Chapter 7
Hormones
7.1 Major types of hormone receptors
- G protein-coupled receptors
– Many peptide hormones: hypothalamic and hypophyseal control hormones, glucagon
– Epinephrine, norepinephrine
- Receptor tyrosine kinases
– Insulin
– Growth hormone and growth factors
- Nuclear hormone receptors
– Steroid hormones
– Thyroid hormones
Notes: Hormones are fundamental to metabolic and other physiological regulation. Accordingly, hormone receptors, as well as enzymes involved in hormone synthesis, are important drug targets.
We have already encountered numerous GPCRs, and we have met some nuclear hormone receptors in the context of drug metabolism, namely the pregnane X receptor and the retinoid X receptor.
The receptor tyrosine kinases (RTKs) are another major receptor class. Like GPCRs, these receptors sit in the cytoplasmic membrane, bind their ligands from the extracellular side, and transmit the signal to the cell interior as a conformational change. However, unlike GPCRs, the RTKs do not transmit their signal through noncovalent interaction with downstream adapters. Instead, the activated receptors themselves function as protein kinases. RTKs typically phosphorylate multiple substrate proteins, which then noncovalently bind and activate various downstream regulatory proteins.
Important examples of the RTK class are the receptors for insulin and for growth hormone. Several other receptor tyrosine kinases are involved in tumor initiation and growth. We will learn a bit more about these in Chapter 12.
7.2 The hypothalamic-pituitary axis
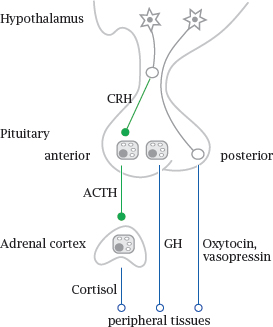
Notes: Hormone-secreting glands are also referred to as endocrine glands; this name reflects the fact that secretion occurs into the body interior, that is, into the bloodstream. In contrast, exocrine glands are the ones that secrete their products across the skin or mucous membranes.
Many of the endocrine glands are under control by the hypophyseal gland or pituitary, which in turn is controlled by the hypothalamus. These two are located next to each other at the base of the brain. The hypothalamus is itself part of the brain, whereas the hypophyseal gland extends downward from it. Hypothalamic neurons extend their axon endings into the hypophyseal gland, which peeks out of the blood brain barrier and is able to release hormones into the systemic circulation; one could say that the hypophyseal gland is an outlet for the brain through which it releases hormones to the body.
The hypophyseal gland consists of an anterior and a posterior lobe. The posterior lobe contains just the axon endings of hypothalamic nerve cells, which secrete two related peptide hormones, oxytocin and vasopressin, directly into the circulation.
The anterior pituitary lobe contains gland cells that are controlled by specific hypothalamic peptide hormones, for example corticotropin releasing hormone (CRH). In response to these hypothalamic hormones, the cells of the anterior pituitary release peptide hormones that stimulate peripheral glands, such as adrenocorticotropic hormone (ACTH), which stimulates the cortex of the adrenal glands, or thyroid-stimulating hormone (TSH), which controls the thyroid gland. In addition to hormones that control endocrine glands, the anterior pituitary also produces prolactin, which stimulates the mammary glands, as well as growth hormone (GH).1
7.2.1 Peripheral glands and hormones controlled by anterior pituitary
Gland | stimulated by | hormones produced |
Thyroid gland | thyroid-stimulating hormone (TSH) | tri-, tetraiodothyronine (T3, T4) |
Adrenal glands (cortex) | adrenocorticotropic hormone (ACTH) | gluco-, mineralocorticoids; androgens |
Testicles / ovaries | follicle-stimulating hormone (FSH), luteinizing hormone (LH) | androgens, estrogens, progestins |
Diffuse Mammary gland | growth hormone prolactin | growth factors — |
7.2.2 Regulatory patterns in the hypothalamic-pituitary axis
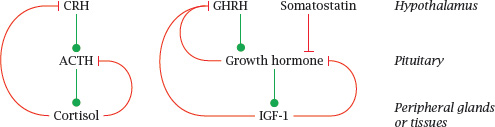
Notes: This scheme illustrates two different regulatory patterns in the hypothalamic-pituitary axis. The production of cortisol by the adrenal glands is stimulated by adrenocorticotropic hormone (ACTH), which in turn is stimulated by hypothalamic corticotropin-releasing hormone (CRH). Cortisol exercises negative feedback on both. The control of the thyroid gland by thyroid-stimulating hormone (TSH) and thyrotropin-releasing hormone (TRH) works analogously, as does the regulation of sexual hormone secretion by endocrine cells in the ovaries and testicles (see later).
The secretion of growth hormone (GH) by the anterior pituitary is controlled by two hypothalamic hormones; it is activated by growth hormone releasing hormone (GHRH) and inhibited by somatostatin (SST). GH itself stimulates the production of insulin-like growth factor 1 (IGF-1) in the liver and other tissues. Both GH itself and IGF-1 exert negative feedback on GH secretion.
Keep in mind that these homeostatic feedback loops are only part of the story. Spontaneous variations in hormone levels, such as the early-morning high of cortisol, the spike of growth hormone secretion shortly after onset of sleep, or the variations of estrogens and progestins that occur during the menstrual cycle, involve additional control mechanisms.
7.2.3 The posterior lobe of the hypophyseal gland produces oxytocin and vasopressin
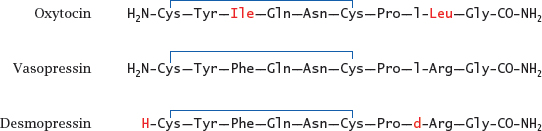
Notes: Both hormones act on GPCRs that couple prominently to the PLC cascade. Oxytocin drives the contraction of smooth muscle in the uterus during labor; it is also used as a drug to induce labor when the spontaneous onset of labor is delayed.2 It is also released during breast feeding and induces contraction of smooth muscle in the milk ducts of the mammary glands, which assists in the ejection of milk.
Vasopressin, also known as antidiuretic hormone, activates smooth muscle in the circulation; it also acts on cells in the distal tubules of the kidney, where it promotes the preservation of water. It is used as a drug in individuals with deficient endogenous production.
Desmopressin is a synthetic vasopressin analogue. All amino acid residues are in the L configuration, except the D-arginine in desmopressin. In oxytocin and vasopressin, the amino group at the C-terminus is derived from a glycine that gets oxidatively truncated after proteolytic cleavage of the precursor polypeptide.
7.3 Thyroid hormones
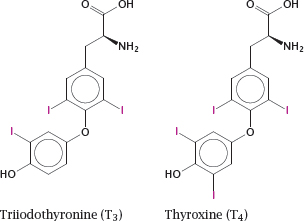
Notes: The thyroid hormones triiodothyronine (T3) and thyroxine (tetraiodothyronine, T4) are produced by the thyroid gland, which is under the control of the thyroid-stimulating hormone from the anterior pituitary. These hormones have broad and diverse activating effects on human physiology, which are mediated through transcriptional regulation. For example, they increase the expression of β-adrenergic receptors, boosting heart rate and ejection volume, and of respiratory chain-uncoupling proteins, which cause metabolic substrates to be burned without ATP production.3
Lack of thyroid hormones in children causes delayed and stunted physical and mental development. If diagnosed in time, this can be corrected by hormone substitution treatment.
7.3.1 Thyroid hormones activate cognate nuclear hormone receptors
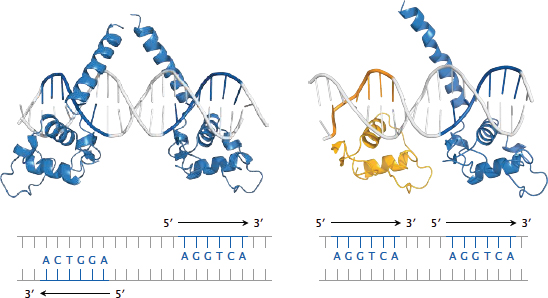
Notes: The thyroid hormones activate cognate nuclear hormone receptors; these are similar to the pregnane X receptor (see slide 4.3) but bind to different recognition elements. The thyroid hormone receptors come in α and β edition, and further diversification arises from the formation of various receptor homo- or heterodimers, which then bind to different recognition sequences on the DNA.
This slide illustrates the recognition of specific DNA sequences (thyroid hormone response elements) by thyroid receptor (TRβ, blue) and retinoid X receptor (RXRα, yellow). Both monomeric receptors recognize the same sequence element—the hexanucleotide AGGTCA. However, different receptor dimers recognize different target sequences nevertheless; this is due to variations in the relative orientations of the DNA-binding domains within these dimers.
Left: The TRβ homodimer recognizes two instances of the consensus sequence that occur on opposite strands and point away from one another; such sequence motifs are referred to as inverted palindromes.
Right: The TRβ-RXRα heterodimer recognizes two instances of the consensus sequence that are located on the same strand (direct repeats). In both panels, only the DNA-binding domains of the receptor molecules are shown; the remaining domains would attach to the tips of the upward pointing helices.
7.3.2 Tissue structure of the thyroid gland, and localization of hormone synthesis
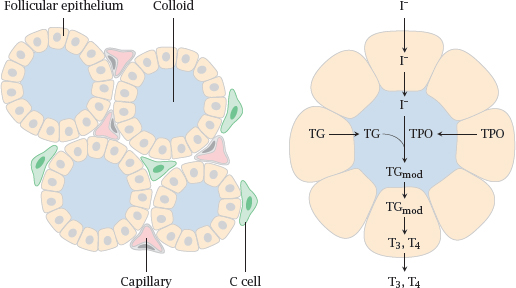
Notes: The thyroid gland has a peculiar tissue structure that is related to its likewise unusual biochemical function. The crucial and characteristic steps in thyroid hormone synthesis occur in confined extracellular compartments, little “reactors”, which are referred to as thyroid follicles.
Left: Follicular epithelial cells enclose the follicle lumen, which is filled with protein-rich colloid. C-cells are located outside the follicles; they are unrelated to T3 and T4 synthesis but instead produce the peptide hormone calcitonin (see slide 7.5.2).
Right: The cells of the follicular epithelium cells synthesize thyroglobulin (TG) and thyroid peroxidase (TPO) and secrete them into the follicle lumen. They also take up iodide and transport it into the follicle lumen, where it is used by thyroid peroxidase for the iodination of tyrosine side chains of thyroglobulin. Thyroid peroxidase also catalyzes the subsequent step, in which it joins two iodinated tyrosine residues (see slide 7.3.4).
The peroxidase-modified thyroglobulin (TGmod) is taken up into the follicle cells again via endocytosis and proteolytically degraded, which yields the free hormones triiodothyronine (T3) and thyroxine (T4).
7.3.3 Tyrosine side chain iodination by thyroid peroxidase
Notes: In the biosynthesis of the thyroid hormones, the first step is the iodination of tyrosyl residues in thyroglobulin. Thyroid peroxidase contains a heme moiety that generates hypoiodite from iodide and H2O2. The hypoiodite then reacts with the hydroxyphenyl side chain of tyrosine.
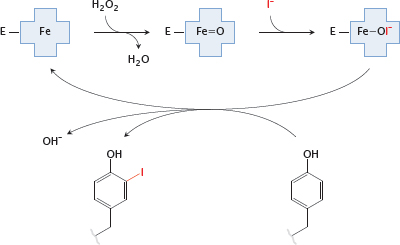
7.3.4 Coupling of two iodinated tyrosine side chains
Notes: The coupling reaction between two iodinated tyrosyl side chains is also catalyzed by thyroid peroxidase. The enzyme abstracts an electron from each of the substrate side chains, which then undergo radical recombination. The reaction leaves a dehydroalanine residue in the protein backbone.
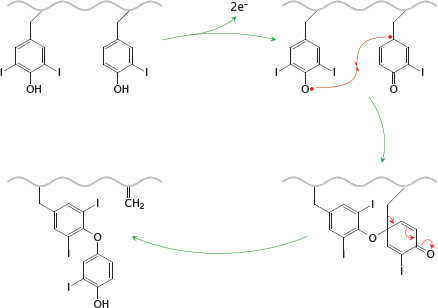
The slide shows the reaction of a diiodotyrosine residue with a monoiodotyrosine side chain; this will produce a precursor of T3. The same reaction can also occur between two diiodotyrosine side chains, which will produce a T4 precursor. After reuptake of the modified thyroglobulin by the follicular epithelial cells, T3 and T4 are liberated simply by proteolytic breakdown of the protein.
Both T3 and T4 are secreted into the bloodstream. Both are able to activate thyroid hormone receptors; however, T3 is much more active. T4 can be enzymatically deiodinated to T3 and thus functions mostly as a reservoir of the latter. Inhibition of this deiodination step by drugs—the antiarrhythmic drug amiodarone (see slide 7.3.6) in particular—causes a functional thyroid hormone deficit, although the combined serum levels of T3 and T4 will be normal.
7.3.5 Thyroid hormones and pharmacotherapy
- Goiter: iodine supplementation
- Hyperthyroidism due to anti-TSH-receptor autoantibodies or hormone-producing tumors:
– thyroid peroxidase inhibitors< div class='tao-gold-member'>Only gold members can continue reading. Log In or Register a > to continueStay updated, free articles. Join our Telegram channel

Full access? Get Clinical Tree
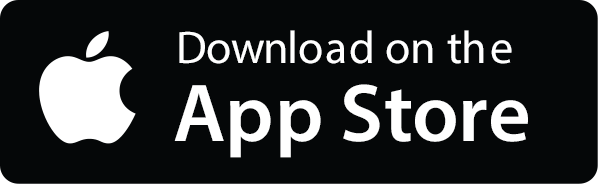
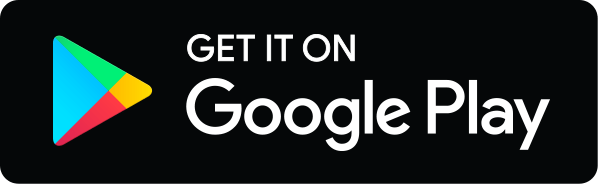