THE TESTES
By the 6th week of development in both sexes, the primordial germ cells have migrated from their extraembryonic location to the gonadal ridges, where they are surrounded by the sex cords to form a pair of primitive gonads. Whether chromosomally 46 XX or 46 XY, the developing gonad at this stage of development is bipotential.
The ovarian pathway is followed unless a gene on the short arm of the Y chromosome, designated TDF (testis-determined factor) also known as sex-determining region Y (SRY) gene, makes a DNA-binding protein that acts as a switch, diverting development into the male pathway. In the presence of the Y chromosome (and due to the SRY protein), the medullary tissue starts forming typical testes with seminiferous tubules and Leydig cells by 7 weeks of gestation. Leydig cells under the stimulation of human chorionic gonadotropin (hCG) from the placenta become capable of androgen secretion by week 10. The spermatogonia, derived from the primordial germ cells by 200 or more successive mitoses, form the walls of the seminiferous tubules together with supporting Sertoli cells.
While the primordial germ cells are migrating to the genital ridges, thickenings in the ridges indicate the developing genital ducts and the mesonephric (formerly called wolffian) and paramesonephric (formerly called müllerian) ducts. In the male, the Leydig cells of the fetal testes produce androgen, which stimulates the mesonephric ducts to form the male genital ducts (ductus deferens, epididymis, seminal vesicles, and ejaculatory ducts), and the Sertoli cells produce a hormone that suppresses formation of the paramesonephric ducts (antimüllerian hormone [AMH]). In the female (or in an embryo with no gonads), the mesonephric ducts regress, and the paramesonephric ducts develop into the female duct system. In the early embryo, the external genitalia consist of a genital tubercle, paired labioscrotal swellings, and paired urethral folds. From this undifferentiated state, male external genitalia develop under the influence of androgens or, in the absence of a testis, female external genitalia are formed regardless of whether an ovary is present.
There are three important steps in sexual differentiation and the development of the normal male phenotype. The first is the differentiation of bipotential gonad primordia (identical in both XX and XY fetuses) into the testes that secrete testosterone, which is under the control of the SRY protein coded by that Y chromosome gene. The second is the development of the internal reproductive tract, initiated by fetal testicular androgen production. In male fetuses, this requires the presence of AMH that causes involution of the müllerian ducts, the anlage for the female type reproductive tract. The third is the development of the external genitalia that requires testosterone and, in some target tissues, its more potent metabolite 5α-dihydrotestosterone (DHT).
Several genes are important for normal gonadal differentiation (and their mutations may lead to disorders of sexual differentiation). Steroidogenic factor 1 (SF-1)1 is important for differentiation and maintenance of both gonadal and adrenal tissue, increasing the synthesis of testosterone and reducing its conversion to estradiol via the transcriptional regulation of the hydroxylases and P450 aromatase, respectively. It may also regulate transcription of the AMH2 gene in synergy with WT1.3 Testicular descent is regulated by the protein product of gene Insl3, which is a member of the insulin-like family and is secreted by Leydig cells. Insl3 seems to be important in gubernaculum formation, and mutations in its gene have been shown to be linked with bilateral cryptorchidism in male mice,4 and in addition, Insl3 protein may also be a biomarker for Leydig cell function. Insl3 also seems to play a role in female fertility (see below).
Functional Anatomy of the Male Reproductive Tract
Adult testes are paired, ovoid organs that hang from the inguinal canal by the spermatic cord, which comprises a neurovascular pedicle, vas deferens, and cremasteric muscle. The testes are located outside the body, encased by a muscular sac. Blood flow is governed by an intricate plexus of arterial and venous blood flow that, together with contraction of the dartos muscle in the scrotal sac, regulates the temperature of the testicles to 2°C below core body temperature. This important function is vital to uninterrupted sperm production. Also encased in the muscular sheath is the spermatic cord, which has the ability to retract the testicles into the inguinal canal in instances of threatened injury. The testes themselves are comprised of two anatomical units: a network of tubules, known as the seminiferous tubules, and an interstitium. The tubules contain germ cells and Sertoli cells and are responsible for sperm production. The testes serve dual functions, which include (1) production of sperm and (2) production of reproductive steroid hormones.5 In the embryonic stage, the dominant male sex hormone, testosterone (T), aids in development and differentiation of the primordial gonads.
Puberty marks the transition from a nonreproductive state into a reproductive state and is associated with adrenarche with increase in adrenal androgen and gonadarche. Tanner staging of the pubertal and axillary hair changes and genitalia is used in children to mark pubertal changes, which are characterized by growth spurt, increased muscle mass, psychological changes, and male pattern hair growth. The earliest sign of puberty in boys is testicular enlargement that results from rising luteinizing hormone (LH) and follicle-stimulating hormone (FSH). After puberty, throughout adulthood, and until late in old age, testosterone helps with sperm production and maintains secondary sexual characteristics.
The sperm move sequentially through the tubuli recti; rete testes; ductuli efferentes testes; the head, body, and tail of the epididymis; and, finally, into the vas deferens. Various secretory products of the seminal vesicles and prostate mix with sperm to form the final product: semen. Seminal vesicle secretions are rich in vitamin C and fructose, important for the preservation of motility of the sperm.
Physiology of the Testicles
Spermatogenesis
Sperm are formed from stem cells called spermatogonia. The spermatogonia undergo mitosis and meiosis; finally, the haploid cells transform to form mature sperm. The mature sperm has a head, body, and tail, which enables it to swim for the purpose of forming a zygote with the haploid ovum. Certain spermatogonia stagger division so that sperm production is uninterrupted and continuous. The Sertoli cells are polyfunctional cells that aid in the development and maturation of sperm.6
Hormonogenesis
Testosterone, the predominant hormone secreted by the testes, is controlled primarily by two pituitary hormones: FSH and LH.7 Because these hormones were first described in women, they are named in reference to the menstrual cycle. Both hormones are produced by a single group of cells in the pituitary called gonadotrophs. FSH acts primarily on germinal stem cells8 and LH acts primarily on the Leydig cells7—located in the testicular interstitium—that synthesize testosterone. Gonadotropins (LH and FSH) are glycoproteins and share an alpha subunit with thyroid-stimulating hormone (TSH) and hCG. The beta subunit confers biological specificity for them.
Hormonal Control of Testicular Function
The hypothalamus, located in the brain, generates a hormone called gonadotropin-releasing hormone (GnRH) in a pulsatile fashion. GnRH is synthesized in neurons situated in the arcuate nucleus and other nuclei of the hypothalamus and is released into the portal hypophyseal system that, in turn, determines the production of LH and FSH from the pituitary gland. Impaired pulse generation of GnRH leads to inadequate production of LH and FSH, resulting in hypogonadism.9 The first, and rate-limiting, step in the testicular steroidogenesis is the conversion of cholesterol to pregnenolone. This cholesterol is either trapped by endocytosis from the blood lipoproteins or synthesized within the Leydig cells. The LH binds to the glycoprotein receptor in the cell wall and induces intracellular cyclic AMP production that, in turn, activates protein kinase A, which catalyzes protein phosphorylation. This latter step induces testosterone synthesis. The testicular steroidogenesis pathway is similar to the pathway in the adrenal cortex and they share the same enzymatic systems. Testosterone is the principal androgen hormone in the blood, and after puberty, the testes secrete 4 to 10 mg daily and less than 5% is derived from adrenal precursors such as dehydroepiandrosterone (DHEA) and androstenedione. It is largely bound, with 2% to 3% free. About 50% of testosterone is bound to albumin and about 45% is bound to sex hormone–binding globulin (SHBG). The concentration of binding protein determines the level of total testosterone but not the free testosterone levels during laboratory estimation. Testosterone and inhibin are the two hormones secreted by the testes that provide feedback control to the hypothalamus and pituitary. Leydig cell steroidogenesis of testosterone is primarily controlled by LH secretion, with negative feedback of testosterone and estradiol on the hypothalamic–pituitary axis. FSH acts on Sertoli cells to stimulate protein synthesis, inhibin, and androgen-binding protein. The actions of both testosterone and FSH on Sertoli cells are synergistic, permitting completion of spermatogenesis. FSH stimulates production of an androgen receptor that makes the Sertoli cell responsive to androgen and, in turn, androgens stimulate synthesis of FSH receptors. Feedback on FSH is attributed to inhibin.10
Testosterone concentration fluctuates in a circadian fashion, reflecting the parallel rhythms of LH and FSH levels. This fact should be considered when interpreting serum levels of testosterone: the highest level is found at about 6 am and correlates with most laboratory normal ranges, and the lowest level is found at about 12 am (in a male with a normal sleep–wake cycle).
Cellular Mechanism of Testosterone Action
Testosterone enters the cell and converts to DHT in cells rich in 5α-reductase, such as some hair follicles and prostate. DHT and testosterone complex with an intracellular receptor protein and this complex bind to the nuclear receptor, effecting protein synthesis and cell growth.
Physiologic Actions of Testosterone
Prenatal Development
Early in development, embryos have primordial components of the genital tracts of both sexes. The primitive gonads become distinguishable at about the 7th week of embryonic stage. Both chorionic gonadotropins and fetal LH stimulate production of testosterone by the fetal Leydig cells. In the fetus, the hypothalamo-hypophyseal vascular connections are responsible for LH release by hypothalamic GnRH and are established between 11 and 12 weeks after conception, which is 3 weeks after testosterone production by the Leydig cells of the testis. hCG, which has LH-like action, made by the placenta, accounts for this gap in stimulation of testosterone production by the fetal testes. Exposure of testosterone to the Wolffian duct leads to differentiation of the various components of the male genital tract. Sertoli cells produce AMH, which aids in regression of the female primordial genital tract. The scrotal skin is rich in 5α-reductase, which converts testosterone to DHT. Fetal exposure to drugs that block this enzyme and DHT formation leads to feminization of the genitalia of the male fetus.
Postnatal Development
Testicular function is reactivated during puberty after a period of quiescence to produce testosterone that results in development of secondary sex hair (face, chest, axilla, and pubis), enhanced linear skeletal growth, development of internal and external genitalia, increased upper body musculature, and development of larynx and vocal cords with deepening of the voice.11, 12, 13 Possible mood changes and aggression are undesired effects that may occur during puberty. The linear growth effects of testosterone are finite, with epiphyseal closure when genetically determined height is achieved. Hypogonadism during puberty leads to imprecise closure of growth plates, leading to excessive height, long limbs, and disproportionate upper and lower body segments. Male secondary sexual characteristics can be staged by a system of development devised by Marshall and Tanner (Table 22.1).
TABLE 22.1 Tanner Staging of Genital and Pubic Hair Development in Males
Effect on Spermatogenesis
Stimulation of Leydig cells induces production of testosterone. Testosterone, acting with FSH, has paracrine effects on the seminiferous and Sertoli cells, inducing spermatogenesis. Exogenous overuse or abuse of testosterone, such as occurs with some athletes, will reduce the high intratesticular concentration of testosterone, leading to reduction of sperm production.
Effect on Secondary Sexual Effects
Testosterone has growth-promoting effects on various target tissues. The secondary sex characteristics that develop during puberty are maintained into late adulthood by testosterone.13 The prostate enlarges progressively during adulthood, while exposure of scalp hair results in regression of the hair follicles (temporal hairline recession). Failure to develop secondary sexual characteristics should prompt evaluation for hypogonadism or constitutional delay in boys. Loss of secondary sexual characteristics might occur gradually and should prompt evaluation for hypogonadism because, among other effects, low testosterone levels lead to loss of bone mass and development of osteoporosis in males at any age.
Disorders of Sexual Development and Testicular Hypofunction
Pubertal development could be premature (precocious) or delayed, even if development is normal at birth.14,15 Precocious sexual development results from the premature exposure of sex steroids, which might arise from early gonadotropin secretion or production by the adrenal glands or testes. Detailed descriptions of the sequence of hormonal pubertal abnormalities of hair, genitals, and breasts are beyond the scope of this text. The differential diagnosis of hypogonadism includes a diverse group of disorders affecting the testicles and the hypothalamic–pituitary regulation of the testes outlined in Box 22.1. Certain important disorders are explained in the following section.
BOX 22.1 Causes of Delayed Puberty
- Delayed puberty or hypogonadism, with increased gonadotropins (FSH and/or LH)
- Klinefelter’s syndrome
- Bilateral gonadal failure
- Primary testicular failure
- Anorchia
- Vanishing testicles
- Chemotherapeutic agents
- Irradiation
- Trauma
- Infection (mumps orchitis)
- Delayed puberty with normal or low FSH and/or LH
- Constitutional delayed puberty16
- Hypothalamic dysfunction
- Malnutrition
- Chronic systemic illness
- Severe obesity
- Central nervous system tumors
- Hypopituitarism
- Panhypopituitarism
- Kallmann’s syndrome (anosmia, cleft palate, and reduced FSH and LH levels)
- Isolated GH deficiency
- Hyperprolactinemia (prolactinoma or drug induced)
- Hypothyroidism
- Miscellaneous
- Prader-Willi syndrome
- Laurence-Moon syndrome
- LEOPARD syndrome
- Bloom syndrome
- Germ cell neoplasia
- Male pseudohermaphroditism
- Ataxia–telangiectasia
- Steroidogenic enzyme defects
Hypergonadotropic Hypogonadism
Hypergonadotropic hypogonadism incorporates a group of disorders characterized by low testosterone, elevated FSH or LH, and impaired sperm production.
Klinefelter’s Syndrome
Klinefelter’s syndrome occurs in about 1 of 400 to 600 men and is caused by the presence of an extra chromosome; in fact, it is the most common human sex chromosome abnormality. The most common karyotype is 47, XXY.11. Men with this disorder have small (<2.5 cm), firm testicles. Gynecomastia (enlargement of the male breast) is commonly present at the time of diagnosis. Due to reduced production of testosterone, LH levels are elevated. Due to deficient seminiferous tubule mass, FSH is elevated from underproduction of inhibin.17 These men also have azoospermia and resultant sterility. Men with mosaicism may produce some sperm and pregnancies have been reported with such men. Elevated levels of FSH and LH induce increased aromatase activity, resulting in elevated estrogen levels. Men with Klinefelter’s syndrome may have reduced bone density and breast cancer risk comparable to women.
Testicular Feminization Syndrome
Testicular feminization syndrome is the most severe form of androgen resistance syndrome, resulting from mutations of the androgen receptor and impaired androgen actions in target tissues. As a result of the lack of androgen and unopposed estrogen effects, the physical development pursues the female phenotype, with fully developed breast and female distribution of fat and hair. Most present for evaluation of primary amenorrhea, at which time the lack of female internal genitalia becomes apparent. The testicles are often undescended, and failure to promptly remove these organs is essential to abort malignant transformation. Biochemical evaluation reveals normal or elevated serum concentrations of testosterone with elevated FSH and LH levels. There is no utility or response to administration of exogenous testosterone. They are reared as girls. There is a wide variation in androgen insensitivity and in corresponding clinical deficits in male sexual development.
5a-Reductase Deficiency
Deficiency of 5α-reductase is a rare cause of androgen insensitivity and results in a mutation encoding the type 2 isoenzyme, maps to chromosome 2p23, and is expressed in XY males. A reduction in levels of the enzyme 5α-reductase results in decreased DHT concentrations. Physical development is similar to the female phenotype until puberty when the wolffian ducts virilize in response to testosterone. The female internal genitalia are absent and the male internal genitalia are well developed (epididymis, vas deferens, and seminal vesicle) in response to testosterone. DHT is essential for the development of the prostate and external genitalia during embryonic virilization.
Myotonic Dystrophy
Myotonic dystrophy (type 1 caused by DMPK gene mutation and type 2 caused by CNBP gene mutation) is inherited in an autosomal dominant fashion (almost always, an affected person has one parent with the condition) and presents with primary hypogonadism, frontal balding, diabetes, and muscle weakness, atrophy, and dystonia (an inability of the muscle to relax adequately after contraction). The incidence of DM is 1 in 8,000 worldwide. Testicular failure typically presents in the twenties and thirties; however, puberty progresses normally and male secondary sexual characteristics, height, bone growth, etc., are attained normally during pubertal course of development. Primary hypogonadism occurs with primarily germ cell compartment failure (i.e., oligozoospermia and infertility), with elevated serum FSH. In later stages, failure of Leydig cell compartment resulting in low serum testosterone, elevated LH concentrations, and testicular atrophy occurs. Testicular failure happens more commonly in DM1 (type 1 myotonic dystrophy) than in DM2.
Testicular Injury and Infection
Mumps orchitis develops in a third of postpubertal males with mumps and is the most frequent extrasalivary manifestation of this highly contagious infection. Mumps epididymo-orchitis is usually unilateral (85% of the times); therefore, permanent sterility is rare (contrary to popular belief). Most cases of orchitis occur within 4 to 6 days of parotitis, but it may precede parotitis or be the only manifestation of mumps. Intermittently, mumps outbreaks have been reported in secondary schools, colleges, universities, and military groups; however, the absolute numbers are still quite low at a few hundred cases reported in a year in the United States. Other infectious causes of orchitis like TB, Chlamydia, gonorrhea, etc., are fortunately rare in the United States. Radiation and chemotherapy for cancer can result in long-term testicular damage.
Sertoli Cell–Only Syndrome
Sertoli cell–only syndrome (SCO syndrome or germ cell aplasia) is characterized by a lack of germ cells. Men present with small testes, high FSH levels, azoospermia, and normal testosterone levels. Testicular biopsy, which is necessary for diagnosis, shows lack (or complete absence) of spermatozoa. This disorder may arise from Y chromosome microdeletions on Yq11 locus (AZF region: Azoospermia Factor). It is relatively rare, affecting 5% to 10% of all infertile men in the country.
Hypogonadotropic Hypogonadism
The hallmark of disorders of hypogonadotropic hypogonadism is the occurrence of low testosterone levels together with low or inappropriately normal FSH or LH levels.
Kallmann’s Syndrome
The impaired secretion of GnRH has been elucidated in the X-linked form of congenital GnRH deficiency, which results from impaired migration of GnRH neurons and olfactory nerves to the ventral hypothalamus during embryogenesis. Proper migration of these neurons is dependent on the correct expression of anosmin, a 680–amino acid neural cell adhesion molecule–like protein, which is the product of the KAL1 gene.
Mutations in the KAL1 gene (a gene found on the X chromosome) occur less often in sporadic cases (<10%). In general, the clinical phenotypes of idiopathic hypogonadotropic hypogonadism (IHH) subjects with KAL1 mutations are characterized with a high incidence of microphallus, cryptorchidism, and small testes. X-linked KAL mutations have never been observed in families with normosmic IHH (nIHH) or in families with both anosmic and nonanosmic individuals.18,19
Kallmann’s syndrome is a result of an inherited, X-linked recessive trait that manifests as hypogonadism during puberty. The frequency of this syndrome is 1 of 10,000 males. Certain men also have red-green color blindness, congenital deafness, or cerebellar dysfunction.
Hyperprolactinemia
Prolactin elevation resulting from any cause (drug-induced or prolactin-producing tumors of the pituitary) can result in hypogonadotropic hypogonadism20,21 due to impairment of both frequency and amplitude of FSH and LH pulses, because of hypothalamic kisspeptin neuron–mediated disruption of GnRH pulsatile secretion.22,23
Type 2 Diabetes
Type 2 diabetes is also associated with hypogonadotropic hypogonadism in at least 25% to 50% of men.24 It is characterized by low free or total serum concentrations of testosterone and inappropriately low LH.25 This may stem from insulin resistance (insulin action seems to be important for LH release by gonadotropes), low SHBG levels, inflammation (hypogonadism in type 2 diabetic males was seen to be associated with high C-reactive protein levels, an inflammatory marker),24 and even elevated estradiol levels (due to testosterone aromatization in adipose tissue). Of note, type 1 diabetes does not seem to be associated with hypogonadism, provided glycemic control has remained good and BMI is within normal limits.26
Age
There is a gradual reduction in testosterone after age 30, with an average decline of about 110 ng/dL every decade. The Baltimore Longitudinal Study of Aging revealed “hypogonadism” (reduced total testosterone concentrations) of 19% at age 60, 28% at age 70, and 49% at age 80,27 with free testosterone concentrations much lower in these men. Age is also associated with elevation of SHBG by about 1%/year. Similar findings emerged from the Massachusetts Male Aging Study, which showed 1.6%/year decline in total testosterone levels and 2% to 3%/year decline in bioavailable testosterone (free and albumin-bound testosterone) levels.28 Recently, the results of the Testosterone Trials were published that showed significant improvement in sexual function in older men greater than 65 years, given testosterone replacement for 1 year, but no significant improvement in physical function or vitality.29
Pituitary Disease
Acquired hypogonadism can follow injury to the pituitary as a result of tumors, surgical- or radiation-induced trauma, vascular injury, autoimmune hypophysitis, or granulomatous or metastatic disease. Hemochromatosis is a rare cause of pituitary dysfunction.
Opioid Use
Long-term or continuous use of narcotics has been linked to severe hypogonadotropic hypogonadism (due to μ-opioid receptor–mediated decreased GnRH pulsatile production) and even decrease in male fertility (decreased sperm motility, decreased sperm counts, and abnormal sperm morphology).30
Obstructive Sleep Apnea
It is unclear if sleep apnea leads to hypogonadotropic hypogonadism (due to hypoxemia and sleep deprivation),31 or if the obesity (which is often present in men with sleep apnea) leads to decreased testosterone levels. It is to be noted that testosterone replacement therapy (especially high-dose testosterone) may worsen preexisting sleep apnea, possibly due to increased oxygen consumption; and therefore, the Endocrine Society guidelines32 recommend not starting testosterone replacement therapy in men with untreated severe OSA, until first starting them on CPAP.
Diagnosis of Hypogonadism
Both clinical and biochemical features must be met (Fig. 22.1) to make the diagnosis of hypogonadism. Testosterone concentrations have a circadian rhythm and the time of sampling must be considered. Morning samples between 8 and 10 am are recommended to match values from reference interval studies. Multiple estimation of free and bound testosterone levels should be done on different days before a diagnosis of testosterone deficiency is confirmed.32 The distinction between primary (disease or destruction of the testes) versus secondary (disease or destruction of the pituitary) or tertiary (hypothalamic) is relatively easy to make. FSH and/or LH33 values are elevated in primary hypogonadism and are inappropriately normal or low with secondary or tertiary etiologies. Pituitary MRI should be done in secondary hypogonadism in young individuals. Older individuals often have secondary or tertiary (hypothalamic) dysfunction as a result of reduced hypothalamic pulse generator frequency, resulting in low or inappropriately normal FSH and/or LH levels.34 Clinical signs and symptoms of hypogonadism (e.g., loss of secondary sexual characteristics, decreased muscle mass, osteoporosis, etc.) should be corroborated with low testosterone levels, particularly when testosterone replacement therapy is considered.32
FIGURE 22.1 Clinical diagnostic evaluation of male hypogonadism. PRL, prolactin; FSH, follicle-stimulating hormone; LH, luteinizing hormone; hCG, human chorionic gonadotropin; GnRH, gonadotropin-releasing hormone.
Testosterone Replacement Therapy
The following principles should guide testosterone therapy: testosterone should be administered only to a man who is hypogonadal, as evidenced by clinical symptoms and signs consistent with androgen deficiency and a distinctly subnormal serum testosterone concentration. Treating symptoms of hypogonadism without corroborating biochemical evidence of testosterone deficiency is not recommended. Restoring testosterone to midnormal concentrations can be achieved satisfactorily whether the testosterone deficiency is due to primary or secondary hypogonadism.
The currently available modes35 of testosterone administration in the United States are as follows:
1. Parenteral testosterone. This is the most widely available and cost-effective mode of administration. The cypionate and enanthate esters of testosterone are available for intramuscular injection. The peak level is achieved in 72 hours and the effect lasts for a period of 1 to 2 weeks. Weekly administration provides for a lower peak and less fluctuation within the normal range of testosterone levels. Usual dosing is 50 to 100 mg weekly or 150 to 250 mg once every 2 weeks. Testosterone dose should be based on lean body mass, not on body weight, and is best reached by administering a standard dose of testosterone, with minor dose escalations based on serum testosterone levels measured midpoint between two injections. The goal is to maintain this middose level at midpoint of the normal ranges. Recently, FDA has approved extra-long-acting testosterone undecanoate injection, which can be injected every 10 weeks (after the first 2 doses, which need to be injected in a 4-week interval), but this injection is available only under AVEED REMS program,36 due to possible risk of serious adverse effects of pulmonary oil microembolism (POME) and anaphylaxis.
2. Transdermal testosterone patch therapy. This mode of administration provides more physiologic levels of testosterone. The patch is permeability enhanced to aid in the absorption of testosterone through normal skin. Local skin irritation can occur and often limits the patch use.
3. Testosterone gel. This hydroalcoholic gel preparation is applied to nongenital skin once daily. The absorption is gradual and provides blood levels of testosterone in the normal range for 24 hours. The main concern with this preparation is potential transmission to female partners or children on close skin contact.
4. Testosterone buccal pellet. This plastic tablet is placed along the gum line twice daily. Local discomfort (irritated gums) and the need for twice-daily dosing sometimes limit use.
5. Subcutaneous testosterone pellet (Testopel) involves implantation of three to six 75 mg testosterone pellets every 3 to 6 months, into the subdermal fat of the buttocks, lower abdominal wall, or thigh with a trocar under sterile conditions using a local anesthetic. This is not a very frequently used mode of testosterone replacement due to pellet extrusion, fibrosis, infection, and the need for an incision to implant the Testopel.
6. Nasal testosterone gel (Natesto). Natesto is administered into each nostril by a metered-dose pump applicator three times daily. The risk of testosterone transfer from a patient to his partner or children is mitigated, but the complications of testosterone replacement are acne, polycythemia, prostate enlargement, possible growth-promoting effect on undiagnosed prostate cancer, worsening of obstructive sleep apnea (OSA), peripheral edema, and gynecomastia.
Oral alkylated androgens are not recommended due to adverse effects of decreased high-density lipoprotein,37 increase in low-density lipoprotein (LDL), and also incidence of cholestatic jaundice and peliosis hepatitis.
Monitoring Testosterone Replacement Therapy
Prostate-specific antigen (PSA), blood counts (for hematocrit), and lipid levels should be checked 3 to 6 months after initiation of testosterone replacement and at least yearly thereafter. Routine clinical evaluation for leg edema, worsening of sleep apnea, and prostate enlargement is also recommended. Pharmacologic use of testosterone may also reduce sperm count by reducing the intratesticular testosterone concentration that is manyfold higher than serum concentrations. If PSA elevation is noted after testosterone replacement, prostate evaluation with possible biopsy is recommended. Prostatic carcinoma is a contraindication to testosterone replacement.
THE OVARIES
Early Ovarian Development
If no Y chromosome or TDF is present, the gonad, by default, forms an ovary; the cortex develops, the medulla regresses, and oogonia begin to develop within follicles. The oogonia are derived from the primitive germ cells by a series of about 30 mitoses, far fewer than the number required for spermatogenesis. Beginning at about the end of the 3rd month, the oogonia enter meiosis I, but this process is arrested at a stage called dictyotene, in which the cell remains until ovulation occurs many years later (this cell is called primary oocyte). Many of the oogonia degenerate before birth, and only about 400 mature into ova during the 30 years or so of sexual maturity of the female. It is evident that the gonads are paired bilateral structures; in very rare circumstances, the developmental processes may be different on either side. Estrogen formation in the fetal ovary begins in early development despite primordial follicles not having begun forming until the second trimester of pregnancy (at 16 weeks of gestation). The gonadotropins from the pituitary gradually take over the role of maternal placental hCG, and fetal pituitary LH and FSH peak near midgestation and then fall to low concentrations at birth. Postpartum, a smaller peak of LH and FSH occur, which stimulates steroid secretion leading to neonatal milk production from the breast.
Pubertal Changes of Ovarian Function
The onset of puberty is characterized by increasing secretion of LH and FSH that stimulates gonadal activity and is driven by increased activity of the hypothalamic GnRH neurons. In the ovary, both LH and FSH are involved in the control of steroidogenesis, and there are numerous isoforms of circulating LH and FSH and their biological potency depends on the degree of glycosylation, which is associated with differences in assays for LH and FSH. The genes coding LH and FSH receptors are on chromosome 2p21. Inactivating mutations of the β-chain of the LH receptor have been described. Before the onset of puberty, both LH and FSH are secreted in small amounts, but as puberty approaches, the amplitude of LH and FSH pulsatile secretions increases and the nocturnal rise in LH secretion increases. The nocturnal rise disappears with adulthood.
The ovaries are paired organs that, like the male gonads, perform the dual functions of gamete (ovum) and steroid hormone production.38, 39, 40 Unlike in the male, the primordial reproductive cells in the female typically produce a solitary gamete. Ovarian and menstrual events are carefully synchronized by a complex interplay of hormones among the hypothalamus, pituitary, and ovaries to prepare the uterus for implantation of an embryo. In the absence of implantation, the uterine lining is shed, resulting in menses.41,42
The length of the menstrual cycle is the time between any two consecutive cycles. The typical duration is 25 to 35 days, with average menstrual flow about 3 to 6 days.42
Functional Anatomy of the Ovaries
The ovaries are oval organs that lie in the pelvic fossa, formed by the posterior and lateral pelvic wall, and attach to the posterior surface of the broad ligament by the peritoneal fold, otherwise known as the mesovarium. They are positioned near the fimbrial end of the fallopian tubes, which are connected to the uterine cavity. An adult ovary averages 2 to 5 cm in length, weighs an average of 14 g, and typically contains 2 to 4 million primordial follicles.43 These primordial follicles are present at birth; however, maturation is blocked until puberty. Following the onset of puberty, each ovarian cycle is marked by recruitment of a few primordial follicles for maturation. Typically, all but one of these follicles will then atrophy, in a process termed the follicular phase.
The single remaining follicle—known as the graafian follicle—is composed of an outer and inner layer (the theca externa and theca interna, respectively) encasing a central fluid-filled cavity and a layer of cells known as the granulosa layer.44, 45, 46 The maturing ovum attaches to the inside of the follicle via cells derived from granulosa cells, called cumulus cells. During the luteal phase of the ovarian cycle, the graafian follicle releases its ovum in response to ovarian stimulation by LH. When the ovum is extruded, the graafian follicle undergoes a morphologic change with hypertrophy of the theca and granulosa cells to become the corpus luteum. This process is called luteinization. The corpus luteum is rich in cholesterol and acts as a substrate for continued production of progesterone and estrogen, maintaining the endometrium for conception. If conception or implantation fails to occur, the endometrium is shed and the corpus luteum atrophies to an atretic follicle.
Hormonal Production by the Ovaries
As in the adrenal glands and the testes, the steroidogenic pathway and synthetic enzymes are present in the ovaries. Cholesterol is either synthesized from acetate or actively transported from the LDL particle in blood and then used as a substrate for hormonal production.47
Estrogen
Naturally synthesized estrogens are carbon-18 compounds. The principal estrogen produced in the ovary is estradiol. Estrone and estriol are primarily metabolites of intraovarian and extraglandular conversion. Estrogens promote breast, uterine, and vaginal development and also affect the skin, vascular smooth muscles, bone cells, and the central nervous system.48 The lack of estrogen that naturally occurs with the onset of menopause leads to atrophic changes in these organs.49 During the reproductive period, it is estrogen that is responsible for follicular phase changes in the uterus, with deficiency resulting in irregular and incomplete development of the endometrium.
Progesterone
Progesterone is a carbon-21 compound within the steroid family and is produced by the corpus luteum. Progesterone induces the secretory activity of those endometrial glands that have been primed by estrogen, readying the endometrium for embryo implantation. Other effects include thickening of the cervical mucus, reduction of uterine contractions, and thermogenic effect, in which basal body temperature rises after ovulation. This effect is of clinical use in marking the occurrence of ovulation. Progesterone is the dominant hormone responsible for the luteal phase, and deficiency results in failure of implantation of the embryo.50
Androgens
Ovaries produce the androgens androstenedione, dehydroandrostenedione, testosterone, and DHT, all of which are carbon-19 compounds. Excess production of ovarian androgens in women leads to excess hair growth (hirsutism), loss of female characteristics, and—in severe cases—development of overt male secondary sexual features (masculinization or virilization).50, 51, 52 Unlike estrogen, which is not produced in the ovary after menopause, ovarian androgen synthesis continues well into advanced age.53
Others
Inhibins A and B, which are produced by the ovaries, are hormones that inhibit FSH production.54 Activin is a hormone that enhances FSH secretion and induces steroidogenesis.54 Folliculostatin, relaxin,55 follicle regulatory protein, oocyte maturation factor, and meiosis-inducing substance are hormones that appear to have important, yet not clearly characterized, functions.
The Menstrual Cycle
By convention, the menstrual cycle is considered to start on the 1st day of menses (day 1). The menstrual cycle consists of two phases of parallel events occurring at the ovaries and endometrium. Within the ovaries, these events are known as the follicular and luteal phases, while the concurrent endometrial events are known as the proliferative and secretory phases.56
The Follicular Phase
The follicular phase begins with the onset of menses and ends on the day of LH surge. Early in the follicular phase, the ovary secretes very little estrogen or progesterone. A rise in FSH, however, stimulates estrogen production. The estrogen secreted by the developing follicle within the ovary stimulates uterine epithelial cells, blood vessel growth, and endometrial gland development to increase the thickness of the endometrium.
The Luteal Phase
Estrogen levels peak 1 day before ovulation, at which point a positive feedback system results in an LH surge. The start of the luteal phase is marked by the extrusion of the ovum approximately 36 hours after this LH surge, with subsequent luteinization of the graafian follicle to form the corpus luteum. The corpus luteum secretes progesterone to aid in the implantation of the embryo. The intense secretory capacity of the uterine glands aids the implantation of the embryo. In the absence of fertilization, with a gradual decline in the production of progesterone and estrogen by the corpus luteum, there is a loss of endometrial blood supply; this results in shedding of the endometrium approximately 14 days after ovulation occurred. The typical duration of menstrual bleeding is 3 to 5 days, with blood loss averaging 50 mL. Onset of menses marks the end of the luteal phase.
Hormonal Control of Ovulation
The central control of FSH and LH secretion resides in the GnRH pulse generator of the arcuate nuclei and medial preoptic nuclei of the hypothalamus.56 Positive and negative feedback responses exist among estrogen, progesterone, LH, and FSH production. It is because of the lack of estrogen after menopause that both FSH and LH levels rise.57 During reproductive years, FSH levels are elevated early in the follicular phase. A midcycle surge in LH production stimulates a series of events that culminate in ovulation, with FSH levels falling after this event. Any injury to the hypothalamus or the presence of either psychosocial or physical stressors leads to changes in these hormonal cues and results in anovulation and amenorrhea.58
CASE STUDY 22.1
A 39-year-old woman presented with hot flashes and irregular menstrual cycles for 6 months. Clinical examination did not reveal abnormality. Laboratory evaluation reveals the following: CBC, normal; blood glucose, 89 mg/dL; TSH, 1.5 mIU/L; FSH, 128 IU/L; and LH, 30 IU/L.
This clinical situation is consistent with one of the following:
1. PCOS
2. Prolactinoma
3. Pituitary tumor
4. Menopause
5. Hypothalamic hormone deficiency
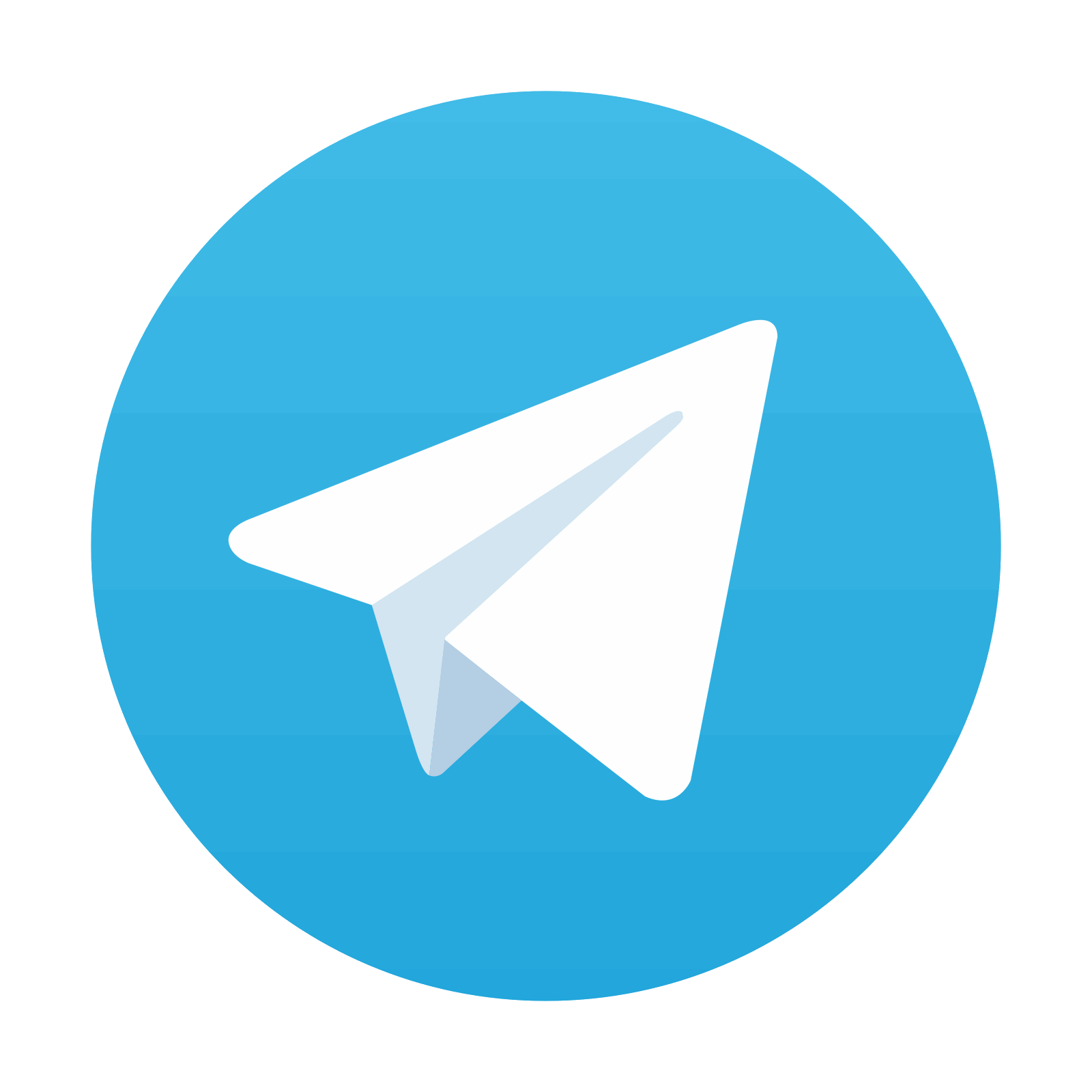
Stay updated, free articles. Join our Telegram channel

Full access? Get Clinical Tree
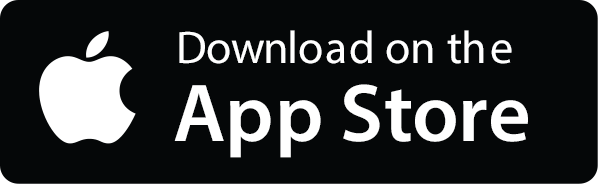
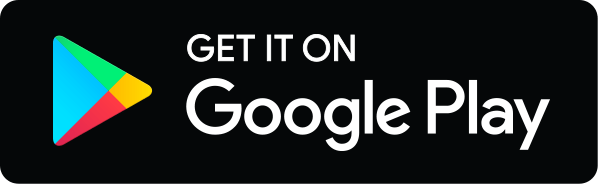