Fluid and Electrolyte Balance
INTERRELATIONSHIP OF FLUID AND ELECTROLYTE BALANCE
Several of the basic physical properties of matter discussed in Chapter 2 help explain the mechanisms of fluid and electrolyte balance. The concept of chemical bonding is a good example. The type of chemical bonds between molecules of certain chemical compounds, such as sodium chloride (NaCl), permits breakup, or dissociation, into separate particles (Na+ and Cl−). Recall that such compounds are known as electrolytes. The dissociated particles of an electrolyte are called ions and carry an electrical charge. Organic substances such as glucose, however, have a type of bond that does not permit the compound to break up, or dissociate, in solution. Such compounds are known as nonelectrolytes.
Many electrolytes and their dissociated ions are of critical importance in fluid balance. Fluid balance and electrolyte balance are so interdependent that if one deviates from normal, so does the other. A discussion of one therefore necessitates a discussion of the other.
TOTAL BODY WATER
Normal values for total body water expressed as a percentage of total body weight will vary between 45% and 75%. Differences occur because of age, fat content of the body, and gender. In newborn infants, total body water represents about 75% of body weight. This percentage then decreases rapidly during the first 10 years of life. At adolescence, adult values are reached and gender differences, which account for about a 10% variation in body fluid volumes between the sexes, appear. In young, nonobese adults, males weighing 70 kg (154 pounds) will have on average about 60% of their body weight as water (nearly 40 liters) and females about 50% (Table 32-1). Adipose, or fat, tissue contains the least amount of water of any tissue (including bone) in the body. Therefore, regardless of age, obese individuals, with their high body fat content, have less body water per kilogram of weight than slender people do. In aged individuals of either sex, body water content may decrease to 45% of total body weight. One reason for this is that old age is often accompanied by a decrease in muscle mass (65% water) and an increase in fat (20% water). In addition, with advancing age the kidneys are less able to produce concentrated urine, and sodium-conserving responses become less effective.
TABLE 32-1
Volumes of Body Fluid Compartments*
BODY FLUID | INFANT | ADULT MALE | ADULT FEMALE |
Extracellular fluid | |||
Plasma | 4 | 4 | 4 |
Interstitial fluid | 26 | 16 | 11 |
Intracellular fluid | 45 | 40 | 35 |
TOTAL | 75 | 60 | 50 |
BODY FLUID COMPARTMENTS
Functionally, the total body water can be subdivided into two major fluid compartments called the extracellular and the intracellular fluid compartments. Extracellular fluid (ECF) consists mainly of the plasma found in the blood vessels and the interstitial fluid that surrounds the cells (Table 32-2). In addition, the lymph and so-called transcellular fluid—such as cerebrospinal fluid, joint fluids, and humors of the eye—are also considered extracellular fluid. The distribution of body water by compartment is shown in Figure 32-1. Intracellular fluid (ICF) refers to the water inside the cells.
TABLE 32-2
Commonly Used Acronyms for Body Fluids and Fluid Pressures
ACRONYM | TERM | MEANING |
BCOP | Blood colloid osmotic pressure | Force that draws water into the plasma (from the presence of colloid particles in plasma) |
BHP | Blood hydrostatic pressure | Force of the blood pushing outward on blood vessel walls; blood pressure |
ECF | Extracellular fluid | Any fluid outside cells, such as interstitial fluid (IF) or blood plasma |
ICF | Intracellular fluid | Fluid inside the cell (cytosol) |
IF | Interstitial fluid | Fluid between tissue cells |
IFCOP | Interstitial fluid colloid osmotic pressure | Force that draws water out of the blood and into tissue spaces (from the presence of colloid particles in IF) |
IFHP | Interstitial fluid hydrostatic pressure | Force (pressure) of the fluid between tissue cells |
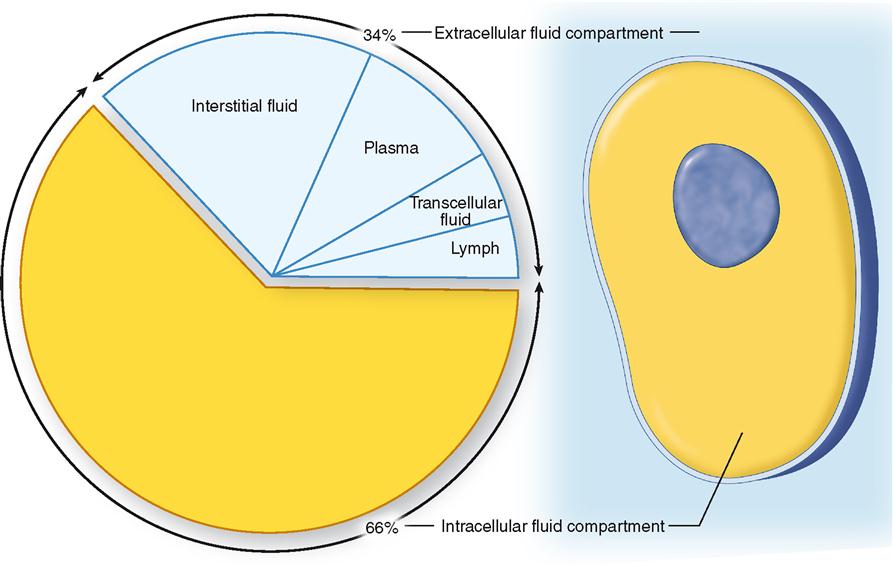
Extracellular fluid makes up the internal environment of the body. It therefore serves the dual vital functions of providing a relatively constant environment for cells and transporting substances to and from them. Intracellular fluid, on the other hand, because it is a solvent, functions to facilitate intracellular chemical reactions that maintain life. When compared according to volume, intracellular fluid is the largest (25 L), plasma the smallest (3 L), and interstitial fluid in between (12 L). Figure 32-2 illustrates the typical normal fluid volumes in a young adult male, and Table 32-1 lists volumes of the body fluid compartments for both sexes as a percentage of body weight.
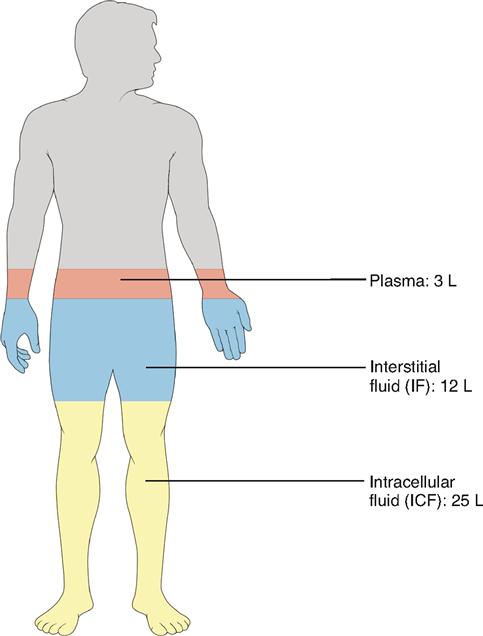
CHEMICAL CONTENT, DISTRIBUTION, AND MEASUREMENT OF ELECTROLYTES IN BODY FLUIDS
We have defined an electrolyte as a compound that will break up or dissociate into charged particles called ions when placed in solution. Sodium chloride, when dissolved in water, provides a positively charged sodium ion (Na+) and a negatively charged chloride ion (Cl−).
If two electrodes charged with a weak current are placed in an electrolyte solution, the ions will move, or migrate, in opposite directions according to their charge. Positive ions such as Na+ will be attracted to the negative electrode (cathode) and are called cations. Negative ions such as Cl− will migrate to the positive electrode (anode) and are called anions. Various anions and cations serve critical nutrient or regulatory roles in the body. Important cations include sodium (Na+), calcium (Ca++), potassium (K+), and magnesium (Mg++). Important anions include chloride (Cl−), bicarbonate (HCO3−), phosphate (HPO4=), and many proteins.
The importance of electrolytes in controlling the movement of water between the body fluid compartments is discussed in this chapter. Their role in maintaining acid-base balance is examined in Chapter 33.
Extracellular vs. Intracellular Fluids
Compared chemically, plasma and interstitial fluid (the two extracellular fluids) are almost identical. Intracellular fluid, on the other hand, shows striking differences as compared with either of the two extracellular fluids. Let us examine first the chemical structure of plasma and interstitial fluid as shown in Figure 32-3 and Table 32-3.
TABLE 32-3
Electrolyte Composition of Blood Plasma
CATIONS | ANIONS | |
142 mEq Na+ | 102 mEq Cl− | |
4 mEq K+ | 26 HCO−3 | |
5 mEq Ca++ | 17 protein | |
2 mEq Mg++ | 6 other | |
2 HPO−4 | ||
TOTAL | 153 mEq/L plasma | 153 mEq/L plasma |
Perhaps the first difference between the two extracellular fluids that you notice (see Figures 32-3 and 32-4) is that blood plasma contains a slightly larger total of electrolytes (ions) than interstitial fluids do. If you compare the two fluids, ion for ion, you will discover the most important difference between blood plasma and interstitial fluid. Look at the anions (negative ions) in these two extracellular fluids. Note that blood contains an appreciable amount of protein anions. Interstitial fluid, in contrast, contains hardly any protein anions. This is the only functionally important difference between blood and interstitial fluid. It exists because the normal capillary membrane is practically impermeable to proteins. Hence, almost all protein anions remain behind in the blood instead of filtering out into the interstitial fluid. Because proteins remain in the blood, certain other differences also exist between blood and interstitial fluid—notably, blood contains more sodium ions and fewer chloride ions than interstitial fluid does.
Extracellular fluids and intracellular fluid are more unlike than alike chemically. Chemical difference predominates between the extracellular and intracellular fluids. Chemical similarity predominates between the two extracellular fluids. Study Figures 32-3 and 32-4 and make some generalizations about the main chemical differences between the extracellular and intracellular fluids. For example: What is the most abundant cation in extracellular fluids? In intracellular fluid? What is the most abundant anion in extracellular fluids? In intracellular fluid? What about the relative concentrations of protein anions in extracellular fluids and intracellular fluid?
The reason we call attention to the chemical structure of the three body fluids is that here, as elsewhere, structure determines function. In this instance the chemical structure of the three fluids helps control water and electrolyte movement between them. Or, phrased differently, the chemical structure of body fluids, if normal, functions to maintain homeostasis of fluid distribution and, if abnormal, results in fluid imbalance. Hypervolemia (excess blood volume) is a case in point. Edema (discussed in detail on p. 1015), too, frequently stems from changes in the chemical structure of body fluids. Box 32-1 discusses therapies involving administration of fluids and electrolytes.
Before discussing mechanisms that control water and electrolyte movement between blood, interstitial fluid, and intracellular fluid, it is important to understand the units used for measuring electrolytes.
Measuring Electrolyte Reactivity
After the important electrolytes and their constituent ions in the body fluid compartments had been established, physiologists needed to measure changes in their levels to understand the mechanisms of fluid balance. To have meaning, measurement units used to report electrolyte levels must be related to actual physiological activity. In the past, only the weight of an electrolyte in a given amount of solution—its concentration—was measured. The number of milligrams per 100 ml of solution (mg%) was one of the most frequently used units of measurement. However, simply reporting the concentration of an important electrolyte such as sodium or calcium in milligrams per 100 ml of blood (mg%) gives no direct information about its chemical combining power or physiological activity in body fluids. The importance of valence and electrovalent or ionic bonding in chemical reactions was discussed in Chapter 2. The reactivity or combining power of an electrolyte depends not just on the number of molecular particles present but also on the total number of ionic charges (valence). Univalent ions such as sodium (Na+) carry only a single charge, but the divalent calcium ion (Ca++) carries two units of electrical charge.
The need for a unit of measurement more related to activity has resulted in increasing use of a more meaningful measurement yardstick—the milliequivalent (mEq). Milliequivalents measure the number of ionic charges or electrovalent bonds in a solution and therefore serve as an accurate measure of the chemical (physiological) combining power, or reactivity, of a particular electrolyte solution. The number of milliequivalents of an ion in a liter of solution (mEq/L) can be calculated from its weight in 100 ml (mg%) by using a convenient conversion formula.
Conversion of milligrams per 100 ml (mg%) to milliequivalents per liter (mEq/L):

Example: Convert 15.6 mg% K+ to mEq/L
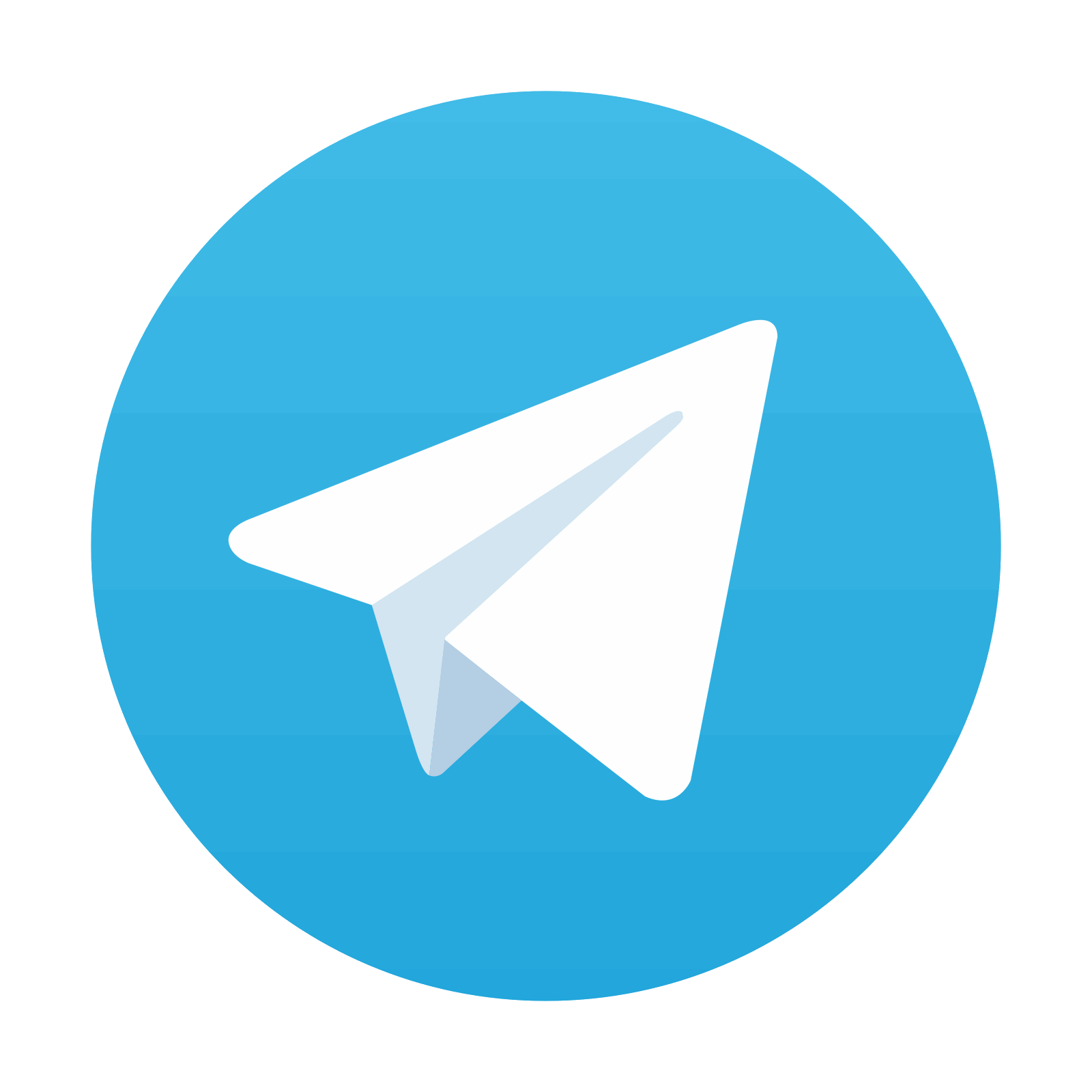
Stay updated, free articles. Join our Telegram channel

Full access? Get Clinical Tree
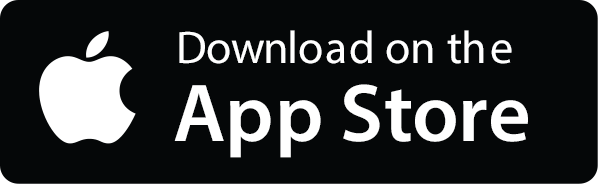
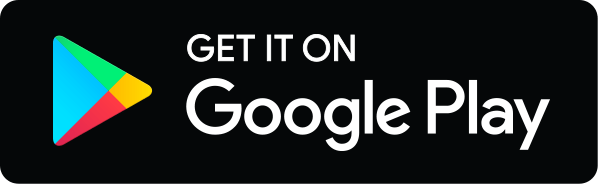
