CHAPTER 39 Hormonal Regulation of Calcium and Phosphate Metabolism
C alcium (Ca++) and phosphate are essential to human life because they play important structural roles in hard tissues (i.e., bones and teeth) and important regulatory roles in metabolic and signaling pathways. In blood, most phosphate exists in the ionized form of phosphoric acid, which is called inorganic phosphate (Pi). The two primary sources of circulating Ca++ and Pi are the diet and the skeleton (Fig. 39-1). Two hormones, 1,25-dihydroxyvitamin D (also called calcitriol) and parathyroid hormone (PTH), regulate intestinal absorption of Ca++ and Pi and release of Ca++ and Pi into the circulation after bone resorption. The primary processes for removal of Ca++ and Pi from blood are renal excretion and bone formation (Fig. 39-1). 1,25-Dihydroxyvitamin D and PTH regulate both processes. Other hormones and paracrine growth factors also regulate Ca++ and Pi homeostasis.
CRUCIAL ROLES OF CALCIUM AND PHOSPHATE IN CELLULAR PHYSIOLOGY
Calcium is an essential dietary element. In addition to obtaining Ca++ from the diet, humans contain a vast store (i.e., > 1 kg) of Ca++ in their bones, which can be called on to maintain normal circulating levels of Ca++ in times of dietary restriction and during the increased demands of pregnancy and nursing. Circulating Ca++ exists in three forms (Table 39-1): free ionized Ca++, protein-bound Ca++, and Ca++ complexed with anions (e.g., phosphates, HCO3−, citrate). The ionized form represents about 50% of circulating Ca++, and because this form is so critical to many cellular functions, [Ca++] in both the extracellular and intracellular compartments is tightly controlled. Circulating Ca++ is under direct hormonal control and normally maintained in a relatively narrow range. Either too little Ca++ (hypocalcemia; total serum [Ca++] below 8.5 mg/dL [4.2 mEq/L]) or too much Ca++ (hypercalcemia; total serum [Ca++] above 10.5 mg/dL [5.2 mEq/L]) in blood can lead to a broad range of pathophysiological changes, including neuromuscular dysfunction, central nervous system dysfunction, renal insufficiency, calcification of soft tissue, and skeletal pathology.
Pi is also an essential dietary element, and it is stored in large quantities in bone complexed with Ca++. Most circulating Pi is in the free ionized form, but some Pi (< 20%) circulates as a protein-bound form or is complexed with cations (Table 39-1). Because soft tissues contain 10-fold more Pi than Ca++, tissue damage (e.g., crush injury with massive muscle cell death) can result in hyperphosphatemia, whereupon the increased Pi complexes with Ca++ to cause acute hypocalcemia.
PHYSIOLOGICAL REGULATION OF CALCIUM AND PHOSPHATE: PARATHYROID HORMONE AND 1,25-DIHYDROXYVITAMIN D
Parathyroid Glands
The predominant parenchymal cell type in the parathyroid gland is the principal (also called chief) cell (Fig. 39-2).
Parathyroid Hormone
Structure, Synthesis, and Secretion
PTH is secreted as an 84–amino acid polypeptide and is synthesized as a prepro-PTH, which is proteolytically processed to pro-PTH in the endoplasmic reticulum and then to PTH in the Golgi and secretory vesicles. Unlike proinsulin, all intracellular pro-PTH is normally converted to PTH before secretion. PTH has a short half-life (<5 minutes).
The primary signal that stimulates PTH secretion is low circulating [Ca++] (Fig. 39-3). The extracellular [Ca++] is sensed by the parathyroid chief cell through a Ca++-sensing receptor (CaSR). In the parathyroid gland, increasing amounts of extracellular Ca++ bind to the CaSR and activate signaling pathways that repress PTH secretion.
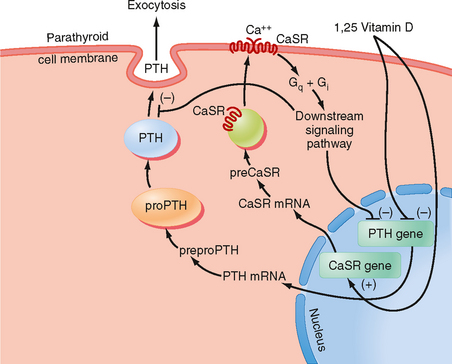
Figure 39-3 Regulation of PTH gene expression and secretion.
(Modified from Porterfield SP, White BA: Endocrine Physiology, 3rd ed. Philadelphia, Mosby, 2007.)
Although the CaSR binds to extracellular Ca++ with relatively low affinity, the CaSR is extremely sensitive to changes in extracellular [Ca++]. A 0.2-mEq/L drop in blood [Ca++] produces an increase in circulating PTH levels from basal (5% of maximum) to maximum levels (Fig. 39-4). Thus, the CaSR regulates PTH output in response to subtle fluctuations in [Ca++] on a minute-to-minute basis.
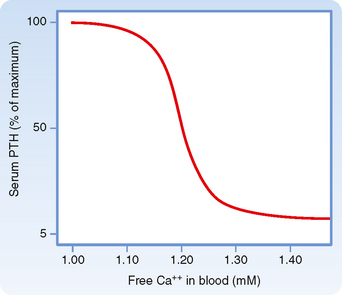
Figure 39-4 Ca++/PTH secretion dose-response curve.
(Modified from Porterfield SP, White BA: Endocrine Physiology, 3rd ed. Philadelphia, Mosby, 2007.)
PTH production is also regulated at the level of gene transcription (Fig. 39-3). The PTH gene is repressed by a calcium response element within the promoter of this gene. Thus, the signaling pathway that is activated by binding of Ca++ to the CaSR ultimately leads to repression of PTH gene expression and synthesis. The PTH gene is also repressed by 1,25-dihydroxyvitamin D (acting through vitamin D response elements’see later). The ability of 1,25-dihydroxyvitamin D to hold PTH gene expression in check is reinforced by the coordinated up-regulation of CaSR gene expression by positive vitamin D response elements in the promoter region of the CaSR gene (Fig. 39-3).
Vitamin D
Vitamin D is actually a prohormone that must undergo two successive hydroxylation reactions to become the active form 1,25-dihydroxyvitamin D (Fig. 39-5). Vitamin D plays a critical role in Ca++ absorption and, to a lesser extent, Pi absorption by the small intestine. Vitamin D also regulates bone remodeling and renal reabsorption of Ca++ and Pi.
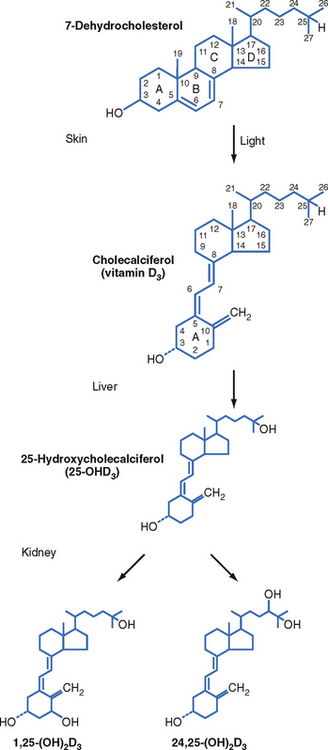
Figure 39-5 Biosynthesis of 1,25-dihydroxyvitamin D.
(Modified from Porterfield SP, White BA: Endocrine Physiology, 3rd ed. Philadelphia, Mosby, 2007.)
Structure, Synthesis, and Transport of Active Vitamin D Metabolites
Vitamin D3 (also called cholecalciferol) is synthesized via the conversion of 7-dehydrocholesterol by ultraviolet B light (UVB) in the more basal layers of the skin (Fig. 39-6). Vitamin D3 is therefore referred to as a secosteroid, which is a class of steroids in which one of the cholesterol rings is opened (Fig. 39-5). Vitamin D2 is produced in plants. Vitamin D3 and, to a lesser extent, vitamin D2 are absorbed from the diet and are equally effective after conversion to active hydroxylated forms. The balance between UVB-dependent, endogenously synthesized vitamin D3 and absorption of the dietary forms of vitamin D becomes important in certain situations. Individuals with higher epidermal melanin content who live in higher latitudes convert less 7-dehydrocholesterol to vitamin D3 and thus are more dependent on dietary sources of vitamin D3. Dairy products are enriched with vitamin D3, but not all individuals tolerate or enjoy dairy products. Institutionalized, sedentary elderly patients who stay indoors and avoid dairy products are particularly at risk for the development of vitamin D3 deficiency.
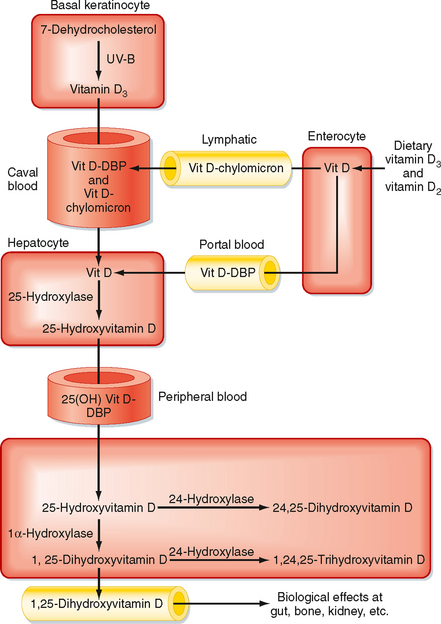
Figure 39-6 Vitamin D metabolism.
(Modified from Porterfield SP, White BA: Endocrine Physiology, 3rd ed. Philadelphia, Mosby, 2007.)
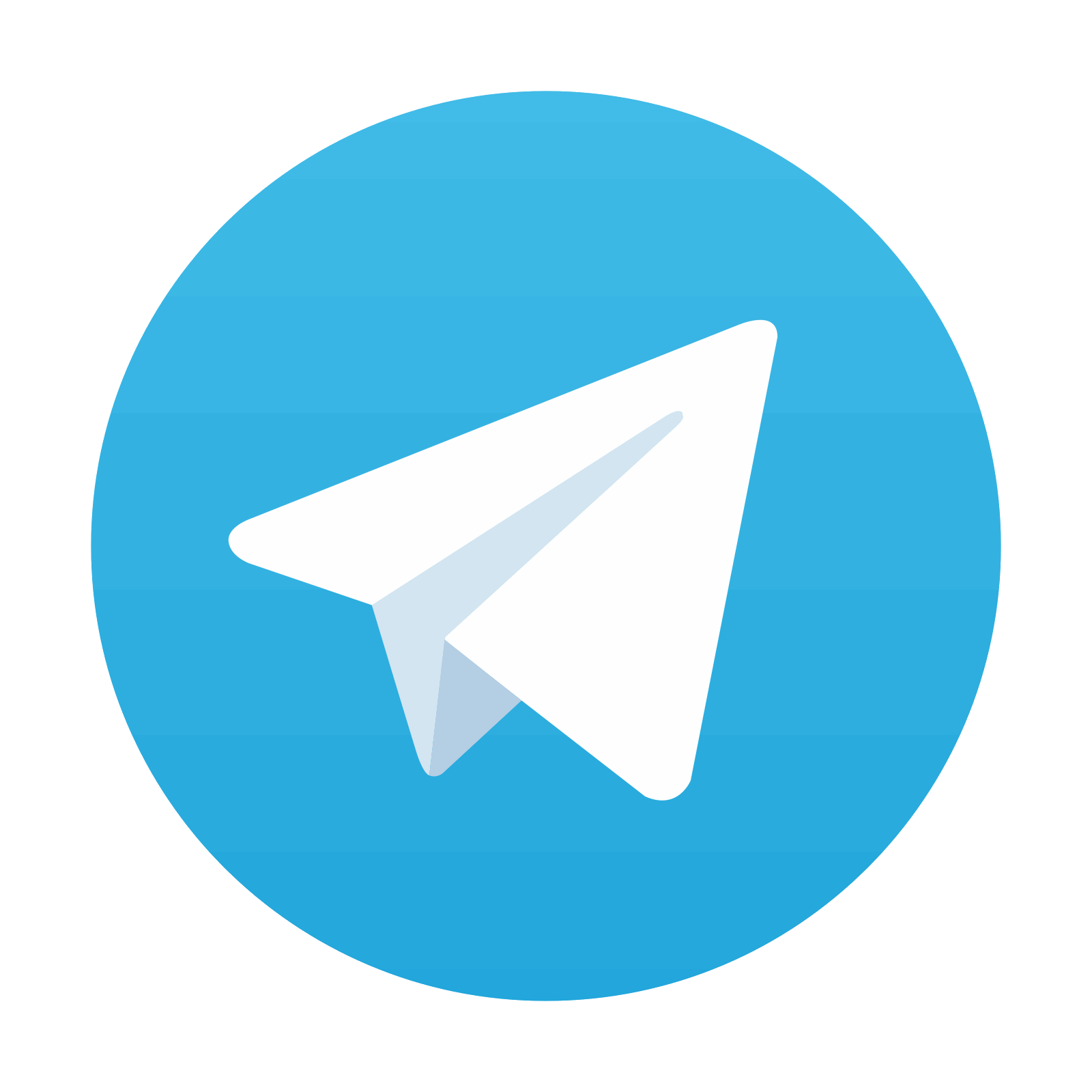
Stay updated, free articles. Join our Telegram channel

Full access? Get Clinical Tree
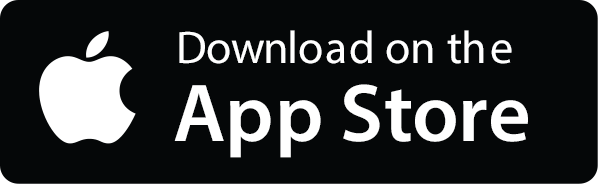
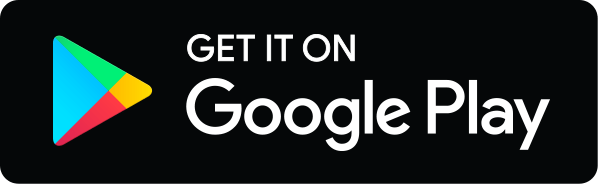