INTRODUCTION
LEARNING OBJECTIVES
After studying this chapter you should know:
The developmental origins of the blood-forming tissues.
The properties and functions that define hematopoietic stem cells.
The role of growth factors and transcription factors in the regulation of hematopoiesis.
The therapeutic roles of growth factors and hematopoietic stem cell transplantation.
In Chapter 1, we introduced the morphologically recognizable marrow progenitors and their progeny, the red cells, white cells, and platelets that make up the formed elements of the blood. Here we turn to the topic of hematopoiesis (from the Greek “to make blood”), the process by which these elements are created. As we will discuss, hematopoiesis depends on rare, morphologically inconspicuous progenitor cells that have remarkable functional properties. Unraveling how hematopoiesis is maintained and regulated has provided a paradigm for understanding the biology of tissue stem cells and has resulted in the development of effective therapies for certain cancers and genetic disorders as well as a variety of conditions in which bone marrow output is inadequate to maintain normal blood cell counts.
DEVELOPMENT OF BLOOD-FORMING TISSUES
The developmental origins of hematopoietic cells are complex and incompletely understood. Cells with the properties of hematopoietic stem cells (HSCs; described in the following section) arise several times in different tissues during prenatal development, producing successive waves of hematopoiesis (Fig. 2-1). Hematopoiesis first appears around day 16 of gestation in the embryonic yolk sac; at this site, it is limited to the production of red cells, which are needed for oxygen transport in the newly developed circulatory system. Hematopoietic cells arise anew around 3 to 4 weeks of gestation in a portion of the ventral mesoderm referred to as the aorta-gonad-mesonephros region. HSCs derived from this region (and possibly the yolk sac as well) are believed to migrate through the blood and take up residence in the liver, which becomes a hematopoietic organ at around 6 weeks of gestation and serves as the major source of hematopoietic cells throughout much of fetal development. HSCs and some hematopoietic progenitors also appear by around 6 weeks of gestation in the placenta and umbilical cord blood and persist in these sites until birth. By 7 to 8 weeks of gestation, lymphoid progenitors derived from the liver begin to seed the newly developed thymus, which is the major site of T-lymphocyte development. Around 5 months of age, HSCs derived from the liver (and possibly other sites) home to the bone marrow, which becomes the dominant source of hematopoietic elements by birth, around the time that hepatic hematopoiesis ceases.
Under normal circumstances, hematopoiesis is confined to the marrow throughout postnatal life, but under stress (e.g., severe inherited anemias) it can persist or reappear in the liver and spread to other extramedullary sites, such as the spleen and the lymph nodes. Hematopoietic neoplasms such as chronic myelogenous leukemia also tend to involve the same organs, sometimes producing massive hepatosplenomegaly and more modest lymphadenopathy.
HEMATOPOIETIC STEM CELLS AND PROGENITORS
Hematopoiesis is a remarkably dynamic process. The marrow normally produces approximately 200 billion red cells, 100 billion platelets, and 60 billion neutrophils each day, numbers that are just sufficient to match the rate of peripheral destruction of these formed elements. Hematopoiesis must persist throughout life and be finely tuned and highly responsive to changes in peripheral blood counts, because both cytopenias (too few formed elements) and cytoses (too many formed elements) can have serious or fatal consequences.
The ability of the hematopoietic system to meet these homeostatic challenges involves a hierarchy of progenitor cells with distinct roles (Fig. 2-2). At the top of this hierarchy lies the HSC. HSCs are essential for the maintenance of hematopoiesis and are by definition multipotent, meaning they can give rise to all other hematopoietic cells. When HSCs divide, under homeostatic conditions, it is thought that at least one of the two daughter cells remains an HSC, a critical property referred to as self-renewal that maintains HSC numbers. Some HSC divisions may be symmetric, such that both daughter cells either become HSCs or begin to differentiate into progenitors (Fig. 2-3). Symmetric divisions that give rise to two HSCs may occur in the fetal liver, a stage of development during which HSC numbers increase. Symmetric divisions in which both daughter cells begin to differentiate into an early hematopoietic progenitor, a process termed commitment, may occur following periods of hematopoietic stress. Other HSC divisions are asymmetric, such that one cell remains an HSC, and the second commits to differentiation. Asymmetric cell divisions are dominant in the bone marrow, in which the number of HSCs remains fairly constant. Details still remain to be worked out, but it appears that the potential of very early committed progenitors is first restricted to myeloid (red cell, granulocyte, and megakaryocyte) or lymphoid (B cell, T cell, and natural killer cell) differentiation. With subsequent divisions, the differentiation potential of progenitors is further refined, so that it is ultimately restricted to a single cell type.
FIGURE 2-2
The hierarchy of hematopoietic cells. Self-renewal is confined to true multipotent hematopoietic stem cells, which lie at the top of the hierarchy, giving rise to all of the cells of the myeloid and erythroid lineage. Immature myeloid cells and both immature and mature lymphoid cells are capable of cell division.
Bone marrow HSCs normally spend most of the time in a resting state referred to as quiescence, only “awakening” to divide, at most, every few months. Quiescence may help to maintain the multipotent state and protect HSCs against the acquisition of mutations that could lead to transformation and cancer development. Under conditions of increased hematopoietic demand, however, HSCs in the marrow divide more frequently and are more likely to divide symmetrically, expanding their numbers. In extreme circumstances, substantial numbers of HSCs and early progenitors may leave the marrow and migrate to the liver, spleen, and lymph nodes, where they can produce extramedullary hematopoiesis.
Given that HSCs have the ability to circulate in the blood, why is postnatal hematopoiesis normally confined to the marrow? One important contributing factor is active homing of HSCs. Circulating HSCs adhere to and migrate across bone marrow capillaries at sites where there are high levels of the chemokine CXCL12. Upon entering the interstitium, the HSCs nestle between blood vessels and osteoblasts (cells that line trabecular bone), which create a specialized microenvironment, or niche. These “niche” cells release factors (such as CXCL12) that regulate HSC behavior in ways that are not yet completely understood. HSCs are resistant to stimulation by hematopoietic growth factors (described in the following section), possibly because niche factors actively promote quiescence. It may be that HSCs expand only when growth factors increase and quiescence factors decrease concomitantly. Another idea posits that HSC numbers are regulated by niche “vacancy,” such that HSCs expand their numbers only when open niches are available. Under conditions of severe hematopoietic stress, it is possible that secondary niches appear in sites of extramedullary hematopoiesis, such as the liver.
Because HSCs are rare cells that are morphologically indistinguishable from lymphocytes, special means must be used to identify them. HSCs express particular surface markers such as CD34 and actively pump out certain dyes, properties that can be used to identify populations of cells that are enriched for HSCs. However, proof that viable HSCs are present in a sample requires functional testing. If a cell preparation can completely reconstitute long-term hematopoiesis when transfused into a host that has had its own marrow cells destroyed (e.g., by high doses of radiation), then it must contain HSCs, which can be quantified by serial dilution of the sample. Although this procedure, termed stem cell transplantation, was originally developed (and is still widely used) for experimental purposes, it was rapidly adopted as a means to treat a variety of diseases (described later in this chapter). It remains the only form of stem cell therapy that is widely used in clinical practice.
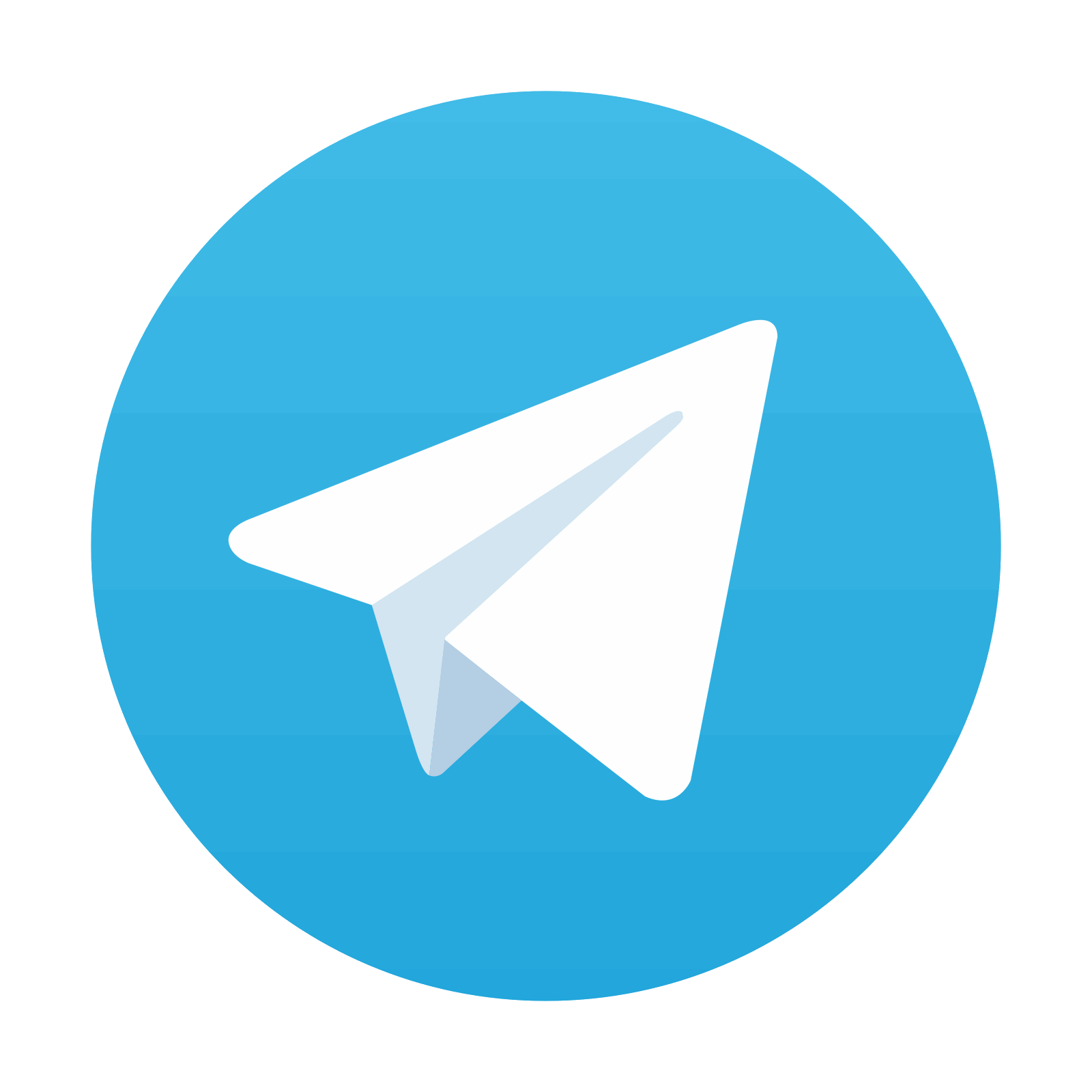
Stay updated, free articles. Join our Telegram channel

Full access? Get Clinical Tree
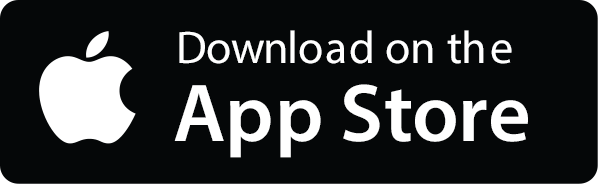
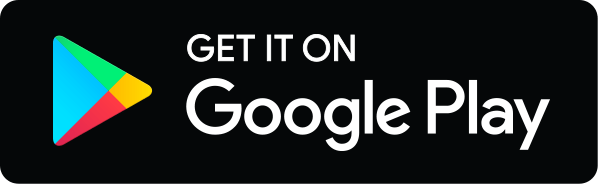