OBJECTIVES
After studying this chapter, you should be able to:
Understand the significance of immunity, particularly with respect to defending the body against microbial invaders.
Define the circulating and tissue cell types that contribute to immune and inflammatory responses.
Describe how phagocytes are able to kill internalized bacteria.
Identify the functions of hematopoietic growth factors, cytokines, and chemokines.
Delineate the roles and mechanisms of innate, acquired, humoral, and cellular immunity.
Understand the basis of inflammatory responses and wound healing.
INTRODUCTION
As an open system, the body is continuously called upon to defend itself from potentially harmful invaders such as bacteria, viruses, and other microbes. This is accomplished by the immune system, which is subdivided into innate and adaptive (or acquired) branches. The immune system is composed of specialized effector cells that sense and respond to foreign antigens and other molecular patterns not found in human tissues. Likewise, the immune system clears the body’s own cells that have become senescent or abnormal, such as cancer cells. Finally, normal host tissues occasionally become the subject of inappropriate immune attack, such as in autoimmune diseases or in settings where normal cells are harmed as innocent bystanders when the immune system mounts an inflammatory response to an invader. It is beyond the scope of this volume to provide a full treatment of all aspects of modern immunology. Nevertheless, the student of physiology should have a working knowledge of immune functions and their regulation, due to a growing appreciation for the ways in which the immune system can contribute to normal physiologic regulation in a variety of tissues, as well as contributions of immune effectors to pathophysiology.
IMMUNE EFFECTOR CELLS
Many immune effector cells circulate in the blood as the white blood cells. In addition, the blood is the conduit for the precursor cells that eventually develop into the immune cells of the tissues. The circulating immunologic cells include granulocytes (polymorphonuclear leukocytes, PMNs), comprising neutrophils, eosinophils, and basophils; lymphocytes; and monocytes. Immune responses in the tissues are further amplified by these cells following their extravascular migration, as well as tissue macrophages (derived from monocytes) and mast cells (related to basophils). Acting together, these cells provide the body with powerful defenses against tumors and viral, bacterial, and parasitic infections.
All granulocytes have cytoplasmic granules that contain biologically active substances involved in inflammatory and allergic reactions.
The average half-life of a neutrophil in the circulation is 6 h. To maintain the normal circulating blood level, it is therefore necessary to produce over 100 billion neutrophils per day. Many neutrophils enter the tissues, particularly if triggered to do so by an infection or by inflammatory cytokines. They are attracted to the endothelial surface by cell adhesion molecules known as selectins, and they roll along it. They then bind firmly to neutrophil adhesion molecules of the integrin family. They next insinuate themselves through the walls of the capillaries between endothelial cells by a process called diapedesis. Many of those that leave the circulation enter the gastrointestinal tract and are eventually lost from the body.
Invasion of the body by bacteria triggers the inflammatory response. The bone marrow is stimulated to produce and release large numbers of neutrophils. Bacterial products interact with plasma factors and cells to produce agents that attract neutrophils to the infected area (chemotaxis). The chemotactic agents, which are part of a large and expanding family of chemokines (see following text), include a component of the complement system (C5a); leukotrienes; and polypeptides from lymphocytes, mast cells, and basophils. Other plasma factors act on the bacteria to make them “tasty” to the phagocytes (opsonization). The principal opsonins that coat the bacteria are immunoglobulins of a particular class (IgG) and complement proteins (see following text). The coated bacteria then bind to G-protein—coupled receptors on the neutrophil cell membrane. This triggers increased motor activity of the cell, exocytosis, and the so-called respiratory burst. The increased motor activity leads to prompt ingestion of the bacteria by endocytosis (phagocytosis). By exocytosis, neutrophil granules discharge their contents into the phagocytic vacuoles containing the bacteria and also into the interstitial space (degranulation). The granules contain various proteases plus antimicrobial proteins called defensins. In addition, the cell membrane-bound enzyme nicotinamide adenine dinucleotide phosphate (NADPH) oxidase is activated, with the production of toxic oxygen metabolites. The combination of the toxic oxygen metabolites and the proteolytic enzymes from the granules makes the neutrophil a very effective killing machine.
Activation of NADPH oxidase is associated with a sharp increase in O2 uptake and metabolism in the neutrophil (the respiratory burst) and generation of O2– by the following reaction:
O2– is a free radical formed by the addition of one electron to O2. Two O2– react with two H+ to form H2O2 in a reaction catalyzed by the cytoplasmic form of superoxide dismutase (SOD-1):
O2– and H2O2 are both oxidants that are effective bactericidal agents, but H2O2 is converted to H2O and O2 by the enzyme catalase. The cytoplasmic form of SOD contains both Zn and Cu. It is found in many parts of the body. It is defective as a result of genetic mutation in a familial form of amyotrophic lateral sclerosis (ALS; see Chapter 15). Therefore, it may be that O2– accumulates in motor neurons and kills them in at least one form of this progressive, fatal disease. Two other forms of SOD encoded by at least one different gene are also found in humans.
Neutrophils also discharge the enzyme myeloperoxidase, which catalyzes the conversion of Cl–, Br–, I–, and SCN– to the corresponding acids (HOCl, HOBr, etc). These acids are also potent oxidants. Because Cl– is present in greatest abundance in body fluids, the principal product is HOCl.
In addition to myeloperoxidase and defensins, neutrophil granules contain elastase, metalloproteinases that attack collagen, and a variety of other proteases that help destroy invading organisms. These enzymes act in a cooperative fashion with O2–, H2O2, and HOCl to produce a killing zone around the activated neutrophil. This zone is effective in killing invading organisms, but in certain diseases (eg, rheumatoid arthritis) the neutrophils may also cause local destruction of host tissue.
Like neutrophils, eosinophils have a short half-life in the circulation, are attracted to the surface of endothelial cells by selectins, bind to integrins that attach them to the vessel wall, and enter the tissues by diapedesis. Like neutrophils, they release proteins, cytokines, and chemokines that produce inflammation but are capable of killing invading organisms. However, eosinophils have some selectivity in the way in which they respond and in the killing molecules they secrete. Their maturation and activation in tissues is particularly stimulated by IL-3, IL-5, and granulocyte-macrophage colony-stimulating factor (GM-CSF) (see below). They are especially abundant in the mucosa of the gastrointestinal tract, where they defend against parasites, and in the mucosa of the respiratory and urinary tracts. Circulating eosinophils are increased in allergic diseases such as asthma and in various other respiratory and gastrointestinal diseases.
Basophils also enter tissues and release proteins and cytokines. They resemble but are not identical to mast cells, and like mast cells they contain histamine (see below). They release histamine and other inflammatory mediators when activated by binding of specific antigens to cell-fixed IgE molecules, and participate in immediate-type hypersensitivity (allergic) reactions. These range from mild urticaria and rhinitis to severe anaphylactic shock. The antigens that trigger IgE formation and basophil (and mast cell) activation are innocuous to most individuals and are referred to as allergens.
Mast cells are heavily granulated cells of the connective tissue that are abundant in tissues that come into contact with the external environment, such as beneath epithelial surfaces. Their granules contain proteoglycans, histamine, and many proteases. Like basophils, they degranulate when allergens bind to cell-bound IgE molecules directed against them. They are involved in inflammatory responses initiated by immunoglobulins IgE and IgG (see below). The inflammation combats invading parasites. In addition to this involvement in acquired immunity, they release TNF-α in response to bacterial products by an antibody-independent mechanism, thus participating in the nonspecific innate immunity that combats infections prior to the development of an adaptive immune response (see following text). Marked mast cell degranulation produces clinical manifestations of allergy up to and including anaphylaxis.
Monocytes enter the blood from the bone marrow and circulate for about 72 h. They then enter the tissues and become tissue macrophages (Figure 3–1). Their life span in the tissues is unknown, but bone marrow transplantation data in humans suggest that they persist for about 3 months. It appears that they do not reenter the circulation. Some may end up as the multinucleated giant cells seen in chronic inflammatory diseases such as tuberculosis. The tissue macrophages include the Kupffer cells of the liver, pulmonary alveolar macrophages (see Chapter 34), and microglia in the brain, all of which come originally from the circulation. In the past, they have been called the reticuloendothelial system, but the general term tissue macrophage system seems more appropriate.
Macrophages are activated by cytokines released from T lymphocytes, among others. Activated macrophages migrate in response to chemotactic stimuli and engulf and kill bacteria by processes generally similar to those occurring in neutrophils. They play a key role in innate immunity (see below). They also secrete up to 100 different substances, including factors that affect lymphocytes and other cells, prostaglandins of the E series, and clot-promoting factors.
Lymphocytes are key elements in the production of acquired immunity (see below). After birth, some lymphocytes are formed in the bone marrow. However, most are formed in the lymph nodes (Figure 3–2), thymus, and spleen from precursor cells that originally came from the bone marrow and were processed in the thymus (T cells) or bone marrow (B cells, see below). Lymphocytes enter the bloodstream for the most part via the lymphatics. At any given time, only about 2% of the body lymphocytes are in the peripheral blood. Most of the rest are in the lymphoid organs. It has been calculated that in humans, 3.5 × 1010 lymphocytes per day enter the circulation via the thoracic duct alone; however, this count includes cells that reenter the lymphatics and thus traverse the thoracic duct more than once. The effects of adrenocortical hormones on the lymphoid organs, the circulating lymphocytes, and the granulocytes are discussed in Chapter 20.
During fetal development, and to a much lesser extent during adult life, lymphocyte precursors come from the bone marrow. Those that populate the thymus (Figure 3–3) become transformed by the environment in this organ into T lymphocytes. Another lymphoid subset that forms in the thymus is the NKT cell, so-called because it shares features of both T lymphocytes and natural killer (NK) cells. The transformation to B lymphocytes occurs in the fetal liver and, after birth, the bone marrow. NK cells also form in these sites. After residence in the thymus, liver, or bone marrow, many of the T and B lymphocytes migrate to the lymph nodes.
FIGURE 3–3
Development of the system mediating acquired immunity. Committed lymphoid progenitors arise in the bone marrow. Maturation into the B and NK cell lineages occurs in this site, whereas development into T and NKT cells takes place after lymphoid progenitors migrate to the thymus. NK, natural killer.
T and B lymphocytes are morphologically indistinguishable but can be identified by markers on their cell membranes. B cells differentiate sequentially into cells capable of production of the various classes of immunoglobulins and thereafter into plasma cells. There are two major types of T cells: cytotoxic T cells and helper/effector T cells. There are at least four subtypes of helper T cells: T helper 1 (TH1) cells secrete IL-2 and γ-interferon and are concerned primarily with stimulating cellular immunity; TH2 cells secrete IL-4 and IL-5 and interact primarily with B cells in relation to humoral immunity. TH17 cells are induced in response to bacterial infections, produce IL-6 and IL-17, and help recruit neutrophils. They are also implicated in the generation of harmful inflammatory responses that occur in autoimmune diseases. Finally, Treg cells produce IL-10 to dampen T cell–driven responses. Cytotoxic T cells destroy transplanted cells and those expressing foreign antigens (eg, virally infected targets), with their development aided and directed by helper T cells.
Markers on the surface of lymphocytes are assigned CD (clusters of differentiation) numbers on the basis of their reactions to a panel of monoclonal antibodies. Most cytotoxic T cells display the glycoprotein CD8, and helper T cells display the glycoprotein CD4. These proteins are closely associated with the T cell receptors and may function as coreceptors. On the basis of differences in their receptors and functions, cytotoxic T cells are divided into αβ and γδ types (see below). NK and NKT cells (see above) are also cytotoxic lymphocytes. Thus, there are four main types of cytotoxic lymphocytes in the body: αβ T cells, γδ T cells, NK cells, and NKT cells.
After exposure to a given antigen, a small number of activated B and T cells persist as memory B and T cells. These cells are readily converted to effector cells by a later encounter with the same antigen. This ability to produce an accelerated response to a second exposure to an antigen is a key characteristic of acquired immunity. The ability persists for long periods of time, and in some instances (eg, immunity to measles) it can be life-long.
After activation in lymph nodes, lymphocytes disperse widely throughout the body and are especially plentiful in areas where invading organisms enter the body (eg, the mucosa of the respiratory and gastrointestinal tracts). This puts memory cells close to sites of reinfection and may account in part for the rapidity and strength of their response. Chemokines are involved in guiding activated lymphocytes to these locations.
The production of white blood cells is regulated with great precision in healthy individuals, and the production of granulocytes is rapidly and dramatically increased in infections. The proliferation and self-renewal of hematopoietic stem cells (HSCs) depends on stem cell factor (SCF). Other factors specify particular lineages. The proliferation and maturation of the cells that enter the blood from the marrow are regulated by growth factors that cause cells in one or more of the committed cell lines to proliferate and mature (Table 3–1). The regulation of erythrocyte production by erythropoietin is discussed in Chapter 38. Three additional factors are called colony-stimulating factors (CSFs) because they cause appropriate single stem cells to proliferate in soft agar, forming colonies. The factors stimulating the production of committed stem cells include GM-CSF, granulocyte CSF (G-CSF), and macrophage CSF (M-CSF). Interleukins IL-1 and IL-6 followed by IL-3 (Table 3–1) act in sequence to convert pluripotential uncommitted stem cells to committed progenitor cells. IL-3 is also known as multi-CSF. Each of the CSFs has a predominant action, but all the CSFs and interleukins also have other overlapping actions. In addition, they activate and sustain mature blood cells. It is interesting in this regard that the genes for many of these factors are located together on the long arm of chromosome 5 and may have originated by duplication of an ancestral gene. It is also interesting that basal hematopoiesis is normal in mice in which the GM-CSF gene is knocked out indicating that loss of one factor can be compensated for by others. On the other hand, the absence of GM-CSF causes accumulation of surfactant in the lungs (see Chapter 34).
Cytokine | Cell Lines Stimulated | Cytokine Source |
---|---|---|
IL-1 | Erythrocyte Granulocyte Megakaryocyte Monocyte | Multiple cell types |
IL-3 | Erythrocyte Granulocyte Megakaryocyte Monocyte | T lymphocytes |
IL-4 | Basophil | T lymphocytes |
IL-5 | Eosinophil | T lymphocytes |
IL-6 | Erythrocyte Granulocyte Megakaryocyte Monocyte | Endothelial cells, fibroblasts, macrophages |
IL-11 | Erythrocyte Granulocyte Megakaryocyte | Fibroblasts, osteoblasts |
Erythropoietin | Erythrocyte | Kidney Kupffer cells of liver |
SCF | Erythrocyte Granulocyte Megakaryocyte Monocyte | Multiple cell types |
G-CSF | Granulocyte | Endothelial cells, fibroblasts, monocytes |
GM-CSF | Erythrocyte Granulocyte Megakaryocyte | Endothelial cells, fibroblasts, monocytes, T lymphocytes |
M-CSF | Monocyte | Endothelial cells, fibroblasts, monocytes, |
Thrombopoietin | Megakaryocyte | Liver, kidney |
As noted in Chapter 38, erythropoietin is produced mainly by kidney cells and is a circulating hormone. The other factors are produced by macrophages, activated T cells, fibroblasts, and endothelial cells. For the most part, the factors act locally in the bone marrow (Clinical Box 3–1).
IMMUNITY
Insects and other invertebrates have only innate immunity. This system is triggered by receptors that bind sequences of sugars, lipids, amino acids, or nucleic acids that are common on bacteria and other microorganisms, but are not found in eukaryotic cells. These receptors, in turn, activate various defense mechanisms. The receptors are coded in the germ line, and their fundamental structure is not modified by exposure to antigen. The activated defenses include, in various species, release of interferons, phagocytosis, production of antibacterial peptides, activation of the complement system, and several proteolytic cascades. Even plants release antibacterial peptides in response to infection. This primitive immune system is also important in vertebrates, particularly in the early response to infection. However, in vertebrates, innate immunity is also complemented by adaptive or acquired immunity, a system in which T and B lymphocytes are activated by specific antigens. T cells bear receptors related to antibody molecules, but which remain cell-bound. When these receptors encounter their cognate antigen, the T cell is stimulated to proliferate and produce cytokines that orchestrate the immune response, including that of B cells. Activated B lymphocytes form clones that produce secreted antibodies, which attack foreign proteins. After the invasion is repelled, small numbers of lymphocytes persist as memory cells so that a second exposure to the same antigen provokes a prompt and magnified immune attack. The genetic event that led to acquired immunity occurred 450 million years ago in the ancestors of jawed vertebrates and was probably insertion of a transposon into the genome in a way that made possible the generation of the immense repertoire of T cell receptors and antibodies that can be produced by the body.
In vertebrates, including humans, innate immunity provides the first line of defense against infections, but it also triggers the slower but more specific acquired immune response (Figure 3–4)
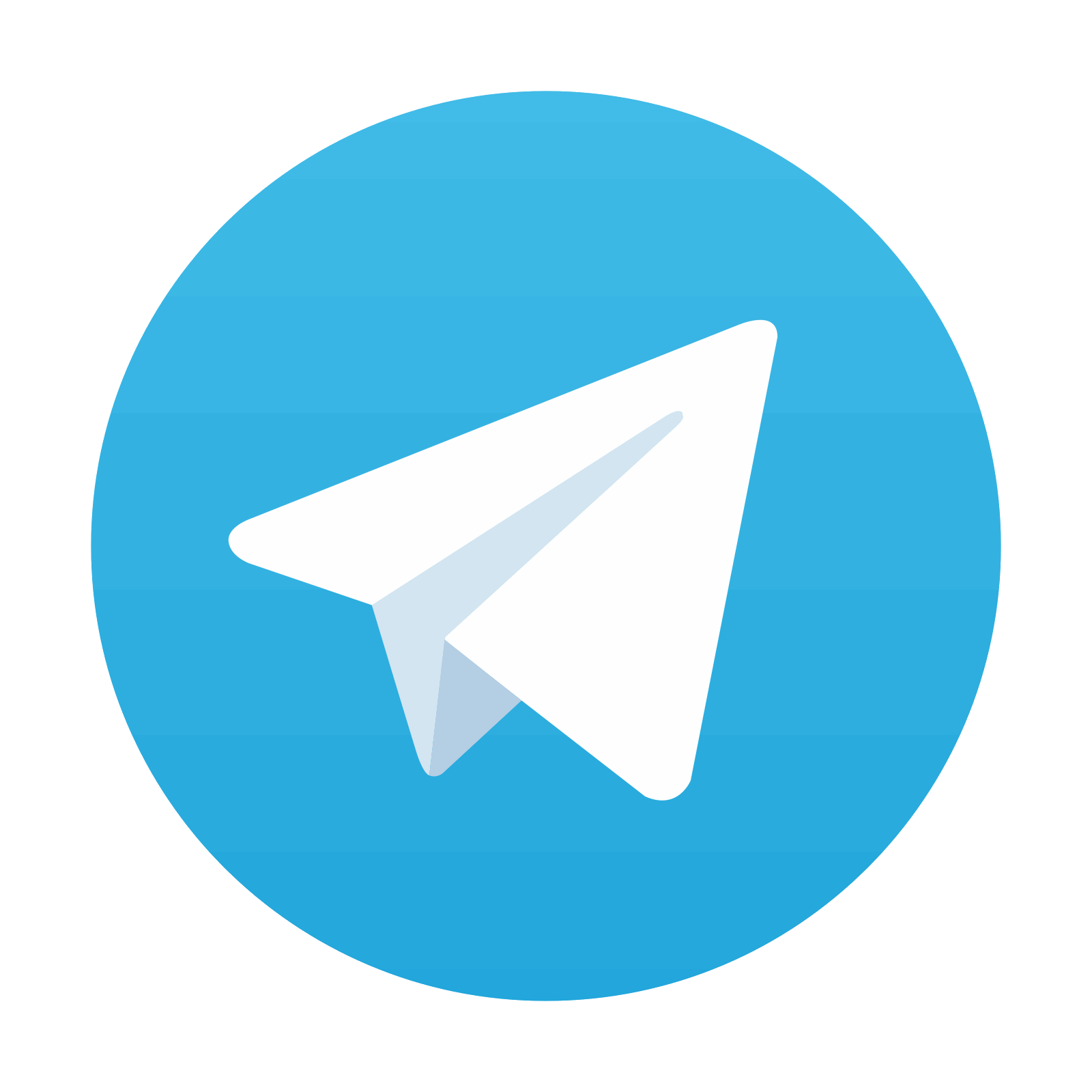
Stay updated, free articles. Join our Telegram channel

Full access? Get Clinical Tree
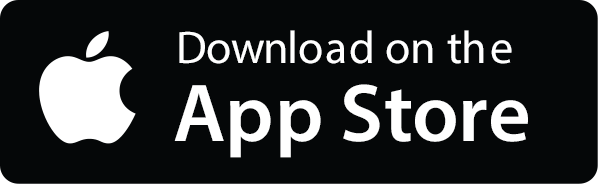
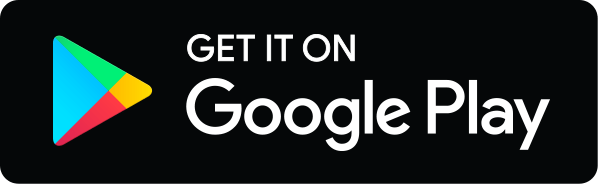
