Fig. 4.1 Development of paralysis. A mouse hemidiaphragm was continuously stimulated via the phrenic nerve at a frequency of 1 Hz. After equilibration, the muscle was exposed to 1 ng/ml botulinum neurotoxin type A (BoNT-A). The arrows indicate when the neurotoxin was applied and when the amplitude was reduced by 50% of its initial value, respectively. Paralysis time is defined as the time elapsed until the contraction amplitude has been halved.
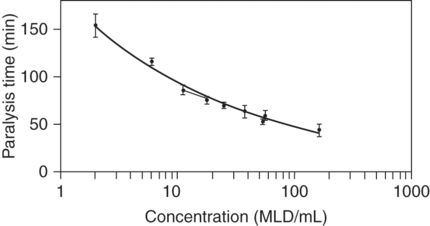
Fig. 4.2 Concentration–response curves for a standard batch of botulinum neurotoxin type A. One curve was constructed using samples containing pure neurotoxin in a median lethal dose (MLD) concentration range of 2 to 162 MLD/ml, the other from the same batch but in the range 11–56 MLD/ml. The curve with the lower range was fitted by linear regression.
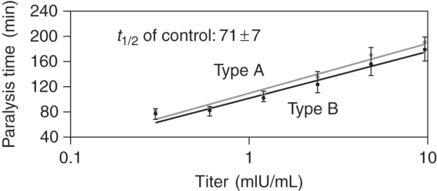
Fig. 4.3 Calibration curves for botulinum neurotoxin types A and B. Antibody titers are plotted against paralysis times in the ex vivo model (n = 3 ±SD). The standard antibody was taken from Botulism Antitoxin from Behring, Marburg, Germany (750 U/ml). Paralysis time in the antibody-free control was 71 minutes. With increasing titers, the paralysis time was prolonged. Use of paralysis time allows antibody titers in patients’ sera to be calculated when these sera are supplemented with the same neurotoxin concentration as used for the calibration curves.
Reactions of the organism to botulinum neurotoxin
As BoNT is a foreign protein, it should be recognized by B-cells, which would bind BoNT with the help of specific, preformed antigen receptors. Subsequently, the BoNT is internalized and proteolysed to small peptides of 9–20 amino acid residues. These peptides are presented to the outside of the B-cells associated with proteins of the major histocompatibility complex (MHC). These antigen-presenting B-cells are bound by T-helper cells in the presence of costimulatory molecules. As a result, the T-cells release cytokines; these, together with the MHC-bound peptides, stimulate the B-cells to differentiate into plasma cells. Plasma cells then produce and release specific BoNT-binding immunoglobulins, anti-BoNT. These antibodies protect the host either by neutralizing BoNT, which then loses its toxic properties, or simply by binding the BoNT. These complexes of BoNT and anti-BoNT antibodies may retain toxicity but because of the linked antibody the complexes can be easily recognized and phagocytosed by accessory cells (clearing antibodies; Shankar et al., 2007). Recently, positive T-cell responses to BoNT-A have been shown in 95 patients who were treated with the neurotoxin, but there was no difference in the T-cell response between the BoNT-A-responsive patients and the non-responsive patients (Oshima et al., 2011).
Some exogenic factors can facilitate the immune response. It is well known that certain lectins, such as wheat germ agglutinin, phytohemagglutinin, concanavalin A, the B-unit of cholera toxin, or ricin, and others (e.g. components of wasp venom) may stimulate immune cells. These lectins may act as immune adjuvants enhancing antibody concentration. Another factor stimulating immune responses is the amount of antigen exposed to the immune system. In exposure to BoNT-A, the probability of stimulating the immune system increases with the dose of BoNT applied (Göschel et al., 1997; Lange et al., 2009).
Product specificity of immune responses
The therapeutic use of proteins is always associated with immune reactions. Even drugs based on proteins of human origin such as insulin, human growth hormone and erythropoietin may induce antibody formation (Kromminga and Schellekens, 2005). The factors that trigger immunogenicity are impurities, aggregation, formulation and degradation (e.g. oxidation). In addition to these product-specific factors, host-specific factors (e.g. host immune competence) can also determine the immunological response (Kromminga and Schellekens, 2005).
As BoNT is a foreign protein, it will be immunogenic. Prevention of antibody formation can only be avoided by administration of BoNT in extremely small quantities and with long intervals. Nevertheless, in a small number of patients, BoNT does elicit antibody formation, which can inactivate it. The formation of anti-BoNT antibodies in sufficient quantities effectively terminates BoNT therapy (Herrmann et al., 2004).
In the following, factors influencing the immunogenic potential of different BoNT drugs are discussed. Although onabotulinumtoxinA, abobotulinumtoxinA and incobotulinumtoxinA are based on the same active substance, the 150 kDa BoNT-A protein, they contain a different set of additional neurotoxin-associated proteins (except incobotulinumtoxinA). Moreover, they are formulated differently. These differences can influence the immune response to the BoNT drugs.
It has long been known that the neurotoxin-associated proteins (particularly the hemagglutinins) elicit antibodies in 40–60% of patients treated with the complex-containing products (Göschel et al., 1997; Critchfield, 2002), whereas the proportion of patients with anti-BoNT antibodies remains small. Antibodies against the neurotoxin-associated proteins do not interfere with the neurotoxins, whereas anti-BoNT antibodies will neutralize BoNT and thus cause therapy failure (Göschel et al., 1997).
Whereas the non-toxic non-hemagglutinating protein is responsible for binding the neurotoxin into the complex (Shenyan et al., 2012), some of the other neurotoxin-associated proteins are hemagglutinins thought to facilitate the absorption of BoNT from the gut (Fujinaga et al., 2009). They act, however, as lectins with high specificity to galactose-containing glycoproteins or glycolipids. Other lectins are known to act as immune adjuvants. For example, the cell-binding subunit of ricin that resembles one of the C. botulinum hemagglutinin (HA-1) stimulates antibody production against a virus antigen (Choi et al., 2006).
Concomitant administration of an adjuvant strongly facilitates the immune response against a single antigen (Critchfield, 2002). In an immunization experiment, Lee et al. (2006) showed that hemagglutinins act as adjuvants, enhancing the antibody titer against BoNT-B. They also demonstrated a hemagglutinin-induced increase of the production of interleukin-6 (a B-cell-activating cytokine). However, Lee et al. (2005) used a formalin-inactivated toxin (toxoid) in a dose 100 000 times greater than therapeutic doses. In addition, they injected at weekly intervals, which does not reflect therapeutic recommendations, as already discussed by Atassi (2006). Therefore, it is difficult to estimate the immunological role of the neurotoxin-associated proteins when therapeutic doses are applied, even though hemagglutinins possess an immune adjuvant activity.
The amount of BoNT that is actually exposed to the immune system is also influenced by the specific potency of the BoNT used in the therapeutic preparation (Göschel et al., 1997; Dressler and Hallett, 2006). In onabotulinumtoxinA, approximately 40% of the original BoNT potency is lost during the manufacturing process, thus producing toxoid that cannot be used for therapeutic purposes but which still acts as an antigen (Hunt, 2007).
The specific potency (activity related to the amount of clostridial protein) of abobotulinumtoxinA (1 U = 25 pg) is higher than that of onabotulinumtoxinA (1 U = 50 pg), which can be partly explained by the difference in size of the complex. Whereas onabotulinumtoxinA consists of the 900 kDa complex, abobotulinumtoxinA contains the 300 kDa complex in addition to the 600 kDa complex (Hambleton, 1992). There is no information about the specific activity of the active substance before formulation; therefore, it is not known if there is any denatured neurotoxin in the final product. Despite the fact that abobotulinumtoxinA has to be administered in three times higher doses than onabotulinumtoxinA numerically, the actual dose applied is probably lower because, owing to a low concentration of albumin in this product, some of the toxin binds irreversibly to glass and plastic surfaces. This bound toxin will not reach the patient’s tissue; consequently, the dose applied is probably as low as a respective dose of onabotulinumtoxinA.
The active substance of abobotulinumtoxinA shows some impurities not related to the complexing proteins (Pickett et al., 2005). It is notable that a flagellin is present, a protein which is known for its immunostimulatory properties (Honko et al., 2006). It reacts with the Toll-like receptor-5 and induces the maturation of dendritic cells that activate T-cells. It was shown that the addition of flagellin to tetanus toxoid in a vaccination experiment enhanced the antibody titer against tetanus toxin (Lee et al., 2006). However, as discussed above for onabotulinumtoxinA, the doses of adjuvant proteins applied experimentically were much higher than the doses given therapeutically; also in this case, difficulties arise about the assessment of the role flagellin plays in patients treated with abobotulinumtoxinA.
In contrast to onabotulinumtoxinA and abobotulinumtoxinA, incobotulinumtoxinA only contains the purified neurotoxin and no other clostridial proteins; it, therefore, shows the highest specific activity (1 U = 4.4 pg) (Frevert, 2010).
The immunogenic potential of BoNT-B versus BoNT-A has not been well investigated. In people vaccinated with the pentavalent botulinum toxoid vaccine, the antibody titer against BoNT-A is markedly higher than the titer against BoNT-B (Siegel, 1989), but the vaccine contains toxins inactivated by treatment with formalin, which could influence their antigenic potential. It has to be considered that BoNT-B is not fully activated. The unnicked, non-activated proportion of BoNT-B (about 25%) is inactive and could act as a toxoid (Aoki, 2002). The specific activity of rimabotulinumtoxinB is remarkably higher (1 U = 11 pg) than the specific activity of BoNT-A-containing products, but this is only true when mice are involved (Callaway, 2004). A single mutation in the gene for the human BoNT-B receptor, synaptotagmin-II, results in a drastic decrease in the the receptor’s affinity for the toxin, of approximately 100-fold (Strotmeier et al., 2012). Therefore, this type has to be applied in much higher doses to achieve a therapeutic effect. If one considers that this substantially higher dose of rimabotulinumtoxinB has to be injected, the specific potency in humans is much lower (estimated 40-fold; Dressler, 2006). This substantially increases the risk of developing antibodies. Therefore, more than 40% of de novo patients treated with rimabotulinumtoxinB for cervical dystonia developed complete antibody-induced therapy failure after only a few treatments (Dressler and Bigalke, 2004). Table 4.1 summarizes the average protein load contained in doses of BoNT products used for the treatment of cervical dystonia.
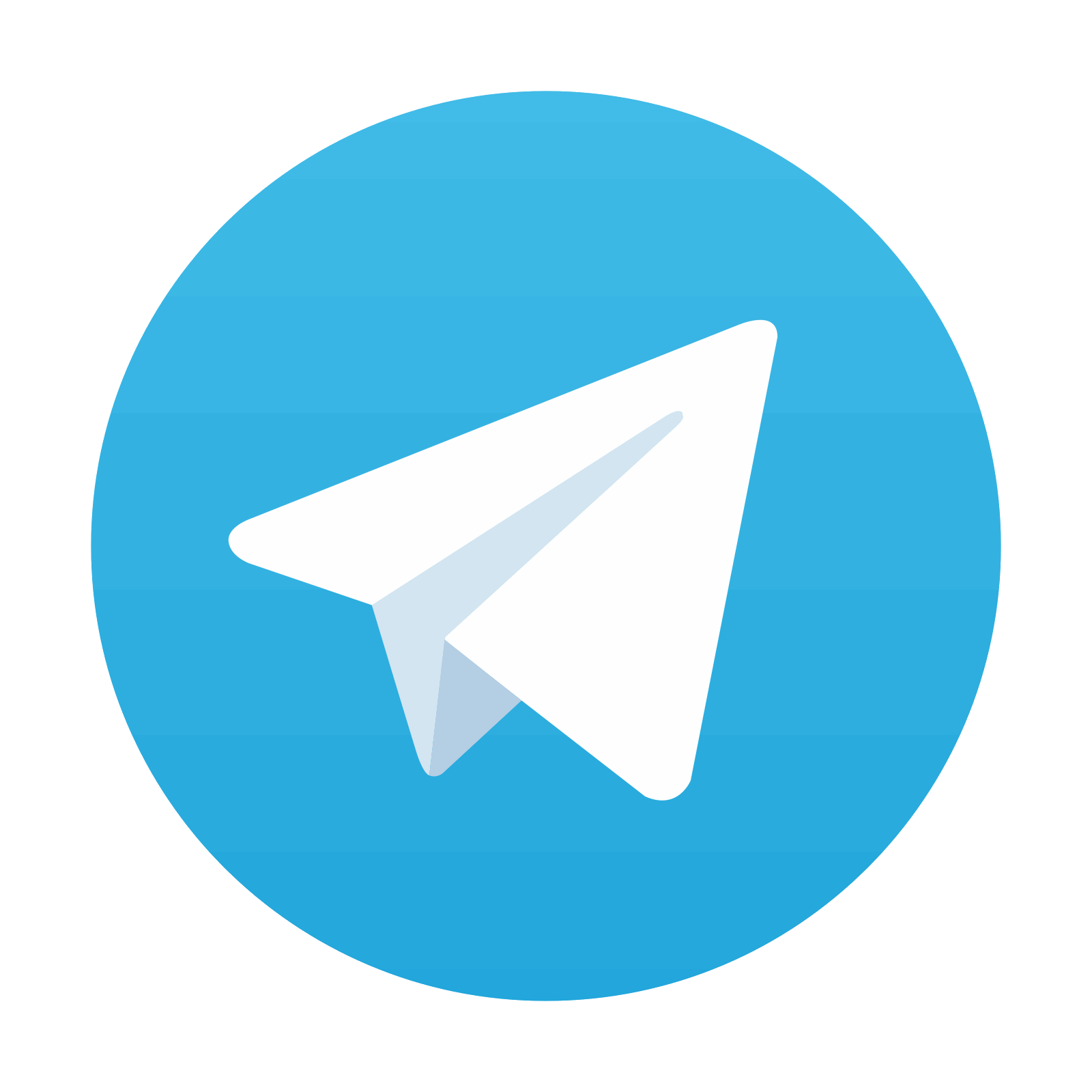
Stay updated, free articles. Join our Telegram channel

Full access? Get Clinical Tree
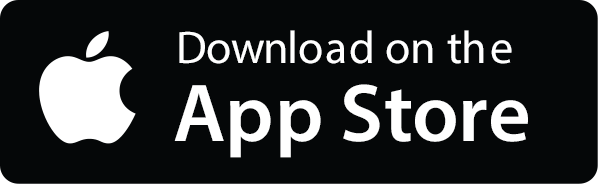
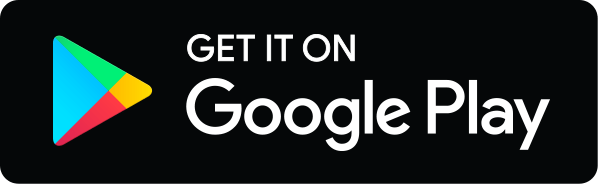