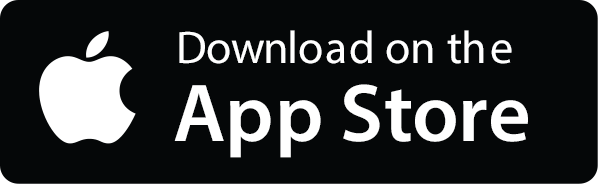
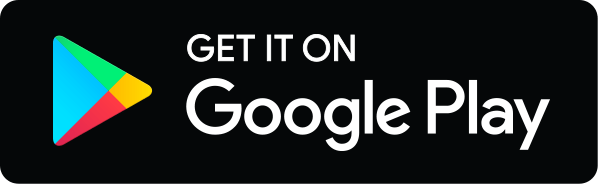