In comparison with carbohydrate and lipid metabolism, the metabolism of amino acids is complex. We must be concerned not only with the fate of the carbon atoms of the amino acids but also with the fate of the nitrogen. During their metabolism, amino acids travel in the blood from one tissue to another. Ultimately, most of the nitrogen is converted to urea in the liver and the carbons are oxidized to CO2 and H2O by a number of tissues (Fig. 36.1).
FIGURE 36.1 Fate of amino acid carbons and nitrogen. Amino acid carbon can be used either for energy storage (glycogen, fatty acids) or for energy. Amino acid nitrogen is used for urea synthesis. One nitrogen of urea comes from NH4+, the other from aspartate.
After a meal that contains protein, amino acids released by digestion (see Chapter 35) pass from the gut through the hepatic portal vein to the liver (see Fig. 36.2A). In a normal diet containing 60 to 100 g protein, most of the amino acids are used for the synthesis of proteins in the liver and in other tissues. Excess amino acids may be converted to glucose or triacylglycerol.
FIGURE 36.2 Roles of various tissues in amino acid metabolism. A. In the fed state, amino acids released by digestion of dietary proteins travel through the hepatic portal vein to the liver, where they are used for the synthesis of proteins, particularly the blood proteins, such as serum albumin. The carbon skeletons of excess amino acids are converted to glucose or to triacylglycerols. The latter are then packaged and secreted in VLDL. The glucose produced from amino acids in the fed state is stored as glycogen or released into the blood if blood glucose levels are low. Amino acids that pass through the liver are converted to proteins in cells of other tissues. B. During fasting, amino acids are released from muscle protein. Some enter the blood directly. Others are partially oxidized and the nitrogen stored in the form of alanine and glutamine, which enter the blood. In the kidney, glutamine releases ammonia into the urine and is converted to alanine and serine. In the cells of the gut, glutamine is converted to alanine. Alanine (the major gluconeogenic amino acid) and other amino acids enter the liver, where their nitrogen is converted to urea, which is excreted in the urine, and their carbons are converted to glucose and ketone bodies, which are oxidized by various tissues for energy. RBCs, red blood cells; TCA, tricarboxylic acid; VLDL, very-low-density lipoproteins.
During fasting, muscle protein is cleaved to amino acids. Some of the amino acids are partially oxidized to produce energy (see Fig. 36.2B). Portions of these amino acids are converted to alanine and glutamine, which, along with other amino acids, are released into the blood. Glutamine is oxidized by various tissues, including the lymphocytes, gut, and kidney, which convert some of the carbons and nitrogen to alanine. Alanine and other amino acids travel to the liver, where the carbons are converted to glucose and ketone bodies and the nitrogen is converted to urea, which is excreted by the kidneys. Glucose, produced by gluconeogenesis, is subsequently oxidized to CO2 and H2O by many tissues, and ketone bodies are oxidized by tissues such as muscle and kidney.
Several enzymes are important in the process of interconverting amino acids and in removing nitrogen so that the carbon skeletons can be oxidized. These include dehydratases, transaminases, glutamate dehydrogenase, glutaminase, and deaminases.
The conversion of amino acid nitrogen to urea occurs mainly in the liver. Urea is formed in the urea cycle from NH4+, bicarbonate, and the nitrogen of aspartate (see Fig. 36.1). Initially, NH4+, bicarbonate, and adenosine triphosphate (ATP) react to produce carbamoyl phosphate, which reacts with ornithine to form citrulline. Aspartate then reacts with citrulline to form argininosuccinate, which releases fumarate, forming arginine. Finally, arginase cleaves arginine to release urea and regenerate ornithine. The cycle is regulated in a feed-forward manner, such that when amino acid degradation is occurring, the rate of the cycle is increased. The urea cycle spans two compartments in the cell; the mitochondria (where carbamoyl phosphate and citrulline are produced) and the cytoplasm (where the remainder of the cycle takes place).
THE WAITING ROOM 
Percy V. and his high school friend decided to take a Caribbean cruise, during which they sampled the cuisine of many of the islands on their itinerary. One month after their return to the United States, Percy V. complained of severe malaise, loss of appetite, nausea, vomiting, headache, and abdominal pain. He had a low-grade fever and noted a persistent and increasing pain in the area of his liver. After a few days, his urine turned the color of iced tea and his stool became a light-clay color. Soon after this, his friend noted a yellow discoloration of the whites of Percy V.’s eyes and skin. He went to see his family physician, who found his liver to be enlarged and tender. Liver function tests were ordered.
Serologic testing for viral hepatitis types B and C were nonreactive, but tests for antibodies to antigens of the hepatitis A virus (anti-HAV) in the serum were positive for the immunoglobulin M type.
A diagnosis of acute viral hepatitis type A was made, probably contracted from virus-contaminated food Percy V. had eaten while on his cruise. His physician explained that there was no specific treatment for type-A viral hepatitis but recommended symptomatic and supportive care and prevention of transmission to others by the fecal–oral route. He took acetaminophen three to four times a day for fever and headaches throughout his illness.
I. Fate of Amino Acid Nitrogen
A. Transamination Reactions
Transamination is the major process for removing nitrogen from amino acids. In most instances, the nitrogen is transferred as an amino group from the original amino acid to α-ketoglutarate, forming glutamate, whereas the original amino acid is converted to its corresponding α-keto acid (Fig. 36.3). For example, the amino acid aspartate can be transaminated to form its corresponding α-keto acid, oxaloacetate. In the process, the amino group is transferred to α-ketoglutarate, which is converted to its corresponding amino acid, glutamate.
FIGURE 36.3 Transamination. The amino group from one amino acid is transferred to another. Pairs of amino acids and their corresponding α-keto acids are involved in these reactions. α-Ketoglutarate and glutamate are usually one of the pairs. The reactions, which are readily reversible, use PLP as a cofactor. The enzymes are called transaminases or aminotransferases. A. A generalized reaction. B. The aspartate transaminase reaction. PLP, pyridoxal phosphate.
All amino acids except lysine and threonine undergo transamination reactions. The enzymes that catalyze these reactions are known as transaminases or aminotransferases. For most of these reactions, α-ketoglutarate and glutamate serve as one of the α-keto acid–amino acid pairs. Pyridoxal phosphate (PLP; derived from vitamin B6) is the required cofactor for these reactions.
Overall, in a transamination reaction, an amino group from one amino acid becomes the amino group of a second amino acid. Because these reactions are readily reversible, they can be used to remove nitrogen from amino acids or to transfer nitrogen to α-keto acids to form amino acids. Thus, they are involved both in amino acid degradation and in amino acid synthesis. The mechanism of a transamination reaction is available online, in the supplemental material.
B. Removal of Amino Acid Nitrogen as Ammonia
Cells in the body and bacteria in the gut release the nitrogen of certain amino acids as ammonia or ammonium ion (NH4+) (Fig. 36.4). Because these two forms of nitrogen can be interconverted, the terms are sometimes used interchangeably. Ammonium ion releases a proton to form ammonia by a reaction with a pKa of 9.3. Therefore, at physiologic pH, the equilibrium favors NH4+ by a factor of approximately 100/1 (see Chapter 4, the Henderson–Hasselbalch equation). However, it is important to note that NH3 is also present in the body, because this is the form that can cross cell membranes. For example, NH3 passes into the urine from kidney tubule cells and decreases the acidity of the urine by binding protons, forming NH4+. Once the NH4+ is formed, the compound can no longer freely diffuse across membranes.
FIGURE 36.4 Summary of the sources of NH4+ for the urea cycle. All of the reactions are irreversible except that of GDH. Only the dehydratase reactions, which produce NH4+ from serine and threonine, require PLP as a cofactor. The reactions that are not shown occurring in the muscle or the gut can all occur in the liver, where the NH4+ generated can be converted to urea. The purine nucleotide cycle of the brain and muscle is described further in Chapter 39. GDH, glutamate dehydrogenase; NAD, nicotinamide adenine dinucleotide; PLP, pyridoxal phosphate.
Glutamate is oxidatively deaminated by a reaction catalyzed by glutamate dehydrogenase that produces ammonium ion and α-ketoglutarate (Fig. 36.5). Either NAD+ or NADP+ can serve as the cofactor. This reaction, which occurs in the mitochondria of most cells, is readily reversible; it can incorporate ammonia into glutamate or release ammonia from glutamate. Glutamate can collect nitrogen from other amino acids as a consequence of transamination reactions and then release ammonia through the glutamate dehydrogenase reaction. This process provides one source of the ammonia that enters the urea cycle. Glutamate dehydrogenase is one of four mammalian enzymes that can “fix” ammonia into organic molecules. The other three are glutamine synthetase, carbamoyl phosphate synthetase I (CPSI), and the glycine cleavage enzyme (also known as glycine synthase).
FIGURE 36.5 Reaction catalyzed by glutamate dehydrogenase. This reaction is readily reversible and can use either NAD+ or NADP+ as a cofactor. The oxygen on α-ketoglutarate is derived from H2O. NAD, nicotinamide adenine dinucleotide.
In addition to glutamate, a number of amino acids release their nitrogen as NH4+ (see Fig. 36.4). Histidine may be directly deaminated to form NH4+ and urocanate. The deaminations of serine and threonine are dehydration reactions that require PLP and are catalyzed by serine dehydratase. Serine forms pyruvate, and threonine forms α-ketobutyrate. In both cases, NH4+ is released.
Glutamine and asparagine contain R-group amides that may be released as NH4+ by deamidation. Asparagine is deamidated by asparaginase, yielding aspartate and NH4+. Glutaminase acts on glutamine, forming glutamate and NH4+. The glutaminase reaction is particularly important in the kidney, where the ammonium ion produced is excreted directly into the urine, where it forms salts with metabolic acids, facilitating their removal in the urine.
In muscle and brain, but not in liver, the purine nucleotide cycle allows NH4+ to be released from amino acids (see Fig. 36.4). Nitrogen is collected by glutamate from other amino acids by means of transamination reactions. Glutamate then transfers its amino group to oxaloacetate to form aspartate, which supplies nitrogen to the purine nucleotide cycle (see Chapter 39). The reactions of the cycle release fumarate and NH4+. The ammonium ion formed can leave the muscle in the form of glutamine.
In summary, NH4+ that enters the urea cycle is produced in the body by deamination or deamidation of amino acids (see Fig. 36.4). A significant amount of NH4+ is also produced by bacteria that live in the lumen of the intestinal tract. This ammonium ion enters the hepatic portal vein and travels to the liver.
C. Role of Glutamate in the Metabolism of Amino Acid Nitrogen
Glutamate plays a pivotal role in the metabolism of amino acids. It is involved in both synthesis and degradation.
Glutamate provides nitrogen for amino acid synthesis (Fig. 36.6). In this process, glutamate obtains its nitrogen either from other amino acids by transamination reactions or from NH4+ by the glutamate dehydrogenase reaction. Transamination reactions then serve to transfer amino groups from glutamate to α-keto acids to produce their corresponding amino acids.
FIGURE 36.6 Role of glutamate in amino acid synthesis. Glutamate transfers nitrogen by means of transamination reactions to α-keto acids to form amino acids. This nitrogen is either obtained by glutamate from transamination of other amino acids or from NH4+ by means of the GDH reaction. GDH, glutamate dehydrogenase; PLP, pyridoxal phosphate, the active form of vitamin B6 (pyridoxine).
When amino acids are degraded and urea is formed, glutamate collects nitrogen from other amino acids by transamination reactions. Some of this nitrogen is released as ammonia by the glutamate dehydrogenase reaction, but much larger amounts of ammonia are produced from the other sources shown in Figure 36.4. NH4+ is one of the two forms in which nitrogen enters the urea cycle (Fig. 36.7).
FIGURE 36.7 Role of glutamate in urea production. Glutamate collects nitrogen from other amino acids by transamination reactions. This nitrogen can be released as NH4+ by GDH. NH4+ is also produced by other reactions (see Fig. 36.4). NH4+ provides one of the nitrogens for urea synthesis. The other nitrogen comes from aspartate and is obtained from glutamate by transamination of oxaloacetate. GDH, glutamate dehydrogenase.
The second form of nitrogen for urea synthesis is provided by aspartate (see Fig. 36.7). Glutamate can be the source of the nitrogen. Glutamate transfers its amino group to oxaloacetate, and aspartate and α-ketoglutarate are formed.
D. Role of Alanine and Glutamine in Transporting Amino Acid Nitrogen to the Liver
Protein turnover and amino acid degradation occur in all tissues; however, the urea-cycle enzymes are active primarily in the liver (the intestine expresses low levels of activity of these enzymes; see Chapter 40). Thus, a mechanism needs to be in place to transport amino acid nitrogen to the liver. Alanine and glutamine are the major carriers of nitrogen in the blood. Alanine is primarily exported by muscle. Because the muscle is metabolizing glucose through glycolysis, pyruvate is available in the muscle. The pyruvate is transaminated by glutamate to form alanine, which travels to the liver (Fig. 36.8). The glutamate is formed by transamination of an amino acid that is being degraded. Upon arriving at the liver, alanine is transaminated to pyruvate, and the nitrogen is used for urea synthesis. The pyruvate formed is used for gluconeogenesis and the glucose exported to the muscle for use as energy. This cycle of moving carbons and nitrogen between the muscle and liver is known as the glucose–alanine cycle.
FIGURE 36.8 The glucose–alanine cycle. Within the muscle, amino acid degradation leads to the transfer of nitrogens to α-KG and pyruvate. The alanine formed travels to the liver, where the carbons of alanine are used for gluconeogenesis and the alanine nitrogen is used for urea biosynthesis. This could occur during exercise, when the muscle uses blood-borne glucose (see Chapter 45). α-KG, α-ketoglutarate.
Glutamine is synthesized from glutamate by the fixation of ammonia, requiring energy (ATP) and the enzyme glutamine synthetase (Fig. 36.9), which is a cytoplasmic enzyme found in all cells. In the liver, glutamine synthetase is located in cells surrounding the portal vein. Its major role is to convert any ammonia that has escaped from urea production into glutamine, so that free ammonia does not leave the liver and enter the circulation.
FIGURE 36.9 Synthesis of glutamine in peripheral tissues and its transport to the liver. Within the liver, glutaminase converts glutamine to glutamate. Note how α-KG can accept two molecules of ammonia to form glutamine. α-KG, α-ketoglutarate; ADP, adenosine diphosphate; ATP, adenosine triphosphate; GDH, glutamate dehydrogenase; Pi, inorganic phosphate.
Under conditions of rapid amino acid degradation within a tissue, so that ammonia levels increase, the glutamate that has been formed from transamination reactions accepts another nitrogen molecule to form glutamine. The glutamine travels to the liver, kidney, or intestines, where glutaminase (see Fig. 36.9) will remove the amide nitrogen to form glutamate plus ammonia. In the kidney, the release of ammonia, and the formation of ammonium ion, serves to form salts with metabolic acids in the urine. In the intestine, the glutamine is used as a fuel (see Chapter 40). In the liver, the ammonia is used for urea biosynthesis.
II. Urea Cycle
The normal human adult is in nitrogen balance; that is, the amount of nitrogen ingested each day, mainly in the form of dietary protein, is equal to the amount of nitrogen excreted. The major nitrogenous excretory product is urea, which exits from the body in the urine. This innocuous compound, produced mainly in the liver by the urea cycle, serves as the disposal form of ammonia, which is toxic, particularly to the brain and central nervous system (CNS). Normally, little ammonia (or NH4+) is present in the blood. The concentration ranges between 30 and 60 μM. Ammonia is rapidly removed from the blood and converted to urea by the liver. Nitrogen travels in the blood mainly in amino acids, particularly alanine and glutamine. The urea cycle was proposed in 1932 by Hans Krebs and a medical student, Kurt Henseleit, based on their laboratory observations. It was originally called the Krebs–Henseleit cycle. Subsequently, Krebs used this concept of metabolic cycling to explain a second process that also bears his name, the Krebs (or tricarboxylic acid [TCA]) cycle.
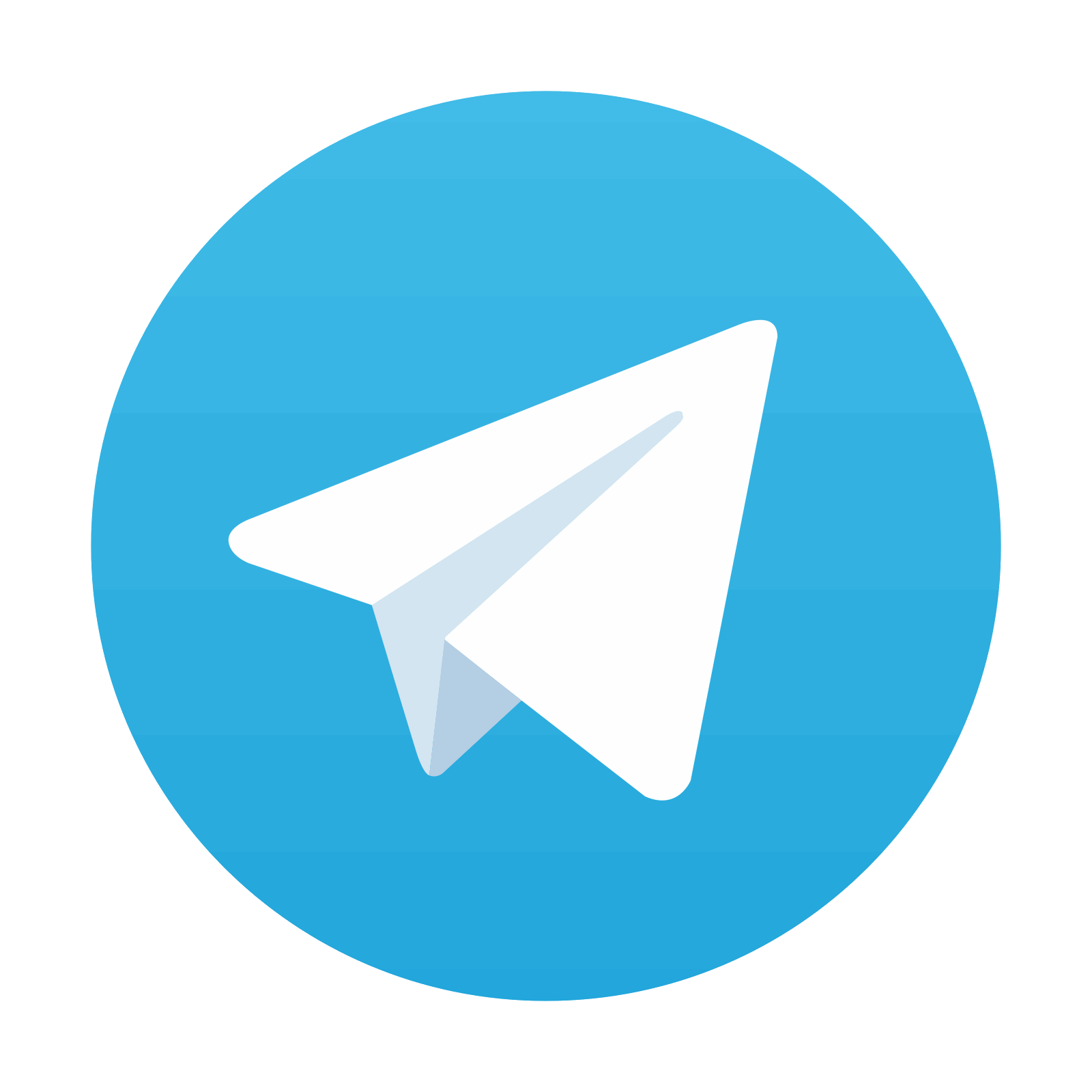
Stay updated, free articles. Join our Telegram channel

Full access? Get Clinical Tree
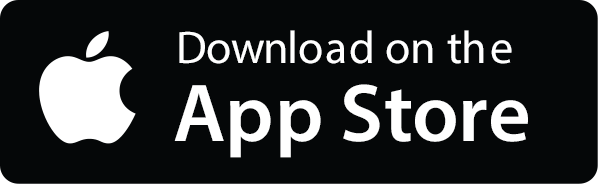
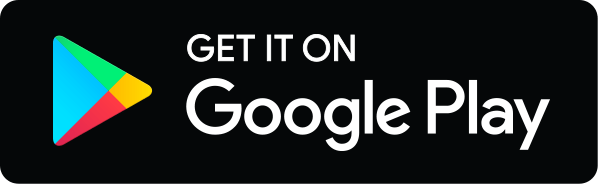