Figure 21-1. Cerebral pressure autoregulation. The normal relationship is indicated by the solid line with autoregulatory breakpoints at 50 and 150 mm Hg. Two disrupted states are also diagrammed. Complete loss of autoregulation (straight dashed line B) results in a pressure-passive system wherein cerebral blood flow (CBF) (and cerebral blood volume [CBV]) increases linearly with cerebral profusion pressure (CPP). The more common form of disruption is indicated by the sigmoid (dashed line A) where the major alteration is a right shift in the lower breakpoint. The circles at the top of the figure represent the diameters of the resistance vessels in the normal situation. The area of the circles represents CBV. Shifting this relationship to the right by 30 mm Hg would represent the partially disrupted state. (© R.M. Chesnut, MD, reproduced with permission.)
CLINICAL ASSESSMENT
The objectives during early clinical assessment of the patient with head injury are multiple and must be accomplished simultaneously. These include establishing adequate oxygenation, ventilation, and circulatory stability and evaluating the extent of brain injury while treating ICP elevations. Although some evidence indicates that systemic hypotension may infrequently be the result of a head injury, always initially presume that hypotension in a trauma patient is the result of hypovolemia. It is a significant error to withhold volume resuscitation in a misdirected effort to control cerebral edema.
During initial assessment, mental status changes cannot be presumed to be the result of drugs or alcohol, although routine toxicology screening is appropriate. It should be presumed that any change in mental status or the neurologic examination in general, or any evidence of herniation (e.g., anisocoria), suggests an expanding intracranial mass lesion. Under such circumstances, therapeutic ICP reduction becomes the first priority and diagnostic imaging or surgical decompression must be accomplished emergently.
Do not assume that apparent neurologic unresponsiveness represents a lack of sensitivity to pain. Noxious stimuli, such as placement of urinary drainage catheters, nasogastric tubes, or IV catheters, can precipitate ICP peaks during resuscitation. These procedures should be done quickly and efficiently, optimally after sedation. Endotracheal intubation is particularly likely to induce herniation in borderline cases. Whenever practical, consider premedication with analgesics, sedatives, or IV or endotracheal lidocaine before airway instrumentation.
With regard to the brain injury, several critical assessments are necessary and should be precisely recorded because trends are at least as important as any single observation. The three key parameters are level of consciousness, pupillary reflexes and size, and the motor examination.
Glasgow Coma Scale
The single most important assessment for a patient with head injury is to evaluate the level of consciousness. In this regard, the Glasgow Coma Scale (GCS) has become an international standard that is easily, rapidly, and reliably obtained (Algorithm 21-1).21 Components of the GCS include assessment of eye opening (E), verbal response (V), and motor response (M). The Guidelines for the Prehospital Care of Severe Brain Injury support the use of the GCS for all prehospital care providers.22 Special training has been developed by the Brain Trauma Foundation to optimize its accuracy (http://www.braintrauma.org/).
For individual patients, it is recommended that all three components be reported separately (e.g., E4V5M6) instead of a sum score (e.g., GCS = 15).23 The derived sum score is more relevant for comparisons at the group level, and for classification and prognosis. For triage purposes, patients can be stratified using their GCS scores into those with severe injuries (GCS ≤8), moderate injuries (GCS 9 to 12), or mild injuries (GCS ≥13; see Algorithm 21-1).
Assessment requires either a spontaneous response or response following application of a stimulus. At more severely disturbed levels of consciousness, the motor score has better discrimination, but in milder injuries the eye and verbal components are more relevant. Thus each component of the scale provides complementary information. Strengths of the GCS are that it covers a broad spectrum of disorders of consciousness, is widely applicable, and offers an important tool for monitoring changes in the level of consciousness. Standardized approaches to both its assessment and its reporting are required in order to be able to compare evaluations over time or when communicating with other healthcare professionals. Spontaneous responses are first observed without stimulating the patient in any way. First verbal stimuli are applied, such as asking a patient to obey commands and at the same time observing whether, for example, an eye opening occurs. If a patient is not responsive, a stimulus is applied to elicit a response. The location of the stimulus (central or peripheral) should be standardized and used consistently. To describe the motor response, only the reaction of the arms should be observed, not the legs.24
Algorithm 21-1. Glasgow Coma Scale (GCS) triage guide for initial evaluation of head injury. For the motor scale, the best response for any limb is recorded.
If a GCS component is untestable due to intubation, sedation, or other confounder, the reason for this should be recorded (e.g., E1VTM2, with “T” indicating “intubated” and not a testable verbal component). A score of “1” should not be assigned because differentiation between a “true 1” and an untestable component is relevant.
Pupils
Pupillary asymmetry, dilation, or loss of light reflex in an unconscious patient usually reflects herniation because of the mass effect from intracranial hemorrhage ipsilateral to the dilated pupil. The probability of an intracranial mass lesion can be roughly approximated given the degree of anisocoria (1 mm or 3 mm), the mechanism of injury (MVC), and age (Fig. 21-2).21 Occasionally, pupillary signs may indicate direct second or third nerve injury or trauma to the globe, but this must always be a diagnosis of exclusion. An unequal and nonreactive pupil is the cardinal sign that herniation is occurring, and rapid lowering of ICP is essential. An ovoid pupil is also ominous and is associated with injuries that result in herniation in approximately 15% to 20% of patients.
Figure 21-2. Estimated percentage chance of an extra-axial intracranial mass lesion greater than 25 mL as a function of degree of anisocoria, age, and mechanism of injury. Mechanism of injury was defined as motor vehicle accident (MVA) or other mechanism (not MVA). (Reproduced with permission from Chesnut RM, Gautille T, Blunt BA, et al. The localizing value of asymmetry in pupillary size in severe head injury: relation to lesion type and location. Neurosurgery 1994;34:840–845.)
Recently, handheld pupillometry has gained acceptance as a method for quantifying pupillary response. Traditionally, the measurement of pupil function has been subjective, using a flashlight and pupil gauge with a qualitative assessment of reactivity, often resulting in poor interrater reliability.25 The currently available devices measure the rate and degree of pupillary contraction and calculates a normalized neurologic pupillary index, or NPi.26,27 NPi >4 is indicative of normal function, while NPi <4 raises suspicion for pupil contractility dysfunction. It should be noted that, although one etiology of decreased NPi is increased ICP, other causes such as direct cranial nerve III dysfunction or diabetic pupillary paresis must also be considered.
Motor Examination
The motor system is examined for asymmetry, abnormal posturing, or lack of movement. Hemiparesis, paraparesis, or quadriparesis suggests a cervical or thoracolumbar spine fracture with spinal cord injury. Hemiparesis secondary to brainstem herniation from the mass effect may be either ipsilateral or contralateral to the side of the dilated pupil or an intracranial mass lesion.21 Hemiparesis may also result from significant brain contusion. In the unconscious patient, a painful stimulus should be used to evaluate motor function. All four extremities should be examined and the results noted, because only the response of the best limb will be reflected in the GCS score.
RADIOGRAPHIC DIAGNOSIS
Neurosurgical evaluation and assessment are initiated as soon as the potential for significant head injury is realized. Prompt radiographic evaluation is essential and CT scanning is the imaging modality of choice for virtually all acute neurologic conditions. Patients with mild head injuries can usually be observed with sequential examinations and radiographic evaluation may be unnecessary unless the results determine whether the patient can be discharged from the hospital. In contrast, however, a cogent argument can be made for the liberal application of CT scanning to even patients with minimal evidence of TBI as a method of making safe, efficient, and economic triage decisions.30 In any instance, evidence of neurologic deterioration or the occurrence of a situation wherein the neurologic examination cannot be followed (e.g., the need for general anesthesia) mandates CT scanning, intraoperative ICP monitoring, or both. General indications for neurologic imaging (generally, CT scanning) are listed in Table 21-1.
Patients with moderate or severe injuries require prompt neurosurgical consultation and rapid radiographic evaluation using the CT scanner. Hemodynamically stable patients with significant neurologic deterioration should go to the CT scanner immediately following ATLS resuscitation. In hemodynamically unstable patients who require immediate surgical intervention to sustain intravascular volume, lifesaving exploratory thoracotomy or laparotomy must take precedence. In such cases, it is a mistake to delay further investigation of the intracranial compartment pending the end of the case and transport to the CT scanner. A number of methods can be employed to evaluate the intracranial compartment during such lifesaving, extracranial surgery, including insertion of an ICP monitor or ventriculostomy (with air ventriculography, if deemed necessary), transcranial Doppler evaluation, or even placement of exploratory burr holes in the instance of herniation. For this reason, neurosurgical consultation should be initiated on arrival in theater rather than at the finish of the case.
Table 21-1 Indications For Neurologic Imaging
At the other end of the spectrum, in cases where there is an obvious surgical brain injury, the choice and timing of diagnostic systemic maneuvers should be subject to modification. Such patients should be transported directly to theater and examinations such as focused assessment with sonography in trauma (FAST) or diagnostic peritoneal lavage performed as the patient is being prepped for craniotomy. In such instances, obviously, early communication between the trauma surgery and neurosurgery departments is critical.
The spine should be cleared radiographically or immobilized and protected in every patient with a severe head injury. Although only 13% of patients with severe head injuries have spinal cord injuries, the potentially devastating consequences of an overlooked spine injury require constant vigilance.
Plain Skull Radiographs
With the routine availability of 24-hour CT scanning capabilities, plain skull radiography has largely become obsolete in TBI. The likelihood of a surgical intracranial hematoma is strongly correlated with the presence or absence of a skull fracture if the neurologic examination is factored in.31 Nevertheless, the superiority of CT imaging in all aspects of evaluating TBI has relegated the use of skull films to unusual circumstances.
Computed Tomography
The CT finding that correlates most highly with intracranial hypertension is compression or obliteration of the basilar cisterns (Fig. 21-3). Not only does this finding portend a stormy ICP course, but also the primary predictor of outcome in patients with this CT picture is the peak level of intracranial hypertension occurring during the first 72 hours.32,33 When cisternal compression is paired with a midline shift of more than 5 mm, the prognosis is even more ominous. ICP monitoring should be immediately initiated in any patient with cisternal compression and intracranial hypertension should be vigorously treated. Such patients, particularly those with minimal evidence of contusions, die primarily from secondary brain insults, which implies that they are potentially salvageable.
Figure 21-3. A computed tomography scan that is highly predictive of intracranial hypertension. The basilar cisterns are obliterated and the sulci are flattened.
Acute epidural hematomas correlate well with skull fractures. The most common association is a linear, nondisplaced fracture in the temporoparietal region, crossing the middle meningeal artery. The classic clinical course involving a lucid interval following a brief loss of consciousness with subsequent suspicion must remain high. The typical CT appearance is a high- or mixed-density concave extra-axial hematoma with smooth borders (Fig. 21-4).
Figure 21-4. Typical computed tomography scan appearance of mixed-density, lens-shaped, acute epidural hematoma with mass effect.
Acute subdural hematomas occur over the convexity of the brain. The hematoma may evolve from rupture of bridging cortical veins or bleeding from the underlying parenchymal injury, the latter being a common source. It is this subjacent tissue damage that generally determines the neurologic outcome of patients not succumbing to intracranial hypertension. On CT scan, a subdural hematoma appears as an extra-axial high- or mixed-density crescentic mass that spreads out over the hemisphere, following the cortical irregularities (Fig. 21-5). The midline shift may be out of proportion to the size of the hematoma because of the contributing mass effect from an underlying brain contusion or hemispheric swelling.
Intracerebral hemorrhage and cerebral contusion are common after trauma and are readily visualized with CT scanning. Brain contusion appears as a focal, heterogeneous density with hemorrhage interspersed with injured tissue (Fig. 21-6). Intracerebral hematomas are generally more homogeneous in their high-density appearance. Both of these lesions tend to “blossom” over time because of some continued hemorrhage and the development of edema. It is important, therefore, to closely observe and monitor the ICP of such patients because significant and hazardous mass effect may evolve, requiring surgical extirpation.
With temporal or deep frontal contusions, late deterioration may occur, generally because of progressive edema development. The peak of such deterioration appears to be around 1 week, although cases have occurred as late as 10 to 14 days. Most of these patients will complain of severe or increasing headache or their CT images will show progressive edema formation. As such, a high index of suspicion must be accompanied by liberal use of follow-up CT imaging and, in particular, not dropping the level of surveillance until both the clinical situation and the CT appearance are stable. In the instance of such lesions showing progressive mass effect, the insertion of an ICP monitor may be considered, even in a patient with a relatively high GCS score.
Figure 21-5. Usual computed tomography scan appearance of a crescent-shaped, high-density blood collection conforming to the contour of the cerebral hemisphere in a subdural hematoma.
Figure 21-6. Contusion and associated intracerebral hematoma in the frontotemporal area.
The typical CT appearance of subarachnoid hemorrhage is a layer of blood over the cerebral cortex, layering over the tentorium and commonly filling the basal cisterns. Cerebral edema appears as areas of decreased density, which may be either focal or diffuse. Posttraumatic edema formation generally takes hours to days to develop unless compounded by hypoxia or hypotension. The “swollen brain” commonly seen in the setting of trauma may be caused by edema or increased CBV (unclotted, intravascular blood is low density). DAI, typical of acute acceleration–deceleration injury, appears on CT scan as small areas of focal hemorrhage in the brainstem, thalamus or deep nuclear region, corpus callosum, and hemispheric white matter and may be accompanied by cerebral swelling. Finally, gunshot wounds or other penetrating injuries can be evaluated with CT scanning to allow accurate preoperative assessment of the anatomic injury for prognostic and therapeutic planning purposes.
One significant issue of recent origin is the “blossoming” or appearance of new lesions subsequent to CT images obtained at very short intervals following injury. Most of the “classic” TBI studies presented initial CT data from studies done hours following trauma. With improved prehospital transport and the ready availability of CT imaging, many initial studies are now performed 15 to 30 minutes after injury. As a result of such ultra-early CT scanning, there is now a risk of missing significant intracranial lesions by obtaining imaging before their appearance or during an early phase of their evolution. For this reason, in any patient with moderate or severe TBI, intracranial hypertension, or anticoagulation/antiplatelets, we routinely obtain an early follow-up CT image at 4 to 6 hours after admission. In patients with mild TBI who have a stable neurologic examination and none of the aforementioned risk factors, routine repeat head CT rarely changes management and may be skipped.28–31
It should be noted that absence of CT findings in a patient with a history of TBI does rule out a TBI. Recent data suggest that a significant number of patients seen at a level I trauma center with a negative CT will have pathologic findings with 3T MRI imaging.32 Advanced MRI techniques, such as diffusion tensor imaging and functional MRI hold great promise for improved diagnosis and prognosis, especially in mild TBI/concussion.33
Ancillary Imaging
As noted earlier, there is a significant association between TBI and injury to the axial skeleton, particularly to the cervical spine. In addition, particularly when associated with basilar skull fractures or facial fractures, TBI suggests the possibility of damage to the major arteries of the neck. As such, particular attention should be given to careful radiographic clearance of the spine and consideration of studies such as CT angiography of the great vessels rostral to the aortic arch.
MANAGEMENT
Evidence-based Medicine and the Management of Traumatic Brain Injury
Wherever possible, the recommendations in this text are evidence based. For details, the reader is referred to the source documents.
The publication of the first edition of the Guidelines for the Management of Severe Brain Injury in 1996 represented a significant step in standardizing the management of TBI based on published, peer-reviewed literature.22 This document represents the application of a strict evidence-based process to 14 topics relevant to TBI care. Following an exhaustive, explicitly defined literature search covering each topic, the recovered literature was carefully classified along a three-point continuum of scientific rigor. As such, each article was ranked as class I, class II, or class III and the analysis of the scientific basis of each topic was predicated on the most scientifically rigorous (highest literature class) reports available. This process produced a set of standards, guidelines, and options for treatment where standards (based on class I evidence) represent principles with a high degree of clinical certainty, guidelines (based on class II evidence) reflect principles with a moderate degree of clinical certainty, and options (based on class III evidence) reflect principles for which unclear clinical certainty exists. By specifically defining the scientific foundation on TBI management issues, the Guidelines for the Management of Severe Brain Injury provided an unbiased reference focused on facilitating scientific management of TBI.
Since the initial publication of the Guidelines for the Management of Severe Brain Injury, it has undergone updating and revision10,11 encompassing more recently published data and revising earlier practice recommendations where indicated as well as expanding the scope of covered topics. The same evidence-based process has also been applied in the generation of other sets of brain injury guidelines. The Guidelines for the Prehospital Management of Traumatic Brain Injury were published in 2002,20 spawning the training efforts mentioned previously. In 2001, the Guidelines for the Management of Penetrating Brain Injury (PBI) were published.23 The Guidelines for the Management of Pediatric Brain Injury were published in 2003.18 The Guidelines for the Surgical Management of Traumatic Brain Injury were published in 2001.24 The third edition of the Guidelines were published in 200734 and work on the fourth edition is now underway.
General Considerations
Although there is no present technology for its quantification before the insertion of an ICP monitoring device, early intracranial hypertension may certainly exert a detrimental influence on outcome. Not only do all treatment modalities for intracranial hypertension have serious potential complications, but also many of them can directly interfere with resuscitation procedures (e.g., use of osmotic diuretics). The efficacy of successful systemic resuscitation in improving the likelihood of survival from trauma in general is well accepted. In addition, the acknowledged negative influence of secondary insults (e.g., hypotension and hypoxia) on outcome from severe head injury renders systemic resuscitation essential. Therefore, all treatment must be consistent with optimal systemic resuscitation.
The composition and volume of the IV fluids used to resuscitate patients with head injuries should be selected with the purpose of restoring intravascular blood volume. Although the widely disseminated but scientifically unsupported adage of “keeping TBI patients dry” has now been discarded, the concept of restricting free water remains desirable. As such, isotonic crystalloid solution in the form of 0.9% normal saline (NS) is preferable to lactated Ringer solution as a resuscitation fluid for TBI.35 For some time, a growing body of indirect scientific support appeared to support the use of 250 mL of 7.5% hypertonic saline as the first resuscitation fluid in TBI victims. However, a recent randomized controlled trial from Australia has suggested that this may not be of benefit under optimal resuscitation conditions.28
The endpoints of resuscitation do not change depending on the presence or absence of a head injury. Blood volume should be normal, with an appropriate blood pressure and central venous pressure, adequate urine output and peripheral perfusion, and progressive improvement of any base deficit. The systolic blood pressure should never be allowed to drop below 90 mm Hg. Some evidence indicates an advantage to targeting a mean arterial pressure of 80 to 90 mm Hg during resuscitation until ICP monitoring can be initiated. Once ICP is available, a minimal CPP of 60 mm Hg should initially be the goal.
Initial Resuscitation
Resuscitation in the Absence of Clinical Signs of Herniation
2, 3 Algorithm 21-2 is based on the Guidelines for the Management of Severe Brain Injury and the Guidelines for the Prehospital Management of Severe Brain Injury for use by prehospital and initial at-hospital care providers and emergency physicians to guide decision making in resuscitating TBI victims and determining the necessity of ICP-lowering therapy.22,34 “Signs of increased ICP” implies pupillary abnormalities, motor posturing, or neurologic deterioration not related to medications. When these signs are not present, mannitol is not given and the goal of ventilation is eucapnia (i.e., no hyperventilation). When signs of herniation are present, the patient is hyperventilated to a PaCO2 of 30 to 35 mm Hg and mannitol may be given if the patient’s volume status is normal.
Algorithm 21-3 is based on the Guidelines for the Management of Severe Brain Injury for evaluation and treatment of the severe TBI patient from arrival at the trauma center prior to the placement of an ICP monitor.34 As with any trauma patient, the first step is Advanced Trauma Life Support (ATLS) resuscitation. When appropriate, brain-specific therapies are incorporated into the treatment course. Brain-friendly initial ICU management should lead directly to monitoring of ICP.
Elevating the head of the bed (reverse Trendelenburg position in the absence of clearance of the axial skeleton), a standard maneuver to improve cerebral venous outflow and reduce ICP, has also been shown to generally lower the CPP in the absence of adequate volume resuscitation. Because the reduction in CPP may elevate the ICP per se, it is not advised until complete resuscitation has been accomplished.
Algorithm 21-2. Prehospital evaluation and treatment of a patient with severe traumatic brain injury. “Signs of increased ICP” is the decision point for determining the necessity of intracranial pressure (ICP)-lowering therapy. These signs include pupillary abnormalities, motor posturing, or neurologic deterioration not related to medications. The order of steps is determined by the risk–benefit ratio for individual treatment maneuvers. This algorithm should be viewed as “expert opinion” and used as a framework, which may be useful in guiding an approach to field management of such patients. (© R.M. Chesnut, MD, FCCM, reproduced with permission.)
The confusion and agitation often associated with head injury can increase intracranial hypertension. Therefore, patients with suspected head injury should generally receive sedatives and analgesics whenever possible. Particularly in the TBI patient, the difference between sedation and analgesia should be kept in mind and the two agents titrated specific to their respective indications. Short-acting agents such as propofol are preferable in the interest of following the neurologic examination.
In addition to eliminating any possibility of spontaneous ventilation and mandating complete ventilatory control, pharmacologic relaxation has the undesirable effect of limiting the neurologic examination to the pupils and the CT scan. Its use in the absence of evidence of herniation, therefore, should be limited to situations where sedation and analgesia alone are not sufficient to optimize safe and efficient patient transport and resuscitation.
The “prophylactic” administration of mannitol is not suggested, because of its volume-depleting diuretic effect. In addition, although it is desirable to approximate the lower end of the normal range of PaCO2 during transport of a patient suspected of having brain injury, the risk of exacerbating early ischemia by vigorous hyperventilation outweighs the questionable benefit in the patient without evidence of herniation. Therefore, ventilation parameters consistent with optimal oxygenation and “normal” ventilation are recommended. The minute ventilation should be targeted at 100 mL/kg per minute until quantitative measurement of end-tidal carbon dioxide (EtCO2) or PaCO2 is available. The Guidelines for the Prehospital Management of Severe Brain Injury suggest the use of EtCO2 monitoring during prehospital resuscitation and transport whenever possible. In the absence of signs of intracranial hypertension, ventilation should be adjusted to accomplish a PaCO2 of 35 mm Hg when arterial gas values become available.
Resuscitation in the Presence of Clinical Signs of Herniation
Signs of intracranial hypertension consist of evidence of transtentorial herniation (pupillary dilation or loss of reactivity or motor posturing or flaccidity) or progressive neurologic deterioration not attributable to other causes (e.g., sedation). When such signs occur, aggressive treatment of suspected intracranial hypertension is indicated. Hyperventilation to a PaCO2 target of approximately 30 mm Hg should be accomplished by increasing the minute ventilation to approximately 120 to 140 mL/kg per minute or as directed by quantitative CO2 monitoring. Because hypotension can produce both neurologic deterioration and intracranial hypertension, the use of mannitol is less desirable unless adequate volume resuscitation has been accomplished. If such is the case, however, mannitol should be administered by bolus infusion to establish the optimal osmotic gradient. Under such circumstances, it is critical that the diagnosis and treatment of the neurologic injury be accomplished with utmost haste.
Aggressive restoration of intravascular volume, maintenance of adequate CPP, and avoidance of hypoxia are essential for the management of intracranial hypertension. Volume resuscitation should be vigorous and thorough, with a target of euvolemia. Restrict free water by using isotonic fluids, which makes NS preferable to lactated Ringer solution. Optimally, continuous monitoring of central venous and arterial pressures should be instituted early.
Algorithm 21-3. Evaluation and treatment of the patient with severe traumatic brain injury on arrival at the trauma center. The order of steps is determined by the risk–benefit ratio for individual treatment maneuvers. This algorithm should be viewed as “expert opinion” and used as a framework, which may be useful in guiding an approach to initial hospital management of such patients prior to the initiation of ICP monitoring. (© R.M. Chesnut, MD, reproduced with permission.)
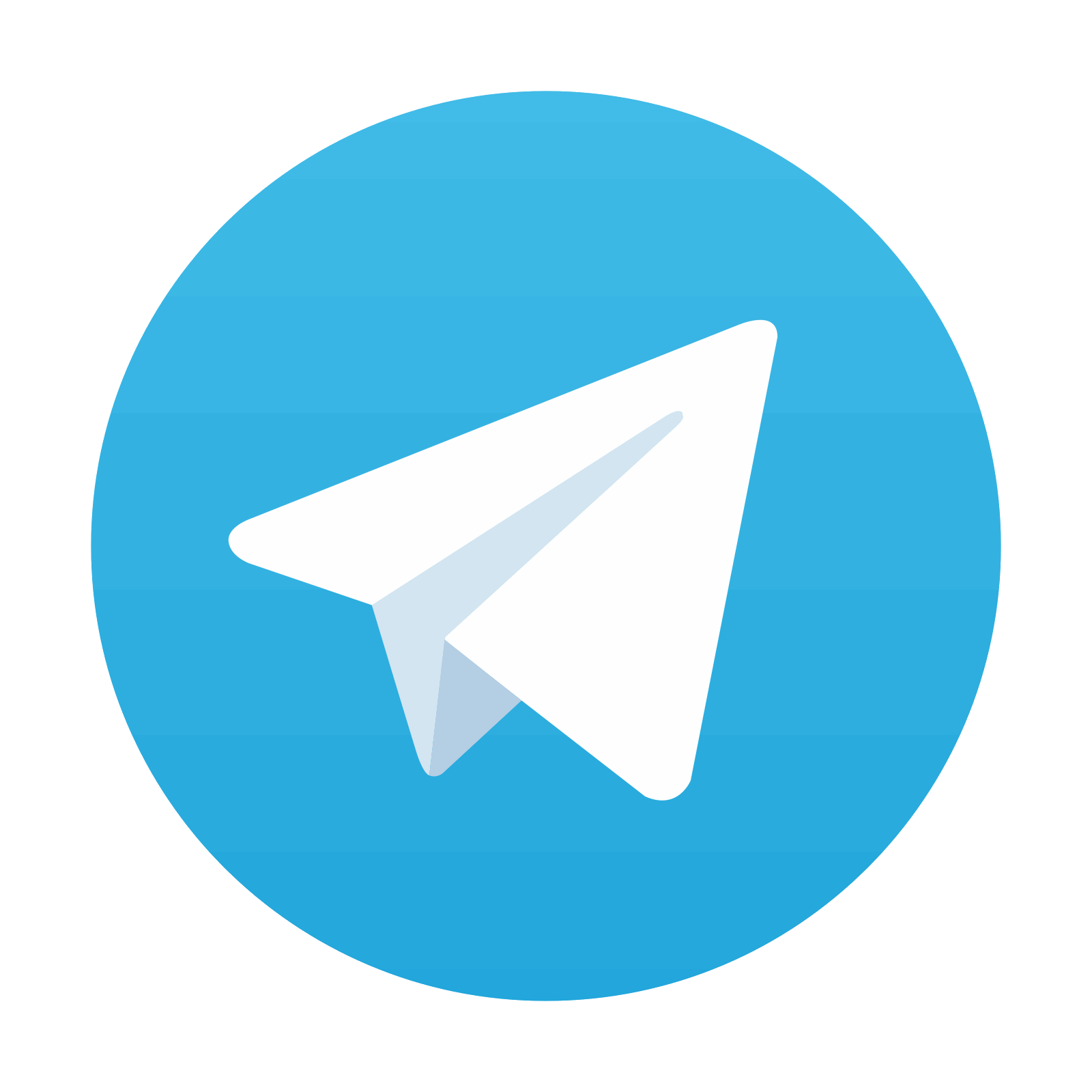
Stay updated, free articles. Join our Telegram channel

Full access? Get Clinical Tree
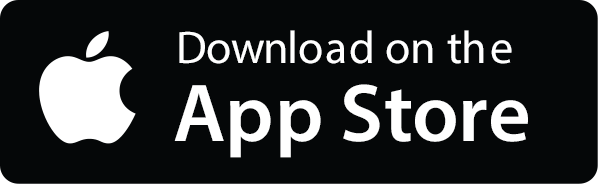
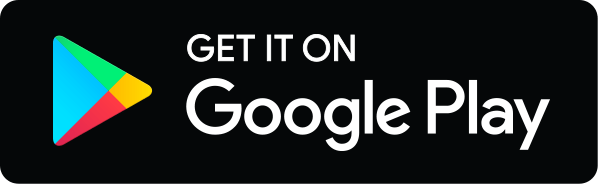