INTRODUCTION
LEARNING OBJECTIVES
After studying this chapter you should be able to:
Describe the structures of the ABO and Rh blood group antigens.
Understand how blood is typed and cross-matched.
Name the clinical and laboratory features of acute and delayed transfusion reactions.
Identify and prioritize the different risks associated with blood transfusion.
In 1492, as Pope Innocent VIII lapsed into a coma, the blood of three boys was infused—into his mouth! Considerable progress has been made since then, particularly following Karl Landsteiner’s discovery of the ABO blood group antigens in 1901. Blood transfusions have had a larger impact on medicine and surgery than any other therapeutic advance in hematology. In the United States, approximately 15 million units of blood are transfused each year.
This chapter begins with the fractionation of donor blood into cellular and plasma components that are used in transfusion. An overview of the important blood group antigens is followed by a consideration of methods used for typing and cross-matching units of blood. After reviewing the indications for transfusing red cells, platelets, and plasma, we discuss the hemolytic, immunologic, inflammatory, and infectious risks posed by transfusion therapy. The chapter ends with a perspective on current and future developments in transfusion medicine.
DONOR BLOOD COMPONENTS
The standard blood donation involves phlebotomy through a large-bore needle inserted into an arm vein. Approximately 450 mL are transferred into a sterile plastic bag containing citrate phosphate dextrose (CPD)-adenine. Citrate (C) prevents coagulation by chelating calcium ions. The phosphate (P) buffer maintains the pH at physiologic levels. Dextrose (D) provides a source of energy during blood storage. Adenine enhances the viability of the stored red cells.
As noted in Chapter 1, when a tube of anticoagulated blood sediments in a gravitational field, the relatively dense red cells go to the bottom, and the less dense white cells and platelets form a “buffy coat” layer on the upper surface of the red cells, whereas cell-free plasma is least dense and collects at the top of the tube. In the blood bank, the bag of freshly collected donor blood is first centrifuged at relatively low speed, allowing separation into packed red cells and platelet-rich plasma (Fig. 25-1). The platelet-rich plasma is then spun at a higher speed, enabling separation into cell-free plasma and a platelet concentrate (Fig. 25-1).
Packed red cells are stored at 4°C for up to 42 days. In most medical centers, the white blood cells are removed by a filter, a maneuver that lowers the incidence of febrile reactions and human leukocyte antigen (HLA) alloimmunization and reduces the risk of infection with cytomegalovirus. Patients undergoing hematopoietic stem cell transplantation receive red cell units that have been irradiated in order to reduce the risk of graft-versus-host disease.
The fresh plasma is frozen and stored at –18°C or colder for up to 1 year. The platelet concentrate is stored at 20°C for a maximum of 5 days. Typically, to prepare a transfusable dose of platelets, concentrates from six donors are pooled. An alternative way to collect platelets in numbers sufficient for transfusion involves circulating venous blood from a donor through an apheresis machine, which continuously removes platelets and returns red cells and plasma to the donor. Platelet units are also irradiated prior to administration to transplant patients.
BLOOD GROUP ANTIGENS
As explained in Chapter 10, red cell stability, pliability, and ion transport depend on specific membrane proteins. In fact, there are over 100 red cell surface membrane proteins. Many of these are polymorphic and have come to attention because they induce clinically significant immune responses when transfused into mismatched recipients. In the past, the blood antigens responsible for these immune responses have been identified by immunologic methods, but in recent years the genes that encode these proteins have been cloned and sequenced, allowing their structure and sometimes function to be established. Figure 25-2 depicts the membrane topology of a number of the well-defined red cell antigens.
A blood group system consists of carbohydrate or protein red cell antigens produced by alleles of a single genetic locus or by closely linked alleles. Most blood group antigens stem from single nucleotide polymorphisms. For example, the S and s alleles of the glycophorin B gene differ by a single nucleotide polymorphism in codon 29, with the S allele encoding a methionine residue (ATG) and the s allele encoding a threonine residue (ACG). Most red cell antigens are proteins expressed on the cell surface, but some, most notably those of the ABO system, stem from differences in carbohydrates linked to surface proteins or glycolipids.
THE ABO SYSTEM
The A and B antigens are defined by the terminal sugar that is attached to glycoproteins and glycosphingolipids by specific transferases. As shown in Figure 25-3, the precursor for these terminal sugars is the H antigen, which has the sequence R-acetylglucosamine-galactose-fucose, where R is a core carbohydrate moiety. The A antigen is formed by a glycosyltransferase that catalyzes the addition of an N-acetylgalactosamine to the subterminal galactose, whereas the B antigen is formed by an allelic variant that catalyzes the addition of another galactose residue to the core galactose. Most populations also contain a common allele lacking enzymatic activity that is designated O. Because OO homozygotes lack A and B terminal transferases, they express only the unmodified H antigen on their red cell surfaces. These individuals have the O blood type. Those who have type A or type B red cells are either homozygotes (AA or BB) or heterozygotes (AO or BO) (Table 25-1). Those with type AB red cells inherit one A allele and one B allele from their two parents.
From fetal development throughout life, individuals are exposed to A and B antigens from a variety of sources and, as a result, develop antigen-specific “natural” IgG and IgM immunoglobulins even in the absence of blood transfusion. The IgM antibodies fix complement and, as explained in more detail, later in this chapter, cause intravascular hemolysis. As shown in Table 25-1, individuals with type A red cells have anti-B antibodies in their plasma, whereas those with type B red cells have anti-A. Individuals with type O red cells have both anti-A and anti-B antibodies in their plasma. They are sometimes called universal donors and, indeed, during the Vietnam War, group O blood was sometimes administered on the battlefield to recipients, irrespective of blood type. Conversely, group AB individuals lack both anti-A and anti-B in their plasma and are sometimes called universal recipients.
THE Rh SYSTEM
The proteins that comprise the Rh system are encoded by two homologous genes on chromosome 1. Both proteins are complex transmembrane proteins that span the plasma membrane of the red cell 12 times. As depicted in Figure 25-4
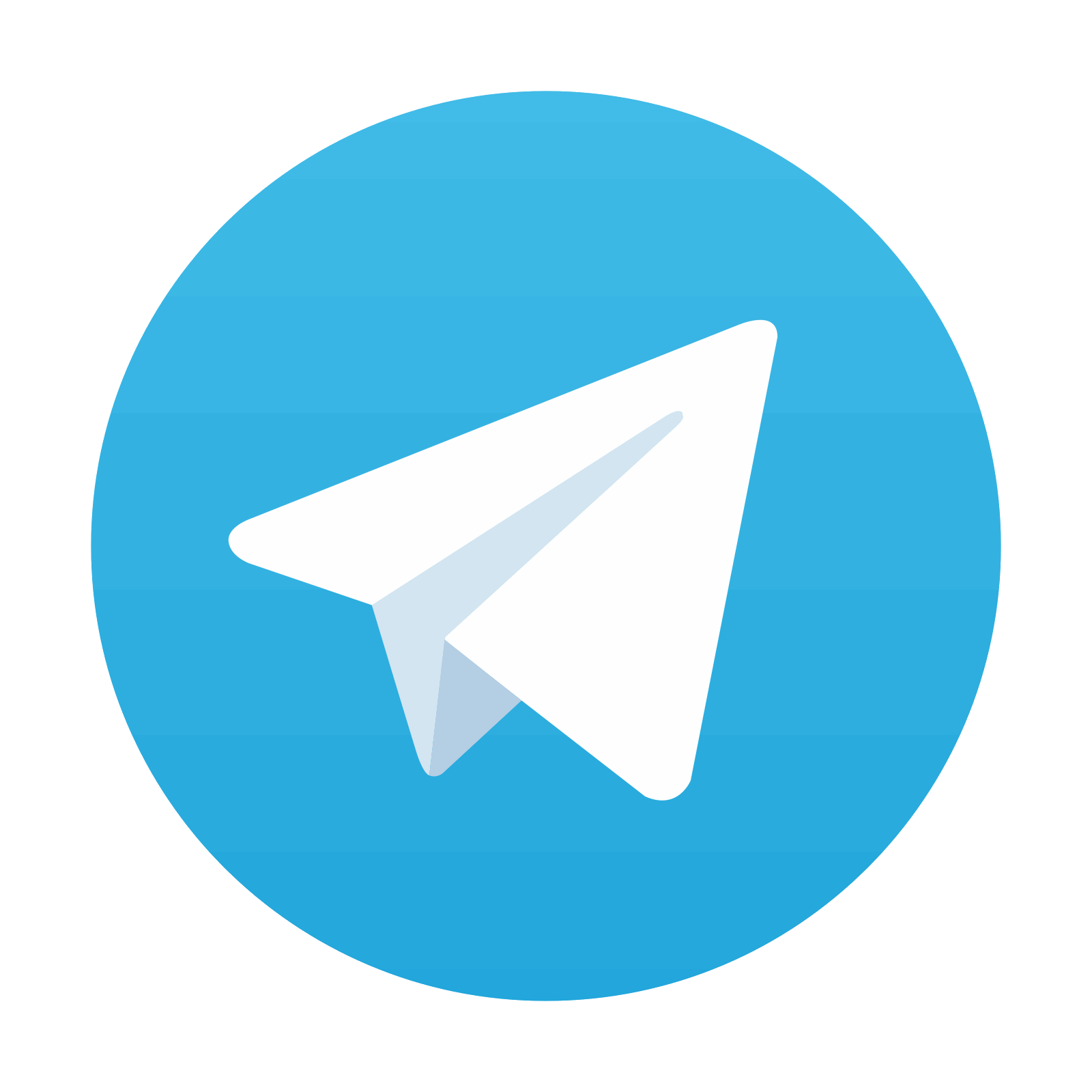
Stay updated, free articles. Join our Telegram channel

Full access? Get Clinical Tree
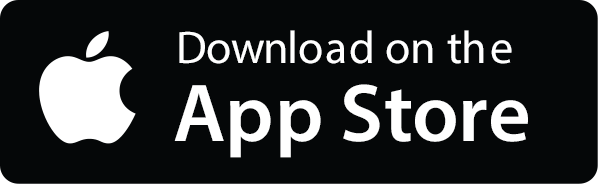
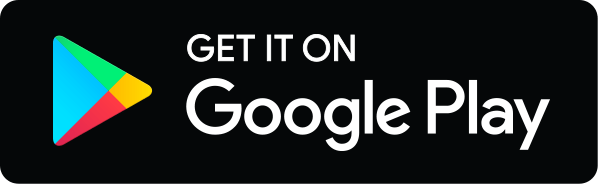
