Nature of therapy
Examples
Pharmacological agents
Physical/chemical agents
Biological materials
Biocompatible nonbiological materials
Scaffolds [10]
Surgical interventions
Novel therapies
Cellular transplantations
Detailed descriptions of SCI pathology would be beyond the scope of this chapter and it may be pertinent just to state that it is a complex interplay of several factors such as ischemia, excitotoxicity, oxidative stress, delayed cell death due to necrosis and apoptosis, axon inhibitory molecular expression, poor regeneration of axons due to lack of proper milieu, failure of axon path finding and synapse formation, etc. [23]. Due to multifaceted pathology of SCI, a single therapy is extremely unlikely to counter all of them. However, combining many different strategies is not only practically difficult but also reported to be counterproductive under certain combinations [24, 25]. Under these circumstances, transplantation of cells was considered as a single method which can tackle different pathomechanisms in one shot [26].
2 Cell Transplantation as a Treatment for SCI
Renaissance caused by embryonic stem cells discovery in biomedical research raised hopes about their usage in SCI also [27]. Despite positive reports from experimental studies [17, 28], clinical usage of them is being delayed. Several reasons, such as tumorigenic potential in vivo [29] and legal and ethical issues [30] associated with embryonic stem cells, stalled their migration from bench to bedside [31]. This prompted a search for alternate sources of stem cells.
3 Mesenchymal Stem (Stromal) Cells, Alternate Source of Stem Cells
In a pursuit to find alternatives to embryonic stem cells, cells present in the connective tissue component of several organs were thought to be resident stem cells. These stromal cells were named somewhat inappropriately as mesenchymal stem cells (MSCs) as the word “mesenchyme” refers to embryonic connective tissue, whereas adult connective tissue should be referred as “stroma.”
Mesenchymal stem cells were discovered in various adult tissues such as skin [32, 33], bone marrow [34], Wharton’s Jelly [35], amniotic fluid [36], dental pulp [37], etc. Woodbury et al. [34] had shown MSCs could be induced to adopt neural lineage and that kindled more interest in using these cells for SCI repair. MSCs derived from different sources have been tested in SCI [38] and some of them were found to be beneficial.
Discovery of MSC did not conclude therapy for SCI as there were negative reports about MSC usage for SCI [39]. MSCs were also not free from immunological rejection [40]. This would mean undesirable need for immunosuppressive therapy if MSCs are used. Thus, it would be better to identify a prospective cell type for SCI repair which can be procured in plenty, immunologically naïve, and biologically safe. In this context, placenta-derived cells find their place as they can be obtained in large quantities without ethical and legal concerns and are found to be immunologically nonantigenic.
4 Placenta as a Source of Stem Cells and Status of Amniotic Epithelial Cells
Placenta was found to harbor MSCs at various parts. Stromal cells derived from amniotic stroma [41, 42], decidua basalis and decidua parietalis [41], and umbilical cord blood cells [43] were shown to possess characteristic features of MSC. Through a series of publication, Sakuragawa et al. [44], reported neural and glial nature exhibited by amniotic epithelial cells which lead to the speculation of using these cells for treating neurological conditions. Later, amniotic epithelial cells were found to exhibit stem cell features [45] although amniotic epithelial cells do not have properties similar to MSCs.
5 Objective and Scope of This Chapter
It was intended to review the status of human amniotic epithelial cells (hAECs) for contusive SCI repair in the light of our own experiments conducted using hAEC in rat model of contusive SCI (unpublished work). We present here our observations which indicate high potentials of these cells in treating SCI. Scope of the phrase “amniotic epithelial cells” was limited to those epithelial cells derived directly from amniotic membrane and amniotic fluid cells are excluded. As amniotic fluid might contain not only epithelial cells shed off from amniotic membrane but also cells derived from the developing fetus, nature of amniotic fluid cells is ambiguous. Similarly, stromal cells obtainable from amnion (amniotic stromal cells) are also excluded as these cells express MSC features and not epithelial type features.
6 Our Experiments Using hAEC for Contusive SCI Repair in Rats
6.1 SCI Model in Rats and hAEC Transplantation
From full-term placenta obtained after elective caesarian deliveries, hAEC were isolated and cultured as per the procedures described elsewhere [46]. Cells were labeled with CellTracker Green (Invitrogen, USA) fluorescent label as per the manufacturer’s recommendations. Female Wistar albino rats (Rattus norvegicus) of 90 days of age (150–180 g of body weight) were used in the study. Animal housing and maintenance were as per recommendations given by Indian and International organizations such as Committee for the Purpose of Control and Supervision on Experiments on Animals (CPCSEA) and Canadian Council on Animal Care [47]. Protocol of the study was approved by Institutional Animal Ethical Committee, which was formed and functioning as per the guidelines of CPCSEA, Government of India.
Under surgical anesthesia, spinal cords were exposed by standard laminectomy at T9-T10 level. Contusion SCI was created by dropping a 10-g weight steel rod of 3 mm diameter from 12.5 mm height [2]. During weight drop, vertebral column was stabilized with clamps. Twenty minutes after creating injury, CellTracker Green labeled hAEC transplantation was carried out. Using microliter syringe, stereotaxically 4 μl of cell suspension at a concentration of 50,000 cells/μl was intraparenchymally injected.
Prophylactic antibiotics (amoxicillin + cloxacillin 20 mg/kg i.m.) and analgesic Pentazocine (1 mg/kg subcutaneous) were given for appropriate duration [48, 49]. Animals were cared for complications of SCI such as urinary tract infections, pressure sores, etc. In general, postoperative periods in all the animals were uneventful.
Animals were grouped as follows:
Sham group | Sham operated animals (n = 5) |
Lesion group | SCI by contusion as described above (n = 15) |
hAEC group | SCI followed by hAEC transplantation as described above (n = 15) |
In each group other than control, there were 15 animals out of which, 5 animals each were euthanized at the end of 2, 4, and 8 weeks of postoperative periods.
6.2 Evaluation of hAEC Transplantation Effects
Effects of hAEC transplantation for contusive SCI were evaluated using behavioral and histological/histochemical parameters. Motor recovery in animals was assessed using BBB scoring method [50]. Animals were euthanized by overdose of anesthesia and tissues were fixed using 4 % paraformaldehyde in phosphate-buffered saline. Both paraffin and cryosections were carried out at 10–15 μm thickness. Sections were used to visualize CellTracker Green labeled cells and for other staining such as antibody-based ED1, silver and CFV staining methods [51]. In long-term animals (>8 weeks), quantitative histology was carried out using standard principles of morphometric procedures [52] to count number of neurons present at C1, T1, T6, T12, and L1 levels. Neuronal density (i.e., number of neurons per cu.mm) was calculated using the formula as:


Where
N V is the numerical density or number of cells per cubic millimeter
N A is the average number of cells in the reference area
A is the area of the reference area in millimeter
t is the thickness of the section in millimeters
D is the mean diameter of the cells in millimeters
6.3 Statistical Analysis
All the numerical data were analyzed using SPSS software. Differences between mean values of the groups were compared for significance using one-way ANOVA with post hoc analysis applying Tukey’s test. Significance was tested at 0.05 level. Histograms represent mean values of the groups with±standard error of mean values.
7 Results
Postoperative period of the animals in general was uneventful. BBB scores of the hAEC transplanted animals in comparison with lesion group animals were given in Fig. 22.1. BBB score comprise of 21-point scale in which 0 represents complete paralysis and 21 indicates normal motor function in hind limbs. Spinal cord injured animals were with score zero immediately after lesions. However, there was a gradual recovery and by 8 weeks, hAEC transplanted animals reached a score of 15 which is about two points higher than lesion group. The difference was significant at 8 weeks postinjury/transplantation.


Fig. 22.1
BBB scores of control/sham operated animals remain at the maximum score in the scale, i.e., 21 points. Lesion and hAEC group animals initially were zero (totally paraplegic) in scale and showed gradual recovery over the period. From 4th week, hAEC group animals showed improved performance when compared with lesion group animals. However, the differences were statistically significant only at 8th week. Note: Lesion group values were from laboratory’s data source and were used in other presentations/publications
CellTracker Green was readily taken up by hAEC in culture and showed bright green fluorescence. hAEC was having either a round or slightly irregular morphology with a granular cytoplasmic appearance in vitro (Fig. 22.2). To discriminate between autofluorescing macrophage and CellTracker Green labeled hAECs, immunofluorescence study for macrophages was carried out using ED1 antibody. Also, hAEC transplantation carried out in uninjured animals served as control for such counter staining study. In uninjured cord, transplanted hAECs were seen to survive up to 2 weeks and there were no ED1 positive macrophages. In injury group, all the macrophages seen in green channel due to autofluorescence were positive for ED1. Interestingly, in hAEC group, there were cells seen in both green and red channels, i.e., macrophages and cells seen only in green channel which were negative for ED1. These cells were presumably the transplanted hAECs. These results are illustrated in Fig. 22.3.



Fig. 22.2
Smear of hAEC labeled with CellTracker Green fluorescent dye fixed with 4 % paraformaldehyde after being used for transplantation. Panel 1 shows fluorescent view of the labeled cells and Panel 2 showed trypan blue stained cells. hAEC appeared as brightly fluorescing cells with more or less round morphology and granular cytoplasm

Fig. 22.3
Immunofluorescence study for macrophages. CellTracker labeled hAECs were observed in uninjured spinal cord up to 2 weeks. In lesion group, autofluorescing macrophages seen in green channel were positive for ED1 as visualized through red channel. In hAEC group, in addition to cells seen in both green and red channels (autofluorescing macrophages which are positive for ED1), there were cells seen only in green channel (indicated by arrows in panel III.D). Since these green fluorescing cells are negative for ED1, they are presumed to be the CellTracker labeled transplanted hAECs. See text for details (Columns a, b, c represents green, red and blue channel views respectively. Column d represents merged view of green and red channel. Row labels are self explanatory)
Such green fluorescing ED1 negative cells were rare by 4 weeks and were not seen beyond that. Cresyl fast violet staining and silver staining showed cells with round morphology among host axons which were absent in lesion group animals (Fig. 22.4). These cells might be the transplanted hAECs. It was not known whether the cells lost their fluorescent label by 4 weeks of postinjury/transplantation period.


Fig. 22.4
Cresyl fast violet and silver staining carried out at 8 weeks of postoperative period showed cells appears to be hAECs in the transplantation group. (a) CFV staining of injury site in lesion group showing vacuolation and fibrous glial scar formation. (b) CFV staining of injury plus transplantation site in hAEC group showing cells with round morphology which were absent in lesion group at the comparable postoperative period. These cells present in hAEC group only were presumed to be the transplanted hAECs. Silver impregnation as per Glees and Marshland’s modification of Belchowsky’s method to stain axons showed extension of neurites among such cells seen in hAEC group (Panel c). Panel d illustration represents enlarged view of boxed area in Panel c showing traversing axons (arrow heads) and cells with outgrowths (arrows). Such appearance was not seen in lesion group animals
Numerical densities of neurons at various spinal segments were given in Fig. 22.5. Lesion group animals showed significant reduction in neurons when compared with control animals. In hAEC group, neuronal densities were less when compared with control animals; nevertheless, they were significantly higher than lesion group animals.


Fig. 22.5
Neuronal densities at various levels of spinal cords during long-term period (>8 weeks). There was significant loss of neurons not only at segments close to lesion viz. T6 and T12 but also at distant segments as far as C1. hAEC transplantation was found to rescue neurons from death with restoration to near normal levels at T1, T6, and T12 levels. However at L1 level, hAEC transplantation did not show rescue effect on neurons. See text for details
8 Discussion
8.1 About the Use of hAEC for SCI Repair
Concept of using hAEC for CNS disorders was probably inspired by the discovery of neural and glial markers expression by amniotic epithelial cells [44]. They were suggested to be a potential cell type for intracerebral transplantation purposes based on their surface expression of neural tissue-specific proteins and their capability to secrete neurotransmitters [53, 54]. Miki et al. [45] report about stem cell properties of hAEC justified pilot animal studies of using hAEC in different neurological disease models.
AEC transplantation in the Parkinson model of rats was found to reverse the condition and prevent death in neurons [55–57]. When transplanted into ischemic hippocampus, they were observed to differentiate into “neuron-like” cells [58].
There were only a few experimental studies in which hAEC was used to treat SCI. Earliest study was from our lab [59] in which, hAEC transplantation after spinal cord transection in monkeys was found to offer neuroprotection and helped in host regeneration. Later, Zhi-yuan et al. [60] reported improved locomotor function in spinal cord transected rats after hAEC transplantation. Cotransplantation of bFGF-expressing amniotic epithelial cells and neural stem cells were reported to promote recovery in spinal cord injured rats [61]. Recently, Xue et al. [62] reported combination of a cellular muscle scaffold with amniotic epithelial cells promote axonal regeneration, better myelination and reduced astrocytic scar formation. Amniotic epithelial cells were found to alleviate mechanical allodynia but not thermal hyperalgesia in spinal cord injured rats.
Surprisingly, except for the above-mentioned studies, there was no other study in which amniotic epithelial cells were tested for SCI repair. Except for our unpublished data presented here, and the work of Meng et al. [61], there were no studies in which amniotic epithelial cells were used in contusive SCI models. Given that Meng et al. [61] had used genetically modified AEC, except for our data presented here, there was no study available about the efficacy of AEC in treating contusive SCI.
8.2 Effect of hAEC Transplantation in Locomotor Function Recovery After SCI
In our study, we have observed improved BBB scores in transplantation group animals when compared with lesion group by 2–4 weeks albeit the differences were statistically insignificant. Nevertheless, by 8 weeks hAEC transplanted animals were showing better recovery and the difference was statistically significant.
Earlier improvement in BBB scores after cell transplantations was considered as the neuroprotective effect of the transplanted cells [63]. Therefore, it was logical to speculate the neuroprotective effect of hAEC transplantation albeit lack of statistical significance in short term. Continued improvement in BBB scores during late phase was reported to be associated with regeneration and improved function of long tracts [64]. Given that beneficial effects were seen in both early and late phases of recovery, it appears both neuroprotection (in short term) and regeneration of axons (in long term) might be the cause for the improved locomotor function in hAEC transplanted animals.
8.3 Effects of hAEC Transplantation at Tissue Level After SCI
Qualitative histology using different stains showed less vacuolation in hAEC group indicating reduction in necrosis and tissue loss. In hAEC group animals, axons were seen traversing through cells at the transplantation site and such scenario was not observed in lesion group animals. This corroborate with our earlier report [59] about possible host regeneration stimulated by transplanted hAECs. Numerical density of neurons is considered as one of the indications of neuroprotection [65–68]. In our quantitative histological studies, increased numerical density of neurons at various levels observed in hAEC group animals shall be a clear indication of neuroprotection offered by these cells. Zhi-yuan et al. [60] also reported similar findings of improved neuronal count after hAEC transplantation in SCI rats.
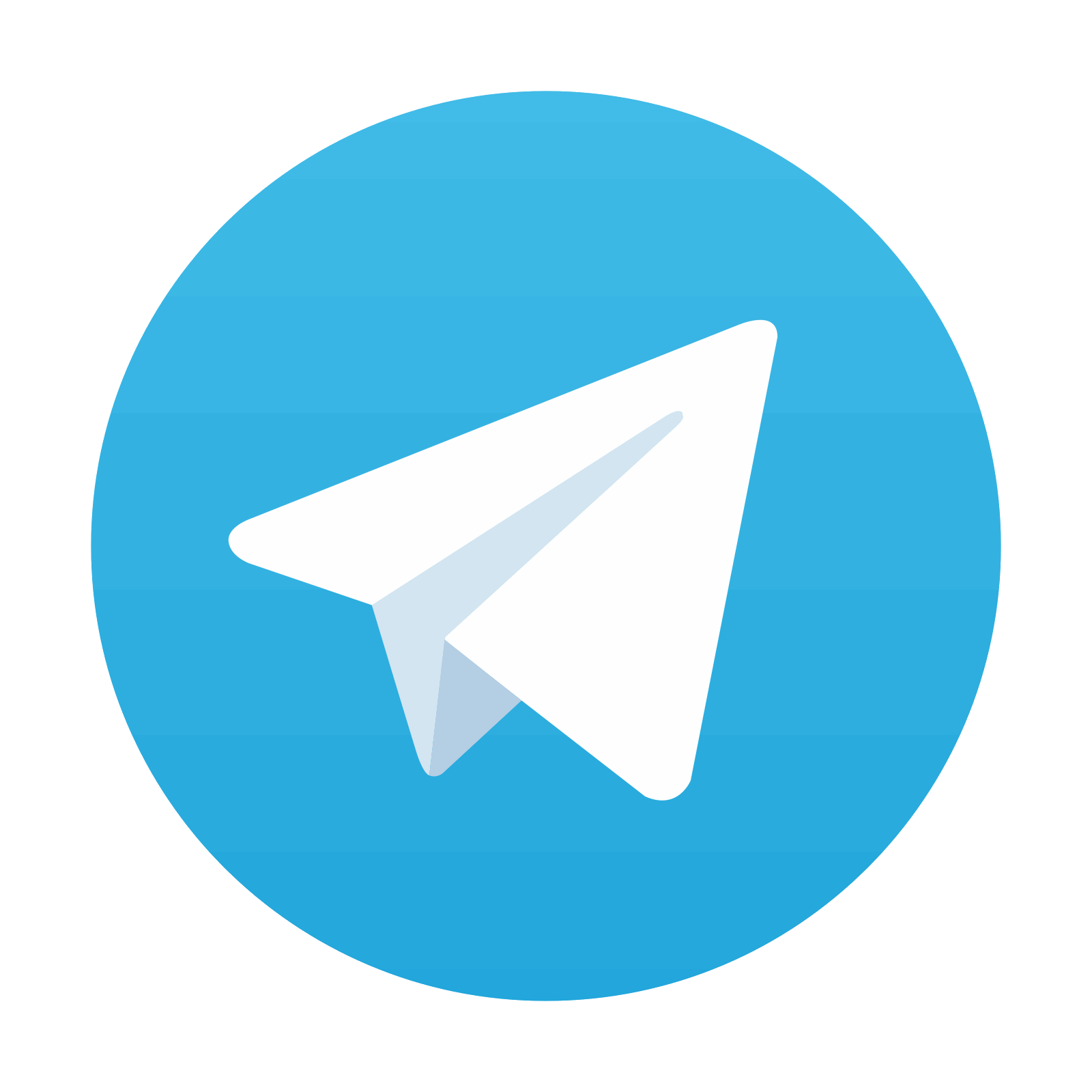
Stay updated, free articles. Join our Telegram channel

Full access? Get Clinical Tree
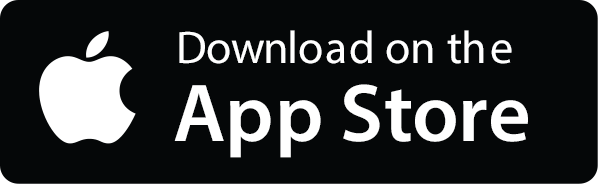
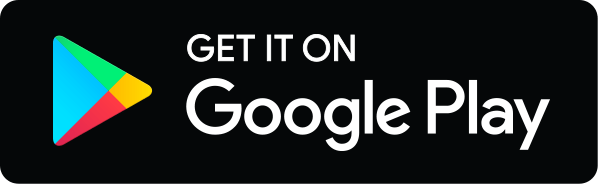