OBJECTIVES
After studying this chapter, you should be able to:
Describe the components and functions of the external, middle, and inner ear.
Describe the way that movements of molecules in the air are converted into impulses generated in hair cells in the cochlea.
Explain the roles of the tympanic membrane, the auditory ossicles (malleus, incus, and stapes), and scala vestibule in sound transmission.
Explain how auditory impulses travel from the cochlear hair cells to the auditory cortex.
Explain how pitch, loudness, and timbre are coded in the auditory pathways.
Describe the various forms of deafness and the tests used to distinguish between them.
Explain how the receptors in the semicircular canals detect rotational acceleration and how the receptors in the saccule and utricle detect linear acceleration.
List the major sensory inputs that provide the information that is synthesized in the brain into the sense of position in space.
INTRODUCTION
Our ears not only let us detect sounds, but they also help us maintain balance. Receptors for two sensory modalities (hearing and equilibrium) are housed in the ear. The external ear, the middle ear, and the cochlea of the inner ear are involved with hearing. The semicircular canals, the utricle, and the saccule of the inner ear are involved with equilibrium. Both hearing and equilibrium rely on a very specialized type of receptor called a hair cell. There are six groups of hair cells in each inner ear: one in each of the three semicircular canals, one in the utricle, one in the saccule, and one in the cochlea. Receptors in the semicircular canals detect rotational acceleration, those in the utricle detect linear acceleration in the horizontal direction, and the ones in the saccule detect linear acceleration in the vertical direction.
STRUCTURE & FUNCTION OF THE EAR
The external ear funnels sound waves to the external auditory meatus (Figure 10–1). In some animals, the ears can be moved like radar antennas to seek out sound. From the external auditory meatus, sound waves pass inward to the tympanic membrane (eardrum).
FIGURE 10–1
The structures of the external, middle, and inner portions of the human ear. Sound waves travel from the external ear to the tympanic membrane via the external auditory meatus. The middle ear is an air-filled cavity in the temporal bone; it contains the auditory ossicles. The inner ear is composed of the bony and membranous labyrinths. To make the relationships clear, the cochlea has been turned slightly and the middle ear muscles have been omitted. (Reproduced with permission from Fox SI. Human Physiology. New York, NY: McGraw-Hill; 2008.)
The middle ear is an air-filled cavity in the temporal bone that opens via the eustachian (auditory) tube into the nasopharynx and through the nasopharynx to the exterior. The tube is usually closed, but during swallowing, chewing, and yawning it opens, keeping the air pressure on the two sides of the eardrum equalized. The three auditory ossicles, the malleus, incus, and stapes, are located in the middle ear (Figure 10–2). The manubrium (handle of the malleus) is attached to the back of the tympanic membrane. Its head is attached to the wall of the middle ear, and its short process is attached to the incus, which in turn articulates with the head of the stapes. The stapes is named for its resemblance to a stirrup. Its footplate is attached by an annular ligament to the walls of the oval window. Two small skeletal muscles, the tensor tympani and the stapedius, are also located in the middle ear. Contraction of the former pulls the manubrium of the malleus medially and decreases the vibrations of the tympanic membrane; contraction of the latter pulls the footplate of the stapes out of the oval window. The functions of the ossicles and the muscles are considered in more detail below.
FIGURE 10–2
The medial view of the middle ear containing three auditory ossicles (malleus, incus, and stapes) and two small skeletal muscles (tensor tympani muscle and stapedius). The manubrium (handle of the malleus) is attached to the back of the tympanic membrane. Its head is attached to the wall of the middle ear, and its short process is attached to the incus, which in turn articulates with the head of the stapes. The footplate of the stapes is attached by an annular ligament to the walls of the oval window. Contraction of the tensor tympani muscle pulls the manubrium medially and decreases the vibrations of the tympanic membrane; contraction of the stapedius muscle pulls the footplate of the stapes out of the oval window. (Reproduced with permission from Fox SI. Human Physiology. New York, NY: McGraw-Hill; 2008.)
The inner ear (labyrinth) is made up of two parts, one within the other. The bony labyrinth is a series of channels in the petrous portion of the temporal bone and is filled with a fluid called perilymph, which has a relatively low concentration of K+, similar to that of plasma or the cerebrospinal fluid. Inside these bony channels, surrounded by the perilymph, is the membranous labyrinth. The membranous labyrinth more or less duplicates the shape of the bony channels and is filled with a K+-rich fluid called endolymph. The labyrinth has three components: the cochlea that contains hair cells (receptors) for hearing, semicircular canals that contain hair cells that respond to head rotation, and the otolith organs that contain hair cells that respond to changes in gravity and head tilt (Figure 10–3).
FIGURE 10–3
The membranous labyrinth of the inner ear has three components: semicircular canals, cochlea, and otolith organs. The semicircular canals are sensitive to angular accelerations that deflect the gelatinous cupula and associated hair cells. In the cochlea, hair cells spiral along the basilar membrane within the organ of Corti. Airborne sounds set the eardrum in motion, which is conveyed to the cochlea by bones of the middle ear. This flexes the membrane up and down. Hair cells in the organ of Corti are stimulated by shearing motion. The otolithic organs (saccule and utricle) are sensitive to linear acceleration in vertical and horizontal planes. Hair cells are attached to the otolithic membrane. Information from the cochlear hair cells is carried by the cochlear division of the auditory (VIII cranial) nerve. Information from the hair cells in the semicircular canals and otolith organs is carried by the vestibular divisions of the auditory nerve.
The cochlea is a coiled tube that, in humans, is 35 mm long and makes two and three quarter turns. The basilar membrane and Reissner membrane divide it into three chambers or scalae (Figure 10–4). The upper scala vestibuli and the lower scala tympani contain perilymph and communicate with each other at the apex of the cochlea through a small opening called the helicotrema. At the base of the cochlea, the scala vestibuli ends at the oval window, which is closed by the footplate of the stapes. The scala tympani ends at the round window, a foramen on the medial wall of the middle ear that is closed by the flexible secondary tympanic membrane. The scala media, the middle cochlear chamber, is continuous with the membranous labyrinth and does not communicate with the other two scalae.
FIGURE 10–4
Schematic of the cochlea and organ of Corti in the membranous labyrinth of the inner ear. Top: The cross section of the cochlea shows the organ of Corti and the three scalae of the cochlea. Bottom: This shows the structure of the organ of Corti as it appears in the basal turn of the cochlea. DC, outer phalangeal cells (Deiters cells) supporting outer hair cells; IPC, inner phalangeal cells supporting inner hair cell. (Reproduced with permission from Pickels JO: An Introduction to the Physiology of Hearing, 2nd ed. Academic Press; 1988.)
The organ of Corti on the basilar membrane extends from the apex to the base of the cochlea and thus has a spiral shape. This structure contains the highly specialized auditory receptors (hair cells) whose processes pierce the tough, membrane-like reticular lamina that is supported by the pillar cells or rods of Corti (Figure 10–4). The hair cells are arranged in four rows: three rows of outer hair cells lateral to the tunnel formed by the rods of Corti, and one row of inner hair cells medial to the tunnel. There are 20,000 outer hair cells and 3500 inner hair cells in each human cochlea. Covering the rows of hair cells is a thin, viscous, but elastic tectorial membrane in which the tips of the hairs of the outer but not the inner hair cells are embedded. The cell bodies of the sensory neurons that arborize around the bases of the hair cells are located in the spiral ganglion within the modiolus, the bony core around which the cochlea is wound. Ninety to 95% of these sensory neurons innervate the inner hair cells; only 5–10% innervate the more numerous outer hair cells, and each sensory neuron innervates several outer hair cells. By contrast, most of the efferent fibers in the auditory nerve terminate on the outer rather than inner hair cells. The axons of the afferent neurons that innervate the hair cells form the auditory (cochlear) division of the eighth cranial nerve.
In the cochlea, tight junctions between the hair cells and the adjacent phalangeal cells prevent endolymph from reaching the bases of the cells. However, the basilar membrane is relatively permeable to perilymph in the scala tympani, and consequently, the tunnel of the organ of Corti and the bases of the hair cells are bathed in perilymph. Because of similar tight junctions, the arrangement is similar for the hair cells in other parts of the inner ear; that is, the processes of the hair cells are bathed in endolymph, whereas their bases are bathed in perilymph.
On each side of the head, the semicircular canals are perpendicular to each other, so that they are oriented in the three planes of space. A receptor structure, the crista ampullaris, is located in the expanded end (ampulla) of each of the membranous canals (Figure 10–3). Each crista consists of hair cells and supporting (sustentacular) cells surmounted by a gelatinous partition (cupula) that closes off the ampulla. The processes of the hair cells are embedded in the cupula, and the bases of the hair cells are in close contact with the afferent fibers of the vestibular division of the eighth cranial nerve.
A pair of otolith organs, the saccule and utricle, are located near the center of the membranous labyrinth. The sensory epithelium of these organs is called the macula. The maculae are vertically oriented in the saccule and horizontally located in the utricle when the head is upright. The maculae contain supporting cells and hair cells, surrounded by an otolithic membrane in which are embedded crystals of calcium carbonate, the otoliths (Figure 10–3). The otoliths, which are also called otoconia or ear dust, range from 3 to 19 μm in length in humans. The processes of the hair cells are embedded in the membrane. The nerve fibers from the hair cells join those from the cristae in the vestibular division of the eighth cranial nerve.
The specialized sensory receptors in the ear consist of six patches of hair cells in the membranous labyrinth. These are examples of mechanoreceptors. The hair cells in the organ of Corti signal hearing; the hair cells in the utricle signal horizontal acceleration; the hair cells in the saccule signal vertical acceleration; and a patch in each of the three semicircular canals signal rotational acceleration. These hair cells have a common structure (Figure 10–5). Each is embedded in an epithelium made up of supporting cells, with the basal end in close contact with afferent neurons. Projecting from the apical end are 30–150 rod-shaped processes, or hairs. Except in the cochlea, one of these, the kinocilium, is a true but nonmotile cilium with nine pairs of microtubules around its circumference and a central pair of microtubules. It is one of the largest processes and has a clubbed end. The kinocilium is lost from the hair cells of the cochlea in adult mammals. However, the other processes, which are called stereocilia, are present in all hair cells. They have cores composed of parallel filaments of actin. The actin is coated with various isoforms of myosin. Within the clump of processes on each cell there is an orderly structure. Along an axis toward the kinocilium, the stereocilia increase progressively in height; along the perpendicular axis, all the stereocilia are of the same height.
FIGURE 10–5
Structure of hair cell in the saccule. Left: Hair cells in the membranous labyrinth of the ear have a common structure, and each is within an epithelium of supporting cells (SC) surmounted by an otolithic membrane (OM) embedded with crystals of calcium carbonate, the otoliths (OL). Projecting from the apical end are rod-shaped processes, or hair cells (RC), in contact with afferent (A) and efferent (E) nerve fibers. Except in the cochlea, one of these, kinocilium (K), is a true but nonmotile cilium with nine pairs of microtubules around its circumference and a central pair of microtubules. The other processes, stereocilia (S), are found in all hair cells; they have cores of actin filaments coated with isoforms of myosin. Within the clump of processes on each cell there is an orderly structure. Along an axis toward the kinocilium, the stereocilia increase progressively in height; along the perpendicular axis, all the stereocilia are the same height. (Reproduced with permission from Llinas R, Precht W (eds): Frog Neurobiology. Springer; 1976.) Right: Scanning electron micrograph of processes on a hair cell in the saccule. The otolithic membrane has been removed. The small projections around the hair cell are microvilli on supporting cells. (Used with permission of AJ Hudspeth.)
Very fine processes called tip links (Figure 10–6) tie the tip of each stereocilium to the side of its higher neighbor, and at the junction are mechanically sensitive cation channels in the taller process. When the shorter stereocilia are pushed toward the taller ones, the open time of these channels is increased. K+, the most abundant cation in endolymph, and Ca2+ enter via the channel and produce depolarization. A myosin-based molecular motor in the taller neighbor then moves the channel toward the base, releasing tension in the tip link (Figure 10–6). This causes the channel to close and permits restoration of the resting state. Depolarization of hair cells causes them to release a neurotransmitter, probably glutamate, which initiates depolarization of neighboring afferent neurons.
FIGURE 10–6
Schematic representation of the role of tip links in the responses of hair cells. When a stereocilium is pushed toward a taller stereocilium, the tip link is stretched and opens an ion channel in its taller neighbor. The channel next is presumably moved down the taller stereocilium by a molecular motor, so the tension on the tip link is released. When the hairs return to the resting position, the motor moves back up the stereocilium.
The K+ that enters hair cells via the mechanically sensitive cation channels is recycled (Figure 10–7). It enters supporting cells and then passes on to other supporting cells by way of tight junctions. In the cochlea, it eventually reaches the stria vascularis and is secreted back into the endolymph, completing the cycle.
FIGURE 10–7
Ionic composition of perilymph in the scala vestibuli, endolymph in the scala media, and perilymph in the scala tympani. SL, spiral ligament. SV, stria vascularis. The dashed arrow indicates the path by which K+ recycles from the hair cells to the supporting cells to the spiral ligament and is then secreted back into the endolymph by cells in the stria vascularis.
As described above, the processes of the hair cells project into the endolymph whereas the bases are bathed in perilymph. This arrangement is necessary for the normal production of receptor potentials. The perilymph is formed mainly from plasma. On the other hand, endolymph is formed in the scala media by the stria vascularis and has a high concentration of K+ and a low concentration of Na+ (Figure 10–7). Cells in the stria vascularis have a high concentration of Na+, K+ ATPase. In addition, it appears that a unique electrogenic K+ pump in the stria vascularis accounts for the fact that the scala media is electrically positive by 85 mV relative to the scala vestibuli and scala tympani.
The resting membrane potential of the hair cells is about –60 mV. When the stereocilia are pushed toward the kinocilium, the membrane potential is decreased to about –50 mV. When the bundle of processes is pushed in the opposite direction, the cell is hyperpolarized. Displacing the processes in a direction perpendicular to this axis provides no change in membrane potential, and displacing the processes in directions that are intermediate between these two directions produces depolarization or hyperpolarization that is proportional to the degree to which the direction is toward or away from the kinocilium. Thus, the hair processes provide a mechanism for generating changes in membrane potential proportional to the direction and distance the hair moves.
HEARING
Sound is the sensation produced when longitudinal vibrations of the molecules in the external environment—that is, alternate phases of condensation and rarefaction of the molecules—strike the tympanic membrane. A plot of these movements as changes in pressure on the tympanic membrane per unit of time is a series of waves (Figure 10–8); such movements in the environment are generally called sound waves. The waves travel through air at a speed of approximately 344 m/s (770 mph) at 20°C at sea level. The speed of sound increases with temperature and with altitude. Other media can also conduct sound waves, but at a different speed. For example, the speed of sound is 1450 m/s at 20°C in fresh water and is even greater in salt water. It is said that the whistle of the blue whale is as loud as 188 dB and is audible for 500 miles.
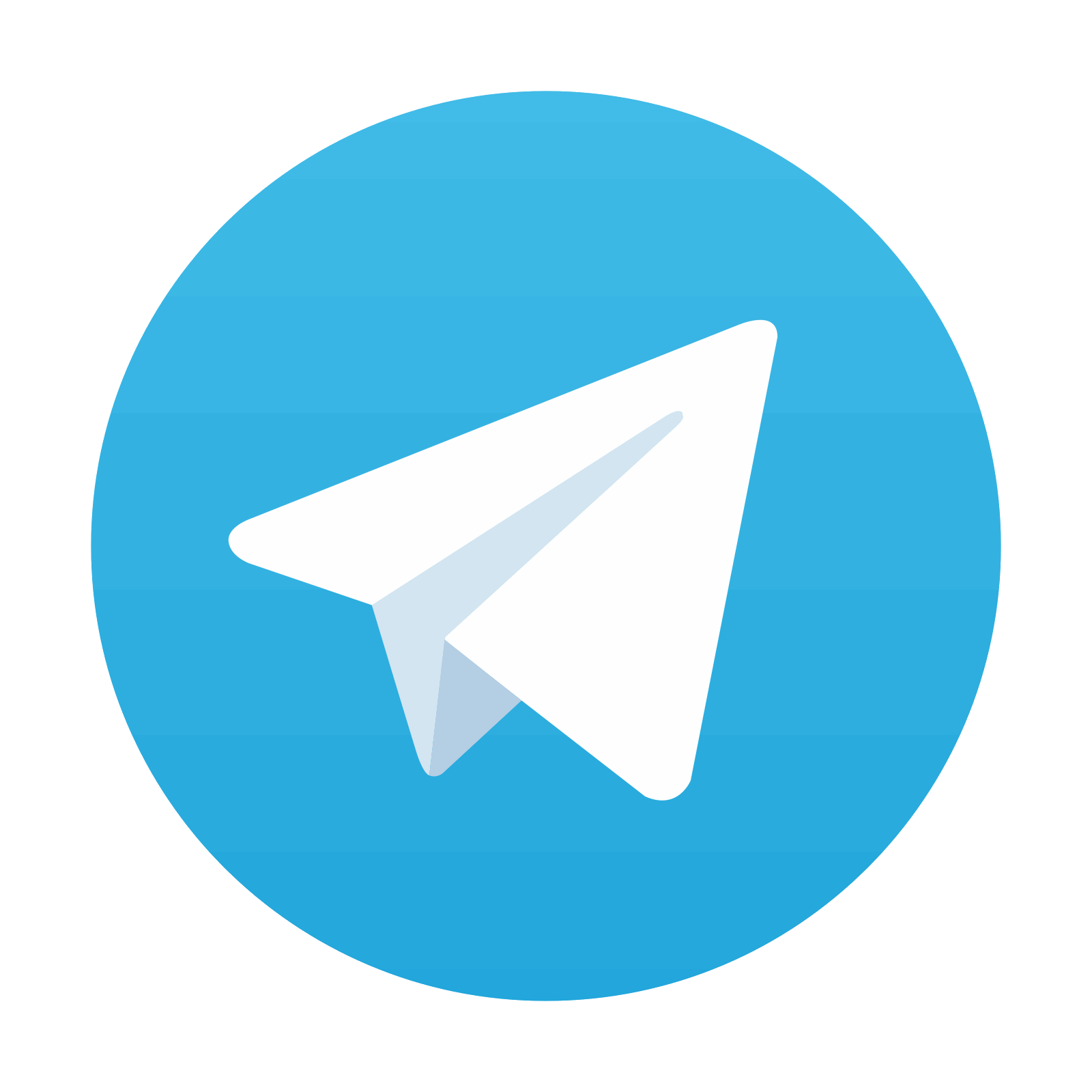
Stay updated, free articles. Join our Telegram channel

Full access? Get Clinical Tree
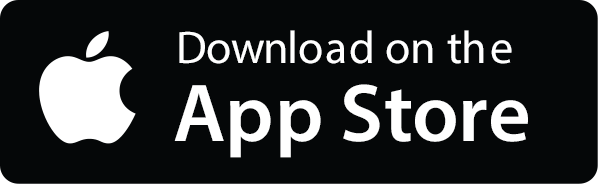
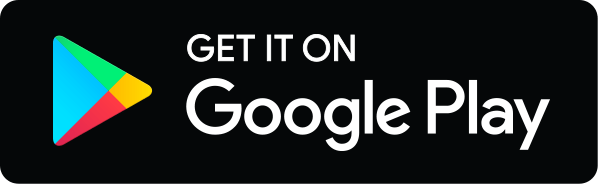