Gastrointestinal Function
Jeffrey S. Sartin
Key Questions
• What are the major structures of the gastrointestinal tract and their corresponding functions?
• How does the autonomic nervous system influence gastrointestinal motility?
• How and where are complex carbohydrates, proteins, and lipids digested and absorbed?
• How and where are water and electrolytes absorbed?
• What alterations in gastrointestinal function occur in association with very young or very old age?
http://evolve.elsevier.com/Copstead/
The gastrointestinal (GI) system represents a remarkable interface between the human organism and the external environment for the purpose of providing nutrients for the body. The components of the GI tract can be thought of as a continuous tube about 7 m in length extending from the mouth to the anus. Beginning with the mouth and pharynx, the GI tract includes the esophagus, stomach, and small and large intestines (Figure 35-1). Other parts of the GI system located outside the GI tract include the salivary glands, the pancreas, and the biliary system (liver, gallbladder, and bile ducts).
The process of ingesting nutrients, propelling them through the GI tract, and transforming them into a form capable of absorption into the body’s internal milieu is remarkably complex. The general functions of the GI tract can be divided into (1) movement of nutrients, including propulsive and mixing movements; (2) secretion of digestive juices; (3) digestion of nutrients; and (4) absorption of nutrients.1,2 This chapter describes each of these functions in detail and provides an overview of the structure and organization of the GI tract and its growth and alteration across the life span.
Structure and Organization of the Gastrointestinal Tract
Embryology
As early as the third week of gestation, the embryonic structure begins to fold inward to create the primitive gut. By the fourth week structures of the foregut, midgut, and hindgut are in place.3
The foregut develops into the pharynx, the esophagus, the stomach, the duodenum proximal to the opening of the common bile duct, the hepatobiliary system, and the pancreas. The midgut forms the small intestine (below the opening of the common bile duct), the cecum, the appendix, the ascending colon, and the proximal portion of the transverse colon. The hindgut develops into the distal part of the transverse colon, the descending and sigmoid colon, the rectum, and the superior portion of the anal canal.
Developmental abnormalities are relatively common as a result of incomplete partitioning during gut differentiation. The trachea and esophagus share a common developmental origin, and incomplete development of these structures may lead to tracheoesophageal fistula, an anomaly characterized by an abnormal connection between the trachea and esophagus (often accompanied by esophageal atresia, where the esophagus is closed off in a blind pouch). These disorders occur in about 1 in 4000 live births, with about one third of affected infants born prematurely. One of the most serious surgical emergencies in newborns, tracheoesophageal fistula requires immediate diagnosis and surgical correction.4 The current prognosis for such infants is very good, with about 90% survival beyond the neonatal period.
Failure of normal partitioning between the foregut and midgut can lead to intestinal atresia, a condition in which the lumen of the small intestine is obliterated. This is an important cause of intestinal obstruction in the newborn and necessitates prompt surgical correction.4 Failure of the midgut to develop or rotate properly with respect to the umbilical cord can result in omphalocele, a congenital herniation of viscera into the base of the umbilical cord.
Congenital malformations resulting from inappropriate development of the anorectal portion of the GI tract include colonic or anal agenesis, a condition in which the rectal pouch ends blindly. This condition has been rarely reported with congenital varicella syndrome.4 Other developmental anomalies of this portion of the GI tract include anal stenosis, in which the anal aperture is small, and anal atresia (or imperforate anus), in which the anal membrane persists and covers the aperture to create an obstruction.
After its initial embryologic development, the GI tract continues to grow in length and diameter until somatic growth ends with puberty. General factors such as adequate nutrition and appropriate levels of insulin, growth hormone, thyroid hormone, cortisol, androgens, and estrogens play a role in GI development, as well as local factors such as the direct effect of ingested nutrients, GI hormones, and secretions.5
Functional Anatomy
Each part of the GI tract is uniquely adapted for a specific function in providing nutrients for the body. The role of each major component of the GI tract will be described in some detail.
Oral Cavity and Pharynx
The mouth, or oral cavity, is the usual point of entry for nutrients and is the site of the initial breakdown of nutrient substances into a form usable by the body. Food is pushed toward the side of the mouth by the tongue to facilitate chewing and grinding on the surfaces of the molar and premolar teeth. As the food is manipulated and broken down, it is moistened by saliva secreted by three major pairs of salivary glands: the parotid, submandibular, and sublingual glands (Figure 35-2). Saliva serves three major functions: (1) by its moistening action, saliva allows the tongue to convert a mouthful of food into a bolus, or semisolid mass, that can be swallowed easily; (2) it allows for taste perception by the papillae on the surface of the tongue, which are sensitive to chemical differences among dissolved food molecules; and (3) the digestive enzyme contained in saliva, salivary amylase (also called ptyalin), initiates carbohydrate digestion by breaking down polysaccharides (also called starch) into the simpler molecular structures of dextrin and maltose.6,7 Important changes in oral structure and function that occur with aging are detailed in Geriatric Considerations: Changes in the Mouth.
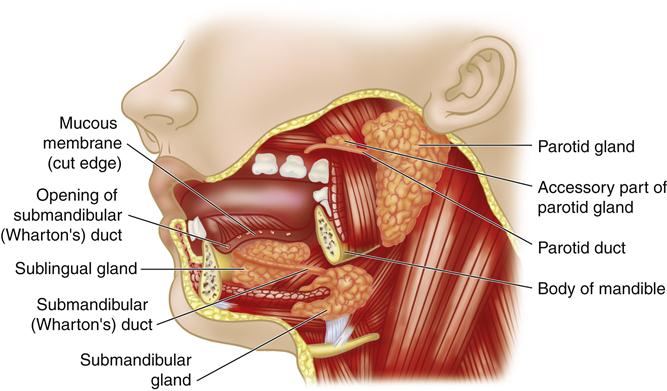
Structures of the mouth and location of the salivary glands. (From O’Toole M, editor: Miller-Keane encyclopedia and dictionary of medicine, nursing, and allied health, ed 7, Philadelphia, 2003, Saunders.)
The pharynx, or throat, is about 12 cm long and serves as the entryway for both the respiratory and the GI systems. The oropharynx, the portion of the pharynx posterior to the mouth, is separated from the nasopharynx, the portion of the pharynx posterior to the nose, by the soft palate. The laryngopharynx is the portion of the pharynx that opens into the larynx and the esophagus. During swallowing, the soft palate is pulled upward to close off the nasopharynx. The bolus of food being swallowed is propelled by reflex movements of muscles in the pharynx through the laryngopharynx and into the esophagus. Simultaneously, the opening to the larynx is closed by the epiglottis. This coordinated set of actions prevents food substances and liquids from inadvertently entering the respiratory system, a potentially life-threatening occurrence referred to as aspiration.
Esophagus
The esophagus is a muscular tube approximately 25 cm in length that initiates the progress of food through the gut after ingestion. Passage of food through the esophagus is greatly facilitated by mucus secreted by cells in the epithelial lining. Extremely rough or fibrous foods may potentially penetrate the mucous lining of the esophagus and cause damage. The stratified squamous epithelium lining the esophagus is constantly renewed by cells moving to the surface from below, thus providing a means of epithelial renewal. The esophagus propels nutrients to the stomach by means of strong muscular contractions. (Presbyesophagus, or abnormal esophageal motility occurring with advanced age, is described in detail in the Age-Related Changes section.) When the body is in an upright position, gravity assists in the downward movement of food to the stomach. However, the muscular contractions of the esophagus are extremely strong and are sufficient to transport nutrients to the stomach even in the absence of gravity, as persons living (and eating) in the weightless conditions of space have demonstrated.1,3
At the lower end of the esophagus, about 2 to 5 cm above its juncture with the stomach, the circular muscle of the esophagus functions as a sphincter; this region is referred to as the lower esophageal sphincter (LES). Although anatomically this sphincter is no different from the remainder of the esophagus, it remains tonically constricted, in contrast to the middle and upper portions of the esophagus, which are completely relaxed under normal conditions.2 Thus the LES serves to prevent the highly acidic gastric contents from moving in a retrograde motion back into the esophagus. Under certain conditions the LES does not function properly, and reflux of gastric contents into the esophagus gastroesophageal reflux disease (GERD) may occur. The resulting subjective sensation of irritation and spasms of the distal portion of the esophagus is often referred to as heartburn or dyspepsia.
Stomach
The stomach (Figure 35-3) is essentially an elastic food reservoir. Under normal circumstances, its capacity is 1000 to 1500 ml, although a capacity of as much as 6000 ml is possible.2 The portion of the stomach immediately below the LES is called the cardia. The fundus is the part of the stomach that continues lateral to and above the cardia; the body of the stomach extends from the cardia to the antrum, which stretches from the angulus to the pylorus. The antrum differs markedly from the rest of the stomach in function and is distinguished by the absence of rugae, the folds present in the mucous membrane of the other areas of the stomach. The pylorus is a muscular sphincter between the stomach and duodenum that serves to control gastric emptying and limits the reflux of bile from the small intestine.
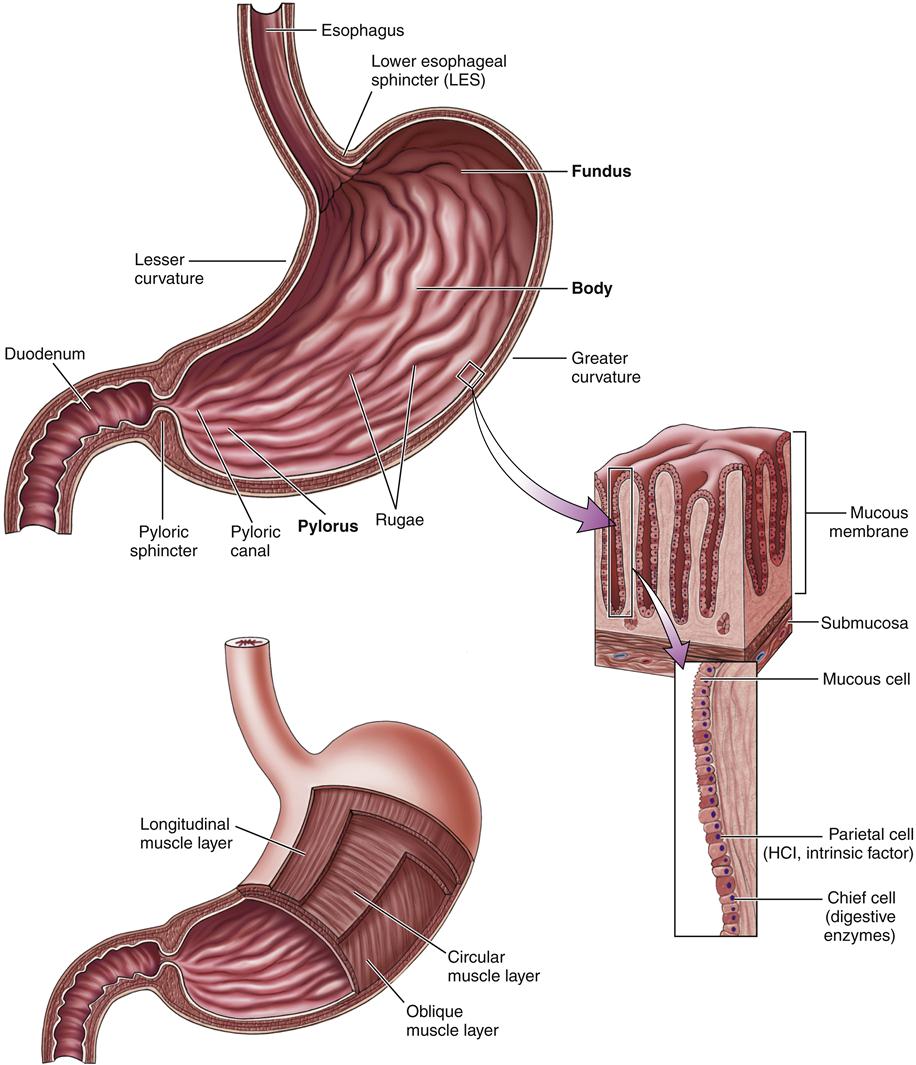
HCl, Hydrochloric acid. (From Herlihy B: The human body in health and illness, ed 4, Philadelphia, 2011, Saunders.)
The stomach is lined with simple columnar epithelium containing millions of gastric glands that extend down to the mucosa. A typical gastric gland is shown in Figure 35-4. As shown in this illustration, gastric glands are lined by several types of specialized cells. Chief cells produce pepsinogen, the inactive form of the enzyme pepsin; parietal cells produce hydrochloric acid and also a substance called intrinsic factor that is needed for adequate intestinal absorption of vitamin B12.8 Mucous cells produce an alkaline mucus that serves to shield the stomach wall and neutralize the acidity in the immediate area of the lining. A layer of mucus more than 1 mm thick continuously bathes the free surfaces of the gastric epithelial lining. In addition to these cells, gastrin cells are located in the antral epithelium and have surface microvilli that monitor intragastric pH. The role of these cells and the substances they secrete in the digestion of nutrients are described in detail in the Secretory Function section.
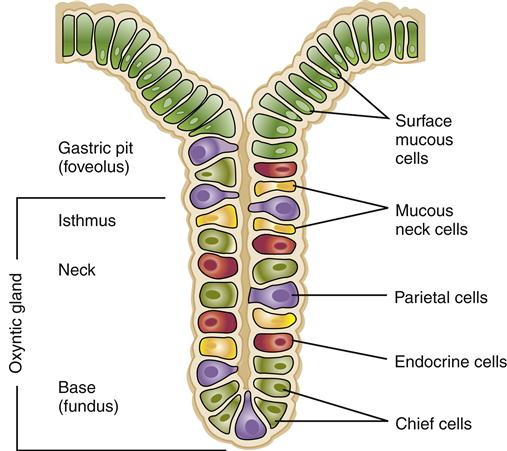
Small Intestine
The small intestine of an adult is approximately 5 to 6 m long (the longest portion of the GI tract). The first 22 cm of the small intestine is called the duodenum; the jejunum constitutes the next 2 m, and the ileum forms the remainder. The entire inner wall of the small intestine is marked by circular folds of a mucous membrane called the plicae circulares, permanent ridges that do not lose their elasticity when the intestine is distended.
On microscopic examination, the lining of the small intestine contains millions of fingerlike projections called intestinal villi (Figure 35-5). Like the circular folds just described, these villi serve to increase the surface area of the intestine for digestion and absorption of nutrients. Each villus has its own microscopic projections called microvilli, which in turn are covered by a fuzzy coat (called the brush border because of its brushlike appearance when viewed with an electron microscope) containing many digestive enzymes. The combined effect of the circular folds, villi, and microvilli is to increase the surface area of the small intestine by about 600 times, thereby creating a remarkably efficient interface for nutrient digestion and absorption. Figure 35-6 shows a microscopic section of the small intestine.
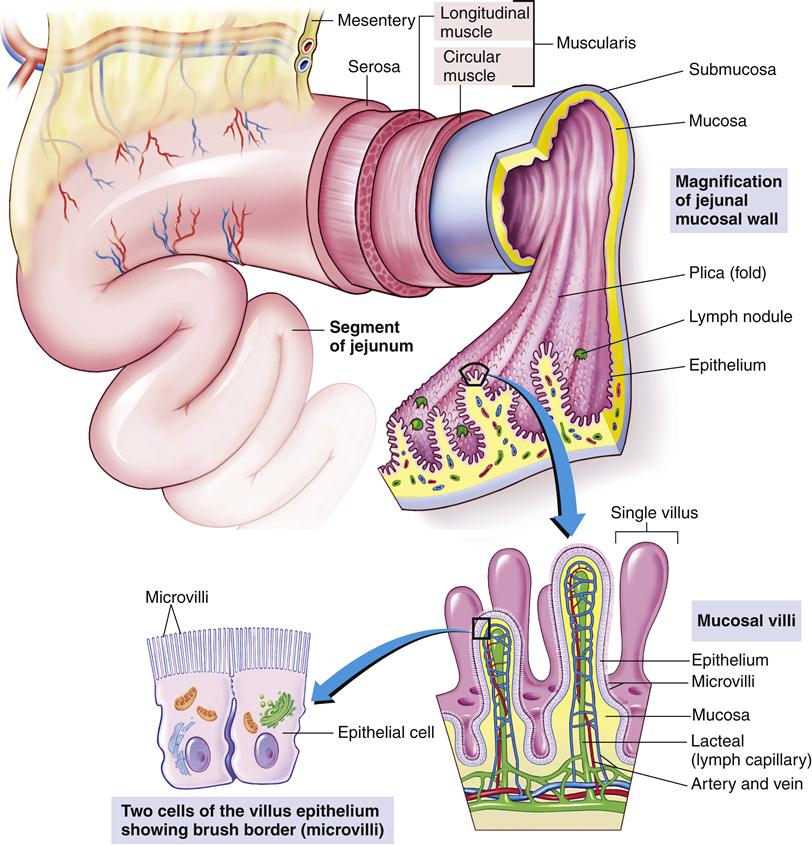
Villi contain blood capillaries and a lacteal (lymph capillary). Villi and microvilli significantly increase the surface area available for absorption. (From Patton KT, Thibodeau GA: Anatomy and physiology, ed 8, St Louis, 2013, Mosby, p 876.)
Between the villi are situated the intestinal glands, or crypts of Lieberkühn. The intestinal glands secrete about 2 L of fluid daily into the lumen of the intestine, but most of the fluid is quickly reabsorbed by the villi. Goblet cells throughout the intestinal mucosa secrete large amounts of mucus. In addition, specialized mucous glands located in the first few centimeters of the duodenum, called Brunner glands, release a thick coating of mucus to protect the mucosa from the potentially damaging effects of acidic gastric juice that may enter through the pylorus.
Although the details of the process of digestion and absorption of nutrients in the intestinal mucosa will be covered in greater detail in subsequent sections, a unique and salient feature of the villus epithelial cells is described here. Villus epithelial cells have both digestive and absorptive functions, apparently dependent on their current stage of maturation. The rapidly dividing cells at the base of the intestinal glands are responsible for secretion, but as they migrate to the villus, they mature into absorptive cells and are eventually pushed out of the villus tip. Turnover of cells in the small intestine occurs in 48 to 72 hours, one of the fastest cell turnover rates in the body. Therefore, conditions such as malnutrition or substances that interfere with cell replication or protein synthesis, such as chemotherapeutic agents, may adversely affect intestinal function.
The ileocecal valve, a sphincter between the small and large intestines, is normally closed so that the contents of the large intestine cannot reflux back into the small intestine. As peristaltic contractions move intestinal contents toward the large intestine, the ileocecal valve opens.
Large Intestine
The large intestine (Figure 35-7) is a muscular tube 1.5 m long and 6.5 cm in diameter that forms a frame around the small intestine. The portion of the large intestine from the cecum to the rectum is known as the colon. The ascending colon extends from the cecum straight up to the lower border of the liver; the transverse colon then extends across the abdomen, anterior to the small intestine. The descending colon turns downward on the left side of the abdomen, finally becoming the S-shaped sigmoid colon, which empties into the rectum. The rectum has its outlet at the anus, the opening for elimination of feces (see Figure 35-7).
The vermiform appendix, attached to the cecum, is a worm-shaped blind tube containing specialized lymphatic structures. It contains T and B lymphocytes, secretes immunoglobulin A (IgA), and contributes to gut-associated lymphoid function.9 Inflammation of the appendix, or appendicitis, is a life-threatening occurrence that can lead to peritonitis if not diagnosed and managed promptly.
The mucosa of the large intestine has no villi and does not produce digestive enzymes (Figure 35-8). The epithelial surface of the colon consists of absorptive cells that predominantly absorb water and electrolytes.10 Mucus-producing goblet cells line the glandular crypts present in the surface epithelium. Endocrine cells are also present, perhaps helping coordinate colon neurologic activity, but at present the function of hormones in the large intestine is poorly understood. The turnover time of cells in the colonic mucosa is 3 to 8 days, comparatively longer than that of cells in the small intestine.
Gastrointestinal Motility
The way in which nutrients and their eventual waste products are propelled through the GI tract is a complex and fascinating process, involving an exquisitely timed set of autoregulatory actions and responses. A summary of the characteristics of the intestinal wall, innervation of the gut, and hormonal control of GI motility will provide a basis for a description of how nutrients move through the GI tract.
Characteristics of the Intestinal Wall
A typical cross-section of the intestinal wall is depicted in Figure 35-9. From the outer surface inward are five main layers: the serosa, a longitudinal muscle layer, a circular muscle layer, the submucosa, and the mucosa. A small layer, the muscularis mucosa, is located between the mucosa and submucosa. The muscular movements of the GI tract are performed mostly by the different layers of the smooth muscle, which extends from the distal end of the esophagus through most of the large intestine. However, skeletal muscle has a key role in motility at both ends of the GI tract; motility from the mouth through the proximal portion of the esophagus at the upper end and through the external sphincter of the anus at the lower end is mediated by the action of skeletal muscle.2
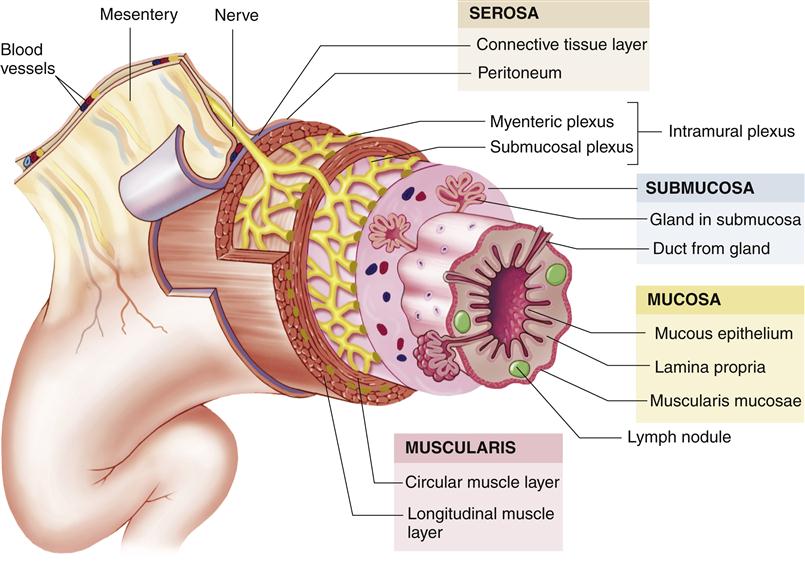
Although different areas of the GI tract specialize in function, the anatomy of the wall is similar in structure. (From Patton KT, Thibodeau GA: Anatomy & physiology, ed 8, St Louis, 2013, Mosby, p 863.)
The general characteristics of smooth muscle are covered in Chapter 5. One of the specific characteristics of smooth muscle in the gut that enables its unique function is the close proximity of these fibers to each other. In most areas of the GI tract, smooth muscle fibers are extremely close; about 12% of their membrane surfaces are actually fused with the membranes of other adjacent muscle fibers to form a nexus, or junction. This allows intracellular current to travel very easily from one muscle fiber to another. Action potentials originating in one smooth muscle fiber in the GI tract are generally propagated from fiber to fiber; therefore, the GI tract acts as a functional syncytium, where separate cells have the ability to function in a unified manner.1
Neural Control
Movement of nutrients through the GI tract is controlled by the central nervous system through its autonomic division and is modulated by numerous hormonal interactions.2,3,8 In addition, the GI system has an intrinsic nervous system of its own affecting most GI functions.9–12 The intrinsic nervous system is composed of two layers: (1) the myenteric, or Auerbach, plexus, which lies between the longitudinal and circular muscular layers; and (2) the submucosal, or Meissner, plexus, which lies in the submucosa. The myenteric plexus is largely responsible for control of GI movements; the submucosal plexus serves to control secretion and is also involved in many sensory functions, with information being received from the gut epithelium and stretch receptors in the intestinal wall. The entire intrinsic nervous system, including both the myenteric plexus and the submucosal plexus, is responsible for many reflexes that occur locally in the GI tract, such as the localized secretion of digestive juices by the submucosal glands or an increase in gut smooth muscle activity.
In general, when the myenteric plexus is stimulated, activity in the GI tract increases. This stimulation has four principal effects: (1) tonic contraction of the intestinal wall increases; (2) rhythmic contractions increase in intensity; (3) rhythmic contractions increase in rate; and (4) the velocity of conduction of excitatory waves along the intestinal wall increases. As part of the parasympathetic nervous system, these excitatory fibers of the myenteric plexus are primarily cholinergic (i.e., secrete acetylcholine), in addition to one or more other excitatory transmitter substances. However, some myenteric plexus fibers have an inhibitory effect and may secrete purine-based transmitter substances such as adenosine triphosphate (ATP).
Input from the sympathetic and parasympathetic nervous systems can strongly affect the activity of the intrinsic nervous system. In general, sympathetic stimulation decreases the activity of the intrinsic nervous system whereas parasympathetic stimulation increases its activity.
Parasympathetic Innervation
The parasympathetic supply to the GI tract is divided into cranial and sacral divisions. Cranial parasympathetic stimulation is transmitted almost entirely by the vagus nerves, which provide extensive innervation to the esophagus, stomach, pancreas, and the first half of the large intestine (with little innervation of the small intestine). The sacral parasympathetic division originates in the second, third, and fourth sacral segments of the spinal cord and innervates the distal half of the large intestine. The sigmoid, rectal, and anal regions of the large intestine are especially well supplied with parasympathetic fibers; these fibers have a key role in the defecation reflex.
Sympathetic Innervation
The sympathetic fibers that innervate the GI tract have their origin in the spinal cord between T8 and L3. After exiting the cord, the preganglionic fibers enter the sympathetic chains and then pass through these chains to various ganglia adjacent to the GI tract, such as the celiac ganglion and the mesenteric ganglia. From these locations, postganglionic fibers radiate out to all parts of the gut. These sympathetic fibers supply essentially all parts of the GI tract (in contrast to the concentration of parasympathetic innervation at locations close to the entry and exit points of the gut).2 The sympathetic nerve endings in the GI tract secrete norepinephrine, which promotes the inhibitory effect of the sympathetic nervous system on the GI tract in the following ways: Norepinephrine acts directly on smooth muscle in the GI tract to inhibit activity; in addition, norepinephrine has an inhibitory effect on the neurons of the intrinsic nervous system of the GI tract. Strong stimulation of the sympathetic nervous system can effectively stop motility in the gut and therefore block the movement of nutrients through the GI tract. Gastrointestinal sympathetic activity can also initiate vomiting through a complex sequence of events mediated by various neurotransmitters.13–15
Afferent Nerve Fibers
The GI tract is richly supplied with afferent nerve fibers arising from the gut that transmit important information about the status of the GI tract. Afferent fibers that have their cell bodies in the submucosal plexus and terminate in the myenteric plexus transmit signals in response to irritation of the gut mucosa, excessive distention, or the presence of specific chemical substances. These signals can result in excitation or, in some circumstances, inhibition of intestinal motility or secretion. Other afferent fibers with cell bodies in the dorsal root ganglia of the spinal cord or cranial nerve ganglia can transmit signals to higher levels of the central nervous system by traveling along sympathetic or parasympathetic pathways. For example, the vagus nerves contain many afferent fibers that transmit signals to the medulla; this information is then used to initiate and modulate vagal signals that control many important functions of the GI tract.
Electrical Activity of Gastrointestinal Smooth Muscle
Electrical activity is almost constantly present in the smooth muscle layers of the GI tract. Two basic types of electrical wave activity have been identified in the gut: slow waves and spikes (the latter named for the spiking appearance of these sudden increases in membrane potential).1,2 These two types of electrical wave patterns are shown in Figure 35-10. Slow-wave electrical activity represents an ongoing basic oscillation in membrane potential that occurs in the smooth muscle of the GI tract, especially in the muscle in the longitudinal layer. Normally, between 3 and 12 slow waves occur per minute, ranging from 40 to 50 millivolts (mV) in amplitude. Slow waves can be any degree of intensity and are not the “all-or-nothing” type of action potential seen in other smooth muscle fibers in the body. In contrast to these nearly continuous slow waves, spikes occur under certain circumstances. When the muscle layer in the GI tract is stimulated by being stretched or by the effects of acetylcholine or parasympathetic excitation, the intracellular resting membrane potential of the muscle fibers becomes relatively more positive. The entire potential level of the slow waves is raised—an effect called depolarization.1 As shown in Figure 35-10, when depolarization rises above a certain level (around −40 mV), sudden increases in the membrane potential, or spikes, start to appear on the peaks of the slow waves. If the resting potential rises further, spikes appear more frequently. With very strong stimulation, the spikes generally disappear because the membrane now remains entirely depolarized. Figure 35-10 also illustrates the response of smooth muscle fibers to stimulation by norepinephrine or sympathetic excitation. In this situation, the resting membrane potential is decreased, or hyperpolarized, and electrical activity is almost abolished.
Hormonal Control
The following section on secretory function of the GI tract describes in detail the role of hormones in controlling GI secretion. It is important to note that many of these same hormones are involved in controlling motility in different portions of the GI tract. Gastrin, which is secreted by specialized endocrine cells (G cells) of the stomach mucosa in response to food entry, increases stomach motility and is the primary mediator of gastric acid secretion. In addition, it promotes increased constriction of the LES, which serves to prevent reflux of stomach contents into the esophagus. Gastrin may also have a small effect in increasing motility of the small intestine and gallbladder.2,3
Cholecystokinin, which is secreted mainly by I cells of the jejunum in response to the entry of fatty substances, has an extremely strong effect on gallbladder contractility. This stimulation of gallbladder activity results in an outpouring of bile, which plays an important role in fat digestion and absorption. Cholecystokinin also stimulates pancreatic secretion, helps regulate gastric emptying and bowel motility, and induces satiety.3
Secretin, which is produced by the mucosa of the duodenum in response to the entry of acidic gastric juice from the stomach, stimulates pancreatic fluid and bicarbonate secretion, with the effect of neutralizing the acidity of intestinal contents. It also has a mild inhibitory effect on motility in most of the GI tract.2,3
Other important gastrointestinal polypeptide hormones include vasoactive intestinal polypeptide (VIP), glucagon, glucose-dependent insulinotropic polypeptide (GIP, also called gastric inhibitory polypeptide), somatostatin, motilin, leptin, and ghrelin.3 Somatostatin analogues (e.g., octreotide) have been used therapeutically for the treatment of some forms of diarrhea, endocrine tumors, parietal hemorrhage and to reduce fluid output from pancreatic fistulas.14
Ghrelin is a recently discovered peptide secreted by the stomach that increases appetite, stimulates growth hormone secretion, and produces weight gain. It helps regulate mealtime hunger and meal initiation, and as such has been the focus of intense research as a mediator for obesity, with the potential for a therapeutic antiobesity drug that would block ghrelin’s effects on the body.16–21
Movement in the Gastrointestinal Tract
Contraction of Gastrointestinal Smooth Muscle
In general, most contraction in the GI tract occurs in response to spike potentials; slow waves without superimposed spikes ordinarily do not lead to contraction. Spike potentials occurring in GI smooth muscle are analogous to action potentials in cardiac muscle and are responsible for the membrane changes that initiate contraction. As calcium enters the cell membrane and passes to the interior of the smooth muscle, it initiates a reaction between actin and myosin,1 a process described in detail in Chapter 17.
The electrical activity occurring in the smooth muscle of the gut develops into tonic contractions and rhythmic contractions, both of which occur in most types of smooth muscle. Tonic contraction is continuous, instigated by pacemaker cells that reside at the interface between the longitudinal and circular muscle layers. The intensity of tonic contraction varies with the frequency of spike potentials and determines the amount of pressure in that segment. Thus, the degree of contraction exerted by the pyloric, ileocecal, and anal sphincters serves to regulate the movement of nutrients through the GI tract.
The degree of rhythmic contraction varies in different parts of the GI tract. These differing rhythmic frequencies are dependent on the rate of slow wave activity in a particular segment and may occur at rates of 3 to 12 times per minute. These slow wave–dependent contractions are responsible for the mixing and peristaltic propulsive movements present in the GI tract.
Two types of muscular activity are involved in the digestive and absorptive functions of the GI tract: mixing movements and propulsive movements. In different portions of the GI tract these movements may serve different functions to achieve proper digestion and absorption of nutrients. For example, mixing movements in the stomach and small intestine promote digestion by mixing the digestive juices with the food that enters from the esophagus. In the small intestine and proximal segment of the large intestine, mixing movements facilitate absorption by exposing newly arrived intestinal contents into contact with absorbing surfaces. In the case of propulsion, the rate at which nutrients are propelled through the GI tract depends on the function of the different organs of the tract. For example, the passageway for nutrients from the mouth through the pharynx and esophagus is simply a conduit; essentially no digestive or absorptive function occurs there and the transit of nutrients is quite rapid. In contrast, transit from the stomach and through the small and large intestines is quite slow. This slow rate of passage allows for completion of the digestive and absorptive processes that occur in these portions of the GI tract.
Although the characteristics of mixing and propulsive movements differ in various parts of the GI tract and will be described separately in the next section, a description of the general characteristics of these movements is presented here.
Propulsive Movements
The basic propulsive movement of the GI tract is called peristalsis (Figure 35-11). Nutrients are propelled by the slow advancement of a circular constriction that squeezes the materials in front of the constricted area forward. Peristalsis is an inherent property of any smooth muscle tube that, like the intestine, is a functional syncytium. However, effective intestinal peristalsis requires the presence of an intact myenteric nerve plexus. The usual stimulus for peristalsis is distention of the intestinal walls. The entry and subsequent stretching of the intestinal wall by a bolus of food will have the effect of stimulating the gut wall 2 to 3 cm above this point, and a circular constriction will then occur and propel the food with a peristaltic movement. Although peristalsis can move in both directions in the gut, it normally moves toward the anus. It is thought that the myenteric plexus is organized in such a way that preferential transmission of signals downward occurs simultaneously with relaxation of the distal portion of the intestine below the distended stimulus point.
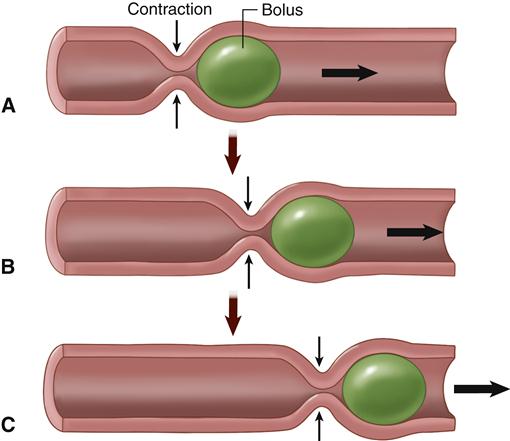
A, A ring of contraction occurs where the GI wall is stretched, pushing the bolus forward. B, The moving bolus triggers a ring of contraction in the next region, which pushes the bolus even farther along. C, The ring of contraction moves like a wave along the GI tract, pushing the bolus forward. (From Patton KT, Thibodeau GA: Anatomy & physiology, ed 8, St Louis, 2013, Mosby, p 906.)
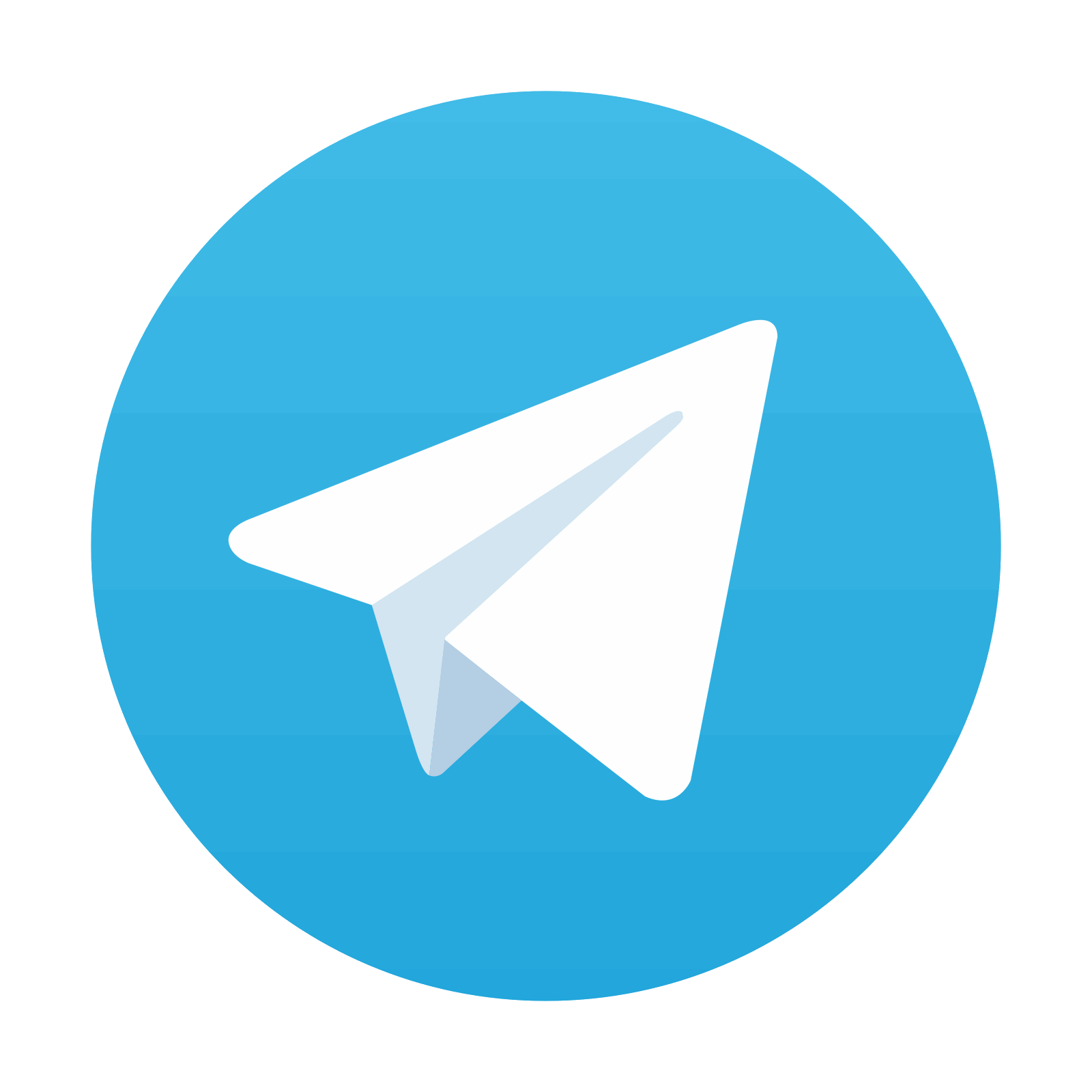
Stay updated, free articles. Join our Telegram channel

Full access? Get Clinical Tree
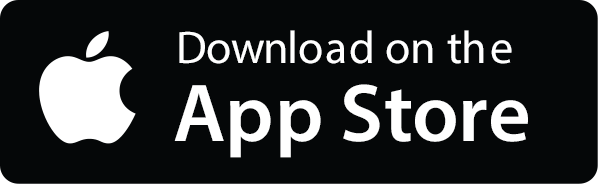
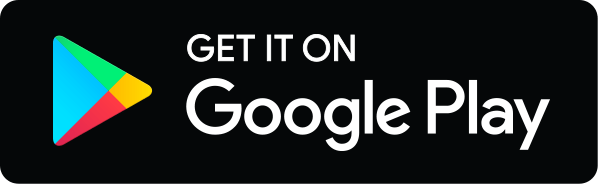