Histamine, Bradykinin, and Their Antagonists
Histamine is a major mediator of inflammation, anaphylaxis, and gastric acid secretion; in addition, histamine plays a role in neurotransmission. Our understanding of the physiological and pathophysiological roles of histamine has been enhanced by the development of subtype-specific receptor antagonists and by the cloning of 4 receptors for histamine. Competitive antagonists of H1 receptors are used therapeutically in treating allergies, urticaria, anaphylactic reactions, nausea, motion sickness, insomnia, and some symptoms of asthma. Antagonists of the H2 receptor are effective in reducing gastric acid secretion. The peptide, bradykinin, has cardiovascular effects similar to those of histamine and plays prominent roles in inflammation and nociception.
HISTAMINE
Histamine is a hydrophilic molecule consisting of an imidazole ring and an amino group connected by an ethylene group, biosynthesized from histidine by decarboxylation (Figure 32–1). The 4 histamine receptors, all GPCRs, can be differentially activated by analogs of histamine and inhibited by specific antagonists (Table 32–1).
Table 32–1
Characteristics of Histamine Receptors
Figure 32–1 Histamine synthesis and metabolism in humans. Histamine is synthesized from histidine by decarboxylation. Histamine is metabolized via 2 pathways, predominantly by methylation of the ring followed by oxidative deamination (left side of figure), and secondarily by oxidative deamination and then conjugation with ribose. These metabolites have little or no activity and are excreted in the urine. Measurement of urinary N-methylhistamine affords a reliable index of histamine production. Artifactually elevated levels of histamine in urine arise from genitourinary tract bacteria that can decarboxylate histidine. MAO, monoamine oxidase.
DISTRIBUTION AND BIOSYNTHESIS
DISTRIBUTION. Almost all mammalian tissues contain histamine in amounts ranging from <1 to >100 μg/g. Concentrations in plasma and other body fluids generally are very low, but human cerebrospinal fluid (CSF) contains significant amounts. The concentration of histamine is particularly high in tissues that contain large numbers of mast cells, such as skin, bronchial mucosa, and intestinal mucosa.
SYNTHESIS, STORAGE, AND METABOLISM. Histamine is formed by the decarboxylation of the amino acid histidine by the enzyme L-histidine decarboxylase (see Figure 32–1). Mast cells and basophils synthesize histamine and store it in secretory granules. At the secretory granule pH of ~5.5, histamine is positively charged and ionically complexed with negatively charged acidic groups on other granule constituents, primarily proteases and heparin or chondroitin sulfate proteoglycans. The turnover rate of histamine in secretory granules is slow. Non–mast cell sites of histamine formation include the epidermis, the gastric mucosa, neurons within the CNS, and cells in regenerating or rapidly growing tissues. Turnover is rapid at these non–mast cell sites because the histamine is released continuously rather than stored. Non–mast cell sites of histamine production contribute significantly to the daily excretion of histamine metabolites in the urine. Because L-histidine decarboxylase is an inducible enzyme, the histamine-forming capacity at such sites is subject to regulation. Histamine that is ingested is rapidly metabolized, and the metabolites are eliminated in the urine.
RELEASE AND FUNCTIONS OF ENDOGENOUS HISTAMINE
Histamine is released from storage granules as a result of the interaction of antigen with immunoglobulin E (IgE) antibodies on the mast cell surface. Histamine plays a central role in immediate hypersensitivity and allergic responses. The actions of histamine on bronchial smooth muscle and blood vessels account for many of the symptoms of the allergic response. In addition, some drugs act directly on mast cells to release histamine, causing untoward effects. Histamine has a major role in regulating gastric acid secretion and also modulates neurotransmitter release.
ROLE IN ALLERGIC RESPONSES. The principal target cells of immediate hypersensitivity reactions are mast cells and basophils. As part of the allergic response to an antigen, IgE antibodies are generated and bind to the surfaces of mast cells and basophils via specific high-affinity Fc receptors. This receptor, FcεRI, consists of α, β, and 2 γ chains (see Chapter 35). Antigen bridges the IgE molecules and via FcεRI activates signaling pathways in mast cells or basophils involving tyrosine kinases and subsequent phosphorylation of multiple protein substrates within 5-15 sec of contact with antigen. These events trigger the exocytosis of the contents of secretory granules.
RELEASE OF OTHER AUTACOIDS. Stimulation of IgE receptors also activates phospholipase A2 (PLA2), leading to the production of a host of mediators, including platelet-activating factor (PAF) and metabolites of arachidonic acid such as leukotrienes C4 and D4, which contract the smooth muscles of the bronchial tree.
HISTAMINE RELEASE BY DRUGS, PEPTIDES, VENOMS, AND OTHER AGENTS. Many compounds, including a large number of therapeutic agents, stimulate the release of histamine from mast cells directly and without prior sensitization. Responses of this sort are most likely to occur following intravenous injections of certain categories of substances. Tubocurarine, succinylcholine, morphine, some antibiotics, radiocontrast media, and certain carbohydrate plasma expanders also may elicit the response. The phenomenon is one of clinical concern, and may account for unexpected anaphylactoid reactions. Basic polypeptides often are effective histamine releasers, and over a limited range, their potency generally increases with the number of basic groups. For example, bradykinin is a poor histamine releaser, whereas kallidin (Lys-bradykinin) and substance P, with more positively charged amino acids, are more active. Some venoms, such as that of the wasp, contain potent histamine-releasing peptides. Basic polypeptides released upon tissue injury constitute pathophysiological stimuli to secretion for mast cells and basophils.
Within seconds of the intravenous injection of a histamine liberator, human subjects experience a burning, itching sensation. This effect, most marked in the palms of the hand and in the face, scalp, and ears, is soon followed by a feeling of intense warmth. The skin reddens, and the color rapidly spreads over the trunk. Blood pressure falls, the heart rate accelerates, and the subject usually complains of headache. After a few minutes, blood pressure recovers, and crops of hives usually appear on the skin. Colic, nausea, hypersecretion of acid, and moderate bronchospasm also frequently occur. Histamine liberators do not deplete tissues of non–mast cell histamine.
INCREASED PROLIFERATION OF MAST CELLS AND BASOPHILS AND GASTRIC CARCINOID TUMORS. In urticaria pigmentosa (cutaneous mastocytosis), mast cells aggregate in the upper corium and give rise to pigmented cutaneous lesions that sting when stroked. In systemic mastocytosis, overproliferation of mast cells also is found in other organs. Patients with these syndromes suffer a constellation of signs and symptoms attributable to excessive histamine release, including urticaria, dermographism, pruritus, headache, weakness, hypotension, flushing of the face, and a variety of GI effects, such as diarrhea or peptic ulceration. Gastric carcinoid tumors secrete histamine, which is responsible for episodes of vasodilation as part of the patchy “geographical” flush.
GASTRIC ACID SECRETION. Histamine acting at H2 receptors is a powerful gastric secretagogue, evoking a copious secretion of acid from parietal cells (see Figure 45–1); it also increases the output of pepsin and intrinsic factor. The secretion of gastric acid from parietal cells also is caused by stimulation of the vagus nerve and by the enteric hormone gastrin. However, histamine undoubtedly is the dominant physiological mediator of acid secretion; blockade of H2 receptors not only antagonizes acid secretion in response to histamine but also inhibits responses to gastrin and vagal stimulation (see Chapter 45).
CNS. Histamine-containing neurons control both homeostatic and higher brain functions, including regulation of the sleep-wake cycle, circadian and feeding rhythms, immunity, learning, memory, drinking, and body temperature. However, no human disease has yet been directly linked to dysfunction of the brain histamine system. Histamine, histidine decarboxylase, enzymes that metabolize histamine, and H1, H2, and H3 receptors are distributed widely but non-uniformly in the CNS. H1 receptors are associated with both neuronal and non-neuronal cells and are concentrated in regions that control neuroendocrine function, behavior, and nutritional state. Distribution of H2 receptors is more consistent with histaminergic projections than H1 receptors, suggesting that they mediate many of the postsynaptic actions of histamine. H3 receptors also are concentrated in areas known to receive histaminergic projections, consistent with their function as presynaptic autoreceptors. Histamine inhibits appetite and increases wakefulness via H1 receptors.
PHARMACOLOGICAL EFFECTS
RECEPTOR–EFFECTOR COUPLING AND MECHANISMS OF ACTION. Histamine receptors are GPCRs, coupling to second messenger systems and producing effects as noted in Table 32–1.
H3 and H4 receptors have a much higher affinity for histamine than do H1 and H2 receptors. Activation of H3 receptors also can activate MAP kinase and inhibit the Na+/H+ exchanger; activation of H4 receptors mobilizes stored Ca2+ in some cells. Activation of H1 receptors on vascular endothelium stimulates eNOS to produce nitric oxide (NO), which diffuses to nearby smooth muscle cells to increase cyclic GMP and cause relaxation. Stimulation of H1 receptors on smooth muscle will mobilize Ca2+ and cause contraction, whereas activation of H2 receptors on the same smooth muscle cell will link via Gs to enhanced cyclic AMP accumulation, activation of PKA, and thence to relaxation. Pharmacological definition of H1, H2, and H3 receptors is clear because relatively specific agonists and antagonists are available. However, the H4 receptor exhibits 35-40% homology to isoforms of the H3 receptor, and the 2 were harder to distinguish pharmacologically. Several non-imidazole compounds that are more selective H3 antagonists have been developed, and there are now several selective H4 antagonists. 4-Methylhistamine and dimaprit, previously identified as specific H2 agonists, are actually more potent H4 agonists.
H1 AND H2 RECEPTORS. H1 and H2 receptors are distributed widely in the periphery and in the CNS. Histamine causes itching and stimulates secretion from nasal mucosa. It contracts many smooth muscles, such as those of the bronchi and gut, but markedly relaxes others, including those in small blood vessels. Histamine also is a potent stimulus of gastric acid secretion. Other, less prominent effects include formation of edema and stimulation of sensory nerve endings. Bronchoconstriction and contraction of the gut are mediated by H1 receptors. Gastric secretion results from the activation of H2 receptors. Some responses, such as vascular dilation, are mediated by both H1 and H2 receptor stimulation.
H3 AND H4 RECEPTORS. H3 receptors are expressed mainly in the CNS, especially in the basal ganglia, hippocampus, and cortex. H3 receptors function as autoreceptors on histaminergic neurons, inhibiting histamine release and modulating the release of other neurotransmitters. H3 receptors have high constitutive activity and histamine release is tonically inhibited. Inverse agonists thus reduce receptor activation and increase histamine release from histaminergic neurons. H3 agonists promote sleep; thus, H3 antagonists promote wakefulness. H4 receptors primarily are found in eosinophils, dendritic cells, mast cells, monocytes, basophils, and T cells but have also been detected in the GI tract, dermal fibroblasts, CNS, and primary sensory afferent neurons. Activation of H4 receptors has been associated with induction of cellular shape change, chemotaxis, secretion of cytokines, and upregulation of adhesion molecules, suggesting that H4 antagonists may be useful inhibitors of allergic and inflammatory responses.
FEEDBACK REGULATION OF RELEASE. H2 receptor stimulation increases cyclic AMP and leads to feedback inhibition of histamine release from mast cells and basophils, whereas activation of H3 and H4 receptors has the opposite effect by decreasing cellular cyclic AMP. Activation of presynaptic H3 receptors also inhibits histamine release from histaminergic neurons.
CARDIOVASCULAR SYSTEM. Histamine dilates resistance vessels, increases capillary permeability, and lowers systemic blood pressure. In some vascular beds, histamine constricts veins, contributing to the extravasation of fluid and edema formation upstream in capillaries and postcapillary venules.
Vasodilation. This is the most important vascular effect of histamine in humans. H1 receptors have a higher affinity for histamine and cause Ca2+-dependent activation of eNOS in endothelial cells; NO diffuses to vascular smooth muscle, increasing cyclic GMP (see Table 32–1) and causing rapid and short-lived vasodilation. By contrast, activation of H2 receptors on vascular smooth muscle stimulates the cyclic AMP–PKA pathway, causing dilation that develops more slowly and is more sustained. As a result, H1 antagonists effectively counter small dilator responses to low concentrations of histamine but only blunt the initial phase of larger responses to higher concentrations of the amine.
Increased “Capillary” Permeability. Histamine’s effect on small vessels results in efflux of plasma protein and fluid into the extracellular spaces and an increase lymph flow, causing edema. H1 receptors on endothelial cells are the major mediators of this response; the role of H2 receptors is uncertain.
Triple Response of Lewis. If histamine is injected intradermally, it elicits a characteristic phenomenon known as the triple response. This consists of:
• A localized “reddening” around the injection site, appearing within a few seconds, maximal ~1 min
• A “flare” or red flushing extending ~1 cm beyond the original red spot and developing more slowly
• A “wheal” or swelling that is discernible in 1-2 min at the injection site
The initial red spot (a few mm) results from the direct vasodilating effect of histamine (H1 receptor–mediated NO production), the flare is due to histamine-induced stimulation of axon reflexes that cause vasodilation indirectly, and the wheal reflects histamine’s capacity to increase capillary permeability (edema formation).
Heart. Histamine affects both cardiac contractility and electrical events directly. It increases the force of contraction of both atrial and ventricular muscle by promoting the influx of Ca2+, and it speeds heart rate by hastening diastolic depolarization in the sinoatrial (SA) node. It also directly slows atrioventricular (AV) conduction to increase automaticity and, in high doses, can elicit arrhythmias. The slowed AV conduction involves mainly H1 receptors, while the other effects are largely attributable to H2 receptors and cyclic AMP accumulation. The direct cardiac effects of histamine given intravenously are overshadowed by baroreceptor reflexes due to reduced blood pressure.
EXTRAVASCULAR SMOOTH MUSCLE. Histamine directly contracts or, more rarely, relaxes various extravascular smooth muscles. Contraction is due to activation of H1 receptors on smooth muscle to increase intracellular Ca2+, and relaxation is mainly due to activation of H2 receptors. Although the spasmogenic influence of H1 receptors is dominant in human bronchial muscle, H2 receptors with dilator function also are present. Thus, histamine-induced bronchospasm in vitro is potentiated slightly by H2 blockade.
PERIPHERAL NERVE ENDINGS. Histamine stimulates various nerve endings and sensory effects. In the epidermis, it causes itch; in the dermis, it evokes pain, sometimes accompanied by itching.
HISTAMINE SHOCK. Histamine given in large doses or released during systemic anaphylaxis causes a profound and progressive fall in blood pressure. As the small blood vessels dilate, they trap large amounts of blood, their permeability increases, and plasma escapes from the circulation. Resembling surgical or traumatic shock, these effects diminish effective blood volume, reduce venous return, and greatly lower cardiac output.
HISTAMINE TOXICITY FROM INGESTION. Histamine is the toxin in food poisoning from spoiled scombroid fish such as tuna. Symptoms include severe nausea, vomiting, headache, flushing, and sweating. Histamine toxicity also can follow red wine consumption in persons with a diminished ability to degrade histamine. The symptoms of histamine poisoning can be suppressed by H1 antagonists.
H1 RECEPTOR ANTAGONISTS
PHARMACOLOGICAL PROPERTIES
All the available H1 receptor “antagonists” are actually inverse agonists (see Chapter 3) that reduce constitutive activity of the receptor and compete with histamine. At the tissue level, the effect is proportional to receptor occupancy by the antihistamine. Most H1 antagonists have similar pharmacological actions and therapeutic applications. Their effects are largely predictable from knowledge of the consequences of the activation of H1 receptors by histamine.
Like histamine, many H1 antagonists contain a substituted ethylamine moiety.
Unlike histamine, which has a primary amino group and a single aromatic ring, most H1 antagonists have a tertiary amino group linked by a 2- or 3-atom chain to 2 aromatic substituents and conform to the general formula
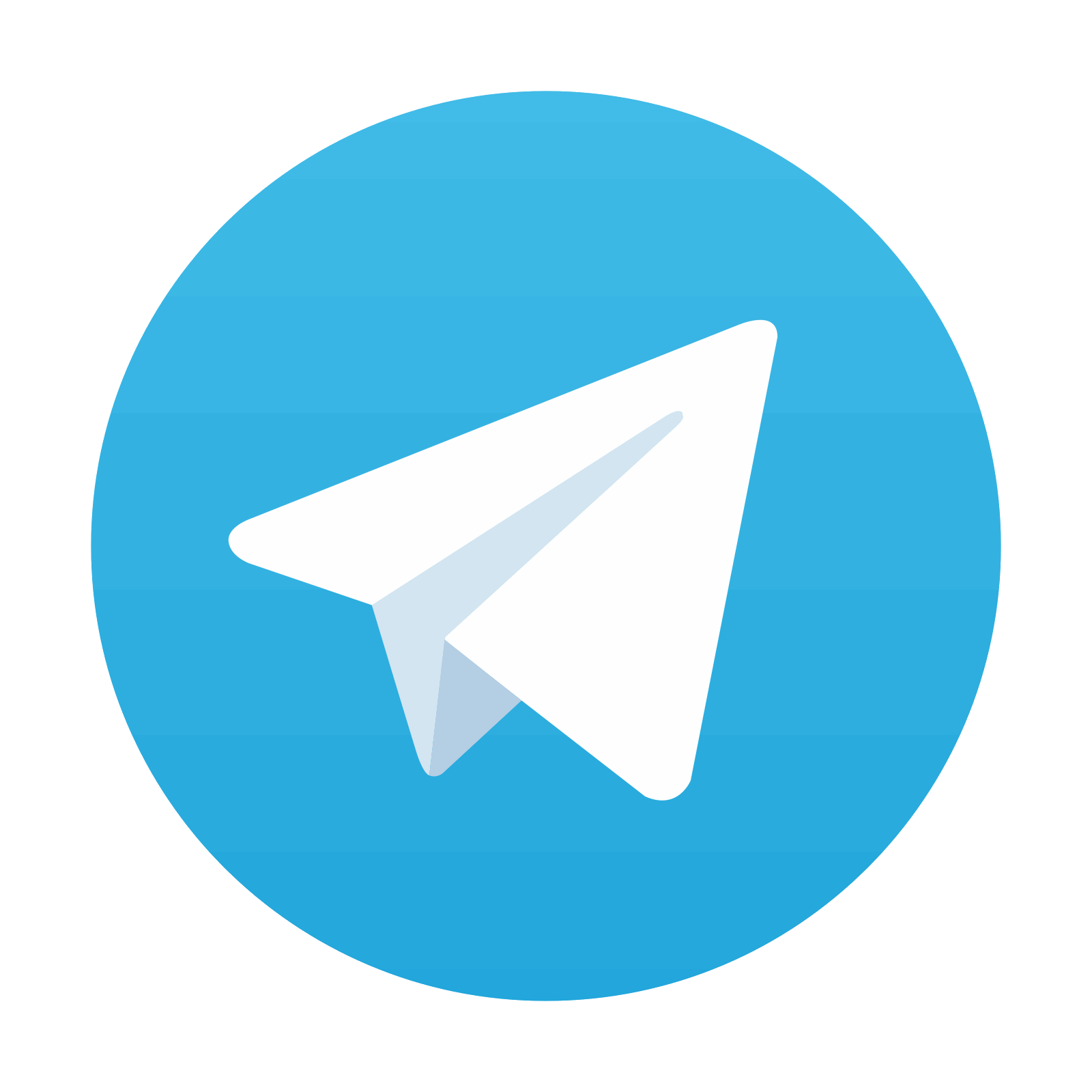
Stay updated, free articles. Join our Telegram channel

Full access? Get Clinical Tree
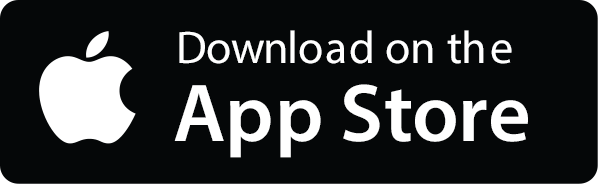
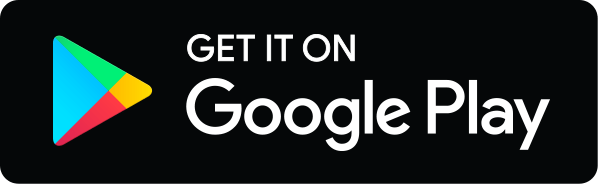