Figure 42-1. Anatomy of the esophagus.
Unlike the remainder of the gastrointestinal tract, the esophagus does not have a serosal layer and its strength is derived from its mucosa. The thoracic esophagus is covered only by parietal pleura, making this portion the weakest and the most common site of perforation in Boerhaave syndrome, usually on the left side where there is a lack of support from adjacent structures.
The azygos vein is closely related to the right of the esophagus as it ascends from the abdomen and then arches from its paraspinal position over esophagus and the right main bronchus to enter the superior vena cava.
The thoracic duct ascends behind and to the right of the distal thoracic esophagus between the azygos vein and aorta. At approximately the level of T5, it passes alongside the aorta and ascends on the left side of the esophagus to enter behind the junction of the left internal jugular and subclavian veins. Due to the possibility of disrupting the thoracic duct during its course across the mediastinum during an esophagectomy, ligation of the duct is generally performed low in the chest where it comes through the aortic hiatus.
Figure 42-2. Posterior view of cervical esophagus.
Abdominal Esophagus
The abdominal portion of the esophagus is approximately 3 to 6 cm long and consists of the abdominal portion of the LES. It begins as the esophagus passes through the diaphragmatic hiatus and is surrounded by the phrenoesophageal membrane, a fibroelastic ligament that arises from the subdiaphragmatic fascia as a continuation of the transversalis fascia lining the abdomen (Fig. 42-3). The upper leaf of the membrane attaches in a circumferential fashion around the esophagus about 1 to 2 cm above the level of the hiatus. The lower leaf of the phrenoesophageal membrane blends with the serosa of the stomach, and its end is marked anteriorly by a prominent fat pad, which corresponds approximately with the gastroesophageal junction. The lower esophageal sphincter is a zone of high pressure 3 to 4 cm long at the lower end of the esophagus1 and does not correspond to any visible macroscopic anatomic landmark either on the external surface of the esophagus, nor in the endoscopic appearance of the mucosa. Its function is derived from the microscopic architecture of the muscle fibers. The esophageal hiatus is surrounded by the right and left crura, which together form a sling of diaphragmatic skeletal muscle around the esophagus that originates from tendinous bands attached to the anterolateral surface of the first lumbar vertebra (Fig. 42-4). The relative contribution of the right and left crura to this sling is variable. Posterior to the esophagus, the crura are united by a tendinous arch, the median arcuate ligament, which lies just anterior to the aorta.
Figure 42-3. Anatomy of the esophageal hiatus and relationship to the phrenoesophageal membrane.
Figure 42-4. Inferior view of diaphragm and anatomy of the esophageal hiatus.
Structure of the Esophageal Wall and Mucosa
The esophagus is composed of three primary layers – mucosa, submucosa, and muscularis propria. The mucosal layer is normally composed of nonkeratinized stratified squamous epithelium, as opposed to the stomach where columnar epithelium is present. In the distal aspect of the esophagus within the lower esophageal sphincter, repeated exposure of the squamous epithelium to gastric contents can result in metaplasia of esophageal squamous mucosa to a columnar epithelium called cardiac mucosa. This nomenclature was originally used to describe what was mistakenly thought to be the proximal stomach or cardia, but there is now convincing evidence that this is in fact metaplastic esophageal mucosa.2 With further injury and exposure to refluxed gastric juice such as bile acids, cardiac mucosa may further transform into intestinal metaplasia or Barrett esophagus, characterized by the presence of goblet cells within cardiac mucosa. Alternatively, cardiac mucosa can also differentiate further to acquire parietal cells and is known as oxyntocardiac mucosa.3
A critical layer within the mucosa for determining the biologic behavior of a superficial cancer is the muscularis mucosa. This thin, poorly developed layer lies below the basement membrane and above the submucosa. It is often duplicated in patients with Barrett esophagus, and can lead to confusion during pathologic evaluation of the depth of tumor invasion in endoscopic resection (ER) specimens.4 The significance of the muscularis mucosa is that tumors confined to this layer rarely are associated with lymph node metastases, but once tumors penetrate through this layer into the submucosa the frequency of lymph node metastases increases substantially.
The submucosa is characterized by a rich lymphatic plexus that extends throughout the esophagus. Lymphatic collecting branches arise from within the submucosa and pierce the muscularis propria to communicate with regional lymph nodes and the thoracic duct outside of the esophagus. This network of lymphatics allow for significant longitudinal spread of esophageal cancers and a high rate of skip metastases. Clinically, tumor invasion beyond the muscularis mucosa into the submucosa is significant due to this lymphatic system, with the rate of regional lymph node metastases increasing from <5% with intramucosal tumors to 20% to 50% for submucosal tumors.5 Finally, the submucosa of the esophagus also harbors mucous glands that drain into the lumen. These glands are helpful for determining the true extent of the esophagus when metaplasia of the mucosa occurs.
The muscularis propria is composed of an inner circular layer and an outer longitudinal layer. The muscles are striated in the upper portion and transition to completely smooth muscle near the upper third of the esophagus. The longitudinal muscle layer courses down in an elongated spiral pattern, turning approximately 90 degrees as it descends to the stomach. The circular muscle layer is thicker than the longitudinal layer, which also takes on an elliptical or spiral orientation (Fig. 42-5). This arrangement of muscle is responsible for the wormlike drive of peristalsis.
Blood Supply, Lymphatics, and Innervation
The cervical portion of the esophagus receives its main blood supply from the inferior thyroid artery. The thoracic portion receives blood from the bronchial and esophageal arteries. Seventy-five percent of individuals have one right-sided and two left-sided bronchial arteries, and usually two esophageal branches arise directly from the aorta. There are rarely aortic branches directly to the esophagus in the lower half of the esophagus in the chest, allowing the surgeon to perform an en bloc dissection of all of the periesophageal tissue directly off the plane of the aortic adventitia from the right to the left pleural spaces. The blood supply of the abdominal portion of the esophagus comes from the ascending branch of the left gastric artery and from the right and left inferior phrenic arteries (Fig. 42-6). After the vessels have entered the muscular wall of the esophagus, branching occurs at right angles to provide an extensive longitudinal vascular plexus. The rich blood supply provided by this vascular plexus allows mobilization of the esophagus from the stomach to the aortic arch without causing ischemic injury.6
Figure 42-5. Endoscopic view of esophageal muscle layers during a peroral endoscopic myotomy (POEM) for achalasia. The endoscopic knife is lifting and cutting the circumferential circular muscle fibers, leaving the longitudinal muscle fibers intact.
Figure 42-6. Arterial anatomy of the esophagus.
The capillaries of the esophagus drain into a submucosal and periesophageal venous plexus, from which the esophageal veins originate. In the cervical region, the esophageal veins empty into the inferior thyroid vein; in the thoracic region, they empty into the bronchial, azygos, or hemiazygos veins; and in the abdominal region, they empty into the coronary vein (Fig. 42-7).
The lymphatic channels are located almost exclusively below the muscularis mucosa in the submucosa of the esophagus, constituting a dense and interconnected plexus with more lymph vessels than blood capillaries (Fig. 42-8). Lymph flow in the submucosal plexus runs in a longitudinal direction, and after the injection of a contrast medium, the longitudinal spread is six times that of the transverse spread. In the upper two-thirds of the esophagus, the lymphatic flow is mostly cephalad; in the lower third, it is mostly caudal. In the thoracic portion of the esophagus, the submucosal lymph plexus extends over a long distance in a longitudinal direction before penetrating the muscle layer to enter lymph vessels in the adventitia. As a consequence of this nonsegmental lymph drainage, the lymphatic spread of tumor cells can extend for a considerable distance superiorly and inferiorly within the submucosal lymphatics before the cells pass through lymphatic channels in the muscularis and on into the regional lymph nodes. There is a high rate of skip metastases in esophageal cancer due to this arrangement. By contrast, the cervical esophagus has a more segmental lymph drainage into the regional lymph nodes, and as a result, tumors in this portion of the esophagus have less submucosal extension.
Lymph from the cervical esophagus drains into the paratracheal and deep cervical lymph nodes, whereas lymph from the upper thoracic esophagus flows mainly into the paratracheal lymph nodes. The lymph from the lower thoracic esophagus drains into the subcarinal and inferior pulmonary nodes. Lymph from the distal thoracic and abdominal portion of the esophagus drains into the parahiatal and perigastric nodes.7
Figure 42-7. Venous anatomy of the esophagus.
The parasympathetic innervation of the pharynx and esophagus is provided mainly by cranial nerve X or the vagus nerves. The constrictor muscles of the pharynx receive branches from the pharyngeal plexus, which is located on the posterior lateral surface of the middle constrictor muscle and is formed by pharyngeal branches of the vagus nerve, with a small contribution from cranial nerves IX and XI. The cricopharyngeal sphincter and the cervical portion of the esophagus receive branches from both the right and left recurrent laryngeal nerves originating from the vagus nerves (Fig. 42-9). Damage to these recurrent nerves interferes not only with the movement of the vocal cords but also with the function of the cricopharyngeal sphincter and the motility of the cervical esophagus, predisposing the patient to pulmonary aspiration on swallowing. The upper thoracic esophagus receives innervation from the left recurrent laryngeal nerve and both vagus nerves. As the right and left vagus nerves descend into the mediastinum, they join the outer surface of the esophagus. The esophageal plexus, which is formed by the branches of the right and left vagus nerves and thoracic sympathetic chain, lies on the anterior and posterior walls of the esophagus and innervates the lower thoracic portion.8 The branches of the plexus coalesce into the left (anterior) and right (posterior) vagal trunks.
Afferent visceral sensory fibers from the esophagus end without synapse in the first four segments of the thoracic spinal cord by a combination of sympathetic and vagal pathways. These pathways are also occupied by afferent visceral sensory fibers from the heart, which explains the similarity of symptoms in esophageal and cardiac diseases.
PHYSIOLOGY
To comprehend the mechanics of alimentation, it is useful to visualize the gullet as a series of pumps and valves. In the pharyngeal segment, the tongue and pharyngeal muscle function as pumps, whereas the soft palate, the epiglottis, and the cricopharyngeus serve as the valves that regulate flow. In the esophageal segment, the esophageal body functions as the pump to propel the food bolus, whereas the lower esophageal sphincter serves as a valve to allow transport into the stomach and to prevent the flow of gastric contents back into the esophagus.
The Swallowing Mechanism
Swallowing can be started at will, or it can be reflexively elicited by the stimulation of the anterior and posterior tonsillar pillars or the posterior lateral walls of the hypopharynx. The afferent sensory nerves of the pharynx are the glossopharyngeal nerves and the superior laryngeal branches of the vagal nerves. Once it is aroused by stimuli entering through these nerves, the swallowing center in the medulla coordinates the complete act of swallowing by discharging impulses through cranial nerves V, VII, X, XI, and XII and the motor neurons of C1 through C3. Discharges through these nerves always occur in a specific pattern and last for approximately 0.5 second. Little is known about the swallowing center except that it can trigger swallowing after a variety of different inputs. Once it is triggered, the swallow response is always a rigidly ordered pattern of outflow neurogenic impulses.
The act of alimentation requires the passage of food and drink from the mouth into the stomach. Food is taken into the mouth in a variety of bite sizes, after which it is broken up by the teeth, mixed with saliva, and lubricated. When food is ready for swallowing, the tongue, acting as a pump, moves the bolus into the posterior oropharynx and forces it into the hypopharynx (Fig. 42-10). Concomitantly with the posterior movement of the tongue, the soft palate is elevated, thereby closing the passage between the oropharynx and nasopharynx. With the initiation of the swallow, the hyoid moves superiorly and anteriorly, thereby elevating the larynx and enlarging the retropharyngeal space. At the same time, the epiglottis covers the laryngeal inlet to prevent aspiration.
Figure 42-8. Lymphatic anatomy of the esophagus.
During swallowing, the pressure in the hypopharynx rises abruptly to 60 mm Hg as a result of the backward movement of the tongue and contraction of the posterior pharyngeal constrictors. A sizable pressure difference develops between the hypopharyngeal pressure and the subatmospheric midesophageal or intrathoracic pressure (Fig. 42-11). This pressure gradient speeds the movement of food from the hypopharynx into the esophagus when the cricopharyngeus or UES relaxes. The bolus is both propelled by the peristaltic contraction of the posterior pharyngeal constrictors and sucked into the thoracic esophagus by this pressure gradient.
Critical to receiving the bolus is the compliance of the cervical esophageal muscle and the timing and degree of relaxation of the UES. Abnormalities of compliance and UES opening can result in pharyngeal dysphagia. During the transfer of the bolus from the mouth into the esophagus, the UES is mechanically pulled open. Elevation of the larynx by muscles attached to the hyoid bone pulls the UES open at the same time that relaxation of the UES is occurring. The active relaxation of the UES is caused by a reduction in the tone of the tonic constriction of the cricopharyngeus muscle, and it is dependent on a neurologically mediated reflex. This is an all-or-nothing event; partial relaxation does not normally occur. The UES closes within 0.5 second of the initiation of the swallow, with a postrelaxation contraction pressure that is approximately twice the resting pressure of 30 mm Hg. The postrelaxation contraction continues down the esophagus as a peristaltic wave (Fig. 42-12). The high closing pressure and the initiation of the peristaltic wave prevent reflux of the bolus from the esophagus into the pharynx. After completion of the swallow, the pressure of the UES returns to its normal resting pressure.
The pharyngeal activity in swallowing initiates the esophageal phase of swallowing. Because of the helical arrangement of its circular muscles, the body of the esophagus functions as a worm-drive propulsive pump, and it is responsible for transmitting a bolus of food into the stomach. With the act of swallowing, the longitudinal muscle of the esophageal body shortens, thus enlarging the lumen to accept the bolus (the “on response”), after which the circular smooth muscle contraction forms the peristaltic wave (the “off response”). During the esophageal phase of swallowing, the bolus is moved into the stomach over a gradient of 12 mm Hg (i.e., from a negative intrathoracic pressure environment of −6 mm Hg to a positive intra-abdominal pressure environment of +6 mm Hg). Effective and coordinated smooth muscle function in the lower two-thirds of the esophageal body is important to allow this movement to occur.
Figure 42-9. Relationship of the esophagus to the vagus nerves and their branches.
The peristaltic wave generates an occlusive pressure that varies from 30 to 120 mm Hg. The wave rises to a peak in 1 second, remains at the peak for about 0.5 second, and then subsides in about 1.5 seconds. The whole course of the rise and fall of an occlusive contraction may occupy one point in the esophagus for 3 to 5 seconds.9,10 The peak of the primary peristaltic contraction moves down the esophagus at a rate of 2 to 4 cm per second and reaches the distal esophagus about 9 seconds after swallowing starts. The lower esophageal sphincter relaxes at the initiation of the peristaltic wave and remains open until the peristaltic wave passes through the body and into the sphincter muscle, and this is followed by a distinctive postrelaxation contraction of the lower esophageal sphincter. A consecutive swallow after 20 seconds produces a similar primary peristaltic wave; however, if repetitive swallowing occurs sooner, the esophagus becomes unresponsive (deglutitive inhibition).
Figure 42-10. Overview of the act of swallowing.
Figure 42-11. Pressure profile of the esophagus in the neck, chest, and abdomen. (From Waters PF, DeMeester TR. Foregut motor disorders and their surgical management. Med Clin North Am 1981;65:1237, with permission.)
Figure 42-12. Overview of esophageal body peristalsis. (From Waters PF, DeMeester TR. Foregut motor disorders and their surgical management. Med Clin North Am 1981;65:1237, with permission.)
To be effective, peristaltic contractions must be of sufficient amplitude to occlude the esophageal lumen and sufficiently organized in a peristaltic waveform to propel a bolus toward the stomach. Low-amplitude contractions that do not occlude the lumen merely indent a semisolid bolus rather than propel it, and simultaneous contractions throughout the body of the esophagus result in splitting the bolus or propelling it orally which can be observed on a barium esophagram as bolus segmentation or cephalic escape.
Clinically, defects in peristalsis occur in three broad categories, depending on which major feature is the most impaired. First, there is a neural abnormality that results in the defective organization of the peristaltic wave; this is recognized by the presence of simultaneous contractions with a loss of the peristaltic sequence and results in typical primary motility disorders (e.g., diffuse esophageal spasm). The second category defect is evident when there is a reduction of the amplitude of the contraction but the peristaltic sequence remains; this is usually due to muscle damage and the formation of fibrous tissue within the muscle. Examples include end-stage gastroesophageal reflux disease (GERD) and connective tissue disorders such as scleroderma. The third category defect results from altered anatomy of the esophageal body. A loss in the efficiency of the peristaltic sequence can result when the esophagus is not anchored distally, as occurs with a large paraesophageal hernia (PEH); which can result in the appearance of an accordion esophagus on a barium swallow esophagram with ineffective clearance of barium.
Lower Esophageal Sphincter
The LES represents the barrier that confines the gastric juice to the stomach and protects the acid-sensitive squamous esophageal mucosa from injury by refluxed gastric juice. As is true for any valve, failure of the LES can occur in two completely opposite ways, which lead to two distinct clinical disease entities. Regardless of the type of LES failure, the secondary effects are produced proximally in the esophagus. Failure of the LES to relax or to open appropriately leads to the inability of the esophagus to propel food into the stomach, esophageal distention, and the condition known as achalasia. On the other hand, failure of the LES to remain closed leads to an increased exposure of the squamous epithelium to gastric juice and the condition known as GERD.
The LES has no anatomic landmarks and cannot be seen endoscopically; it is identified on manometry as a rise in pressure over gastric baseline pressure. This high-pressure zone is normally present except in two situations: (1) after a swallow, when it is momentarily dissipated or relaxes to allow passage of food into the stomach; and (2) during a belch, when it allows gas to be vented from a distended fundus. The common denominator for virtually all episodes of gastroesophageal reflux is the loss of this normal high-pressure zone or barrier. When the barrier is absent, resistance to the flow of gastric juice from an environment of higher pressure (the stomach) to an environment of lower pressure (the esophagus) is lost. In early GERD, this is usually caused by a transient loss of the barrier. In advanced GERD, there is usually a permanent loss of the barrier.11
There are three characteristics of the LES or high-pressure zone that maintain its function as a barrier to intragastric and intra-abdominal pressure challenges. Two of these characteristics – the overall length and pressure of the LES – work together and depend on each other to provide resistance to the flow of gastric juice from the stomach into the esophagus.12 The shorter the overall length, the higher the pressure must be for the LES to maintain sufficient resistance to remain competent (Fig. 42-13). Consequently, the effect of a normal LES pressure can be nullified by a short overall LES length, and the effect of a normal overall LES length can be nullified by a low LES pressure. A fundamental principle for surgeons to understand is that the length of the barrier or LES is critical to its function.
Figure 42-13. Relationship of the length and pressure of the lower esophageal sphincter in maintaining competence of the gastroesophageal barrier.
Foreshortening of LES length occurs naturally with gastric filling as the terminal esophagus is “taken up” by the expanding fundus (Fig. 42-14);13 this is similar to the shortening of the neck of a balloon as it is inflated. With excessive gastric distention (e.g., with overeating), the length of the LES shortens to a critical point at which it gives way, the pressure drops precipitously, and reflux occurs (Fig. 42-15).14 If the length of the LES is permanently shortened, then further shortening caused by the normal gastric distention with normal-volume meals results in postprandial reflux. In this situation, competency of the barrier is an ever-constant clinical problem. The observation that gastric distention results in shortening of the LES down to a critical length so that the pressure dissipates, the lumen opens, and reflux occurs provides a mechanical explanation for transient LES relaxations without invoking a neuromuscular reflex. If only the LES pressure and not its length is measured (e.g., with a Dent sleeve), the event appears as a spontaneous relaxation of LES pressure.15 In reality, it is the progressive shortening of the LES rather than the transient LES relaxations that results in the loss of LES pressure.
Variations in the anatomy of the cardia, from a normal acute angle of His to an abnormal dome architecture of a sliding hiatal hernia, influence the ease with which the sphincter is shortened by gastric distention. A hernia can result from the pulsion force of abdominal pressure on the esophageal hiatus or from the traction produced by inflammatory fibrosis of the esophageal body. The resulting alteration in the geometry of the cardia places the sphincter at a mechanical disadvantage in maintaining its length with progressive degrees of gastric distention. Greater gastric distention is necessary to open the barrier in patients with an intact angle of His than in those with a hiatal hernia.16 The reason is that the dome or funnel shape of a hiatal hernia allows the wall tension forces that pull open the barrier with gastric distention to be more effectively applied to the gastroesophageal junction,17 and it accounts for the common association of a hiatal hernia with GERD. Kahrilas et al.18 demonstrated this mechanical disadvantage by studying the effect of intragastric air infusion on the number of transient LES relaxations or “shortenings” per hour. Patients with hiatal hernias had significantly more transient LES relaxations per hour than did control subjects without hernias. The reduction in length became significant 20 to 30 minutes after the beginning of air infusion and occurred in a distal to cephalad direction before a loss of LES pressure was observed.
Figure 42-14. Effect of increasing gastric volume on the shortening of the lower esophageal sphincter length. As the stomach expands, the gastric body takes up the inferior aspect of the sphincter causing its length to shorten. (From Mason RJ, Lund RJ, DeMeester TR, et al. Nissen fundoplication prevents shortening of the sphincter during gastric distention. Arch Surg 1997;132:719–726, with permission.)
Figure 42-15. Representation of the loss of competence of the lower esophageal sphincter with shortening of its length. As a critical length is reached, there is a precipitous drop in sphincter pressure reflecting loss of competence. (From Pettersson GB, Bombeck CT, Nyhus LM. The lower esophageal sphincter: mechanisms of opening and closure. Surgery 1980;88:307–314, with permission.)
The third characteristic of the LES high-pressure zone is its position. A portion of the overall length of the high-pressure zone is normally exposed to the positive intra-abdominal pressure environment and is commonly referred to as the abdominal length of the LES.19 During periods of increased intra-abdominal pressure, the resistance of the LES would easily be overcome if its position were such that abdominal pressure was unable to be applied equally to the LES and the stomach.20–22 This is analogous to sucking on a soft soda straw immersed in a bottle of liquid; the positive hydrostatic pressure of the fluid and the negative pressure inside the straw from sucking cause the straw to collapse instead of allowing the liquid to flow up the straw in the direction of the negative pressure. When the abdominal length of the LES is inadequate, increases in intra-abdominal pressure will be applied to the stomach but not the LES thereby encouraging reflux to occur. Studies have shown that the critical length of abdominal LES is 1 cm, below which almost no LES pressure will be sufficient to maintain competency of the sphincter.15
In the fasting state deficits in LES pressure, overall length, or abdominal length will lead to an increased likelihood of sphincter incompetence. An LES defective in all three parameters is particularly likely to be associated with increased reflux of gastric juice into the esophagus. This reflux can result in inflammatory injury to the mucosa and ultimately to the muscularis propria of the esophageal body, thereby causing a reduced contraction amplitude of the esophageal body and interrupted or dropped peristaltic sequences. Continued reflux can lead to progressive loss of effective esophageal clearance, protracted esophageal exposure to the refluxed material and ultimately further organ injury (Fig. 42-16).23,24
Causes and Consequences of the Failure of the Gastroesophageal Barrier
Early GERD is initiated by increased transient losses of the barrier as a result of gastric overdistention from excessive air and food ingestion.11,25 The tension vectors produced by gastric wall distention pull on the gastroesophageal junction, which results in the terminal esophagus being “taken up” into the stretched fundus, thereby reducing the length of the LES. With overeating, a critical length is reached (usually about 1 to 2 cm) at which the sphincter gives way; its pressure drops precipitously, and reflux occurs (Fig. 42-15). If the swallowed air is vented, gastric distention is reduced, the length of the LES is restored, and competency returns until subsequent distention again shortens it and further reflux occurs. Aerophagia is common in patients with GERD because they swallow their saliva more frequently to neutralize the acidic gastric juice that is refluxed into the esophagus.26 Together, the actions of overeating and air swallowing result in the common complaint of postprandial bloating, repetitive belching, and heartburn in patients with early GERD. The high prevalence of the disease in the Western world is thought to be a result of the eating habits of Western society.27 Gastric distention from overeating, along with delayed gastric emptying resulting from the increased ingestion of fatty foods, leads to prolonged periods of postprandial gastric distention with shortening of the LES and repetitive transient loss of the barrier. Surgical correction with a fundoplication prevents the shortening of the barrier with progressive degrees of gastric distention by diverting the forces that pull on the gastroesophageal junction.14
Figure 42-16. Overview of the progressive nature of GERD and the effect of the lower esophageal sphincter and esophageal body on reflux in the upright and supine positions.
In advanced GERD, permanent loss of sphincter length occurs from inflammatory injury that extends from the mucosa into the muscular layers of the LES. Fletcher et al.28 showed that in the fasting state, there is a persistent region of high acidity in the area of the gastroesophageal junction and that this region of acidity migrates 2 cm proximally after meals.29 This migration occurs from distention of the stomach with eating and pulling apart of the distal high-pressure zone or LES, thus allowing the area of high acidity to move proximal to the squamocolumnar junction. This proximal movement exposes the distal esophageal squamous mucosa to acid and results in the formation of cardiac mucosa. Cardiac mucosa is an acquired mucosa that replaces chronically injured squamous mucosa in the terminal esophagus.30 The inflammatory process can extend into the muscular layer of the LES, thereby resulting in muscle cell injury with permanent shortening of the high-pressure zone or LES and a concomitant reduction in the amplitude of the high-pressure zone or barrier pressure.30–32 A defective barrier is recognized when the length or pressure of the LES measured during the fasting state is below the 2.5 percentile of normal.33
For clinicians, the finding of a permanently defective LES has several implications. First, symptoms in patients with a defective LES can be difficult to manage, and mucosal damage may persist with medical therapy.34 Surgery is usually required to achieve consistent long-term symptom relief in these patients to restore a gastroesophageal barrier and interrupt the natural history of the disease. It has been shown repeatedly that a laparoscopic Nissen fundoplication can consistently restore the length and pressure of the LES to normal parameters.35 Newer techniques of sphincter augmentation such as with a magnetic bracelet is an alternative means of restoring the LES that is gaining in popularity.36 Second, a permanently defective LES is commonly associated with reduced contractility and abnormal wave progression of the esophageal body.37 For this reason, careful evaluation of the esophageal body is critical in the evaluation for antireflux surgery for potential tailoring of the operation with a partial fundoplication. Third, a permanently defective LES and the loss of effective esophageal clearance can lead to mucosal injury such as erosive esophagitis or Barrett metaplasia, repetitive regurgitation and aspiration events and ultimately pulmonary fibrosis. Without reestablishing a barrier, chronic use of acid suppression therapy may simply mask the symptoms due to modification of the pH; however, in the setting of a structurally defective LES, reflux will continue unabated.38
EVALUATION OF ESOPHAGEAL FUNCTION
A thorough understanding of the patient’s underlying anatomic and functional deficits is fundamental to the successful treatment of esophageal disease. The diagnostic tests that are employed to evaluate the esophagus are those used to visualize structural abnormalities, to detect functional abnormalities, and to measure esophageal exposure to gastric juice. At the authors’ center a panel of four diagnostic tests are used routinely in the evaluation of known or suspected reflux disease: barium esophagram, upper endoscopy, high-resolution esophageal manometry, and ambulatory pH monitoring.
Radiographic Evaluation
Radiographic assessment of the anatomy and function of the esophagus and stomach is one of the most important aspects of the esophageal evaluation, provided the surgeon has a working knowledge of esophageal physiology. Classically, the barium esophagram has been described as a road map for the esophagus. The first diagnostic test in patients with suspected esophageal disease should be a barium swallow that includes a full assessment of the stomach and the duodenum.39 Video recording of the study greatly aids in the evaluation by providing the surgeon with a real-time visualization of bolus transport and the size and reducibility of the hiatal hernia. The study also provides anatomic information, such as the presence of obstructing lesions and structural abnormalities of the foregut.
The pharynx and the UES are evaluated in the upright position, allowing assessment of the timing and coordination of the events of pharyngeal transit.40 This includes oropharyngeal bolus transport, pharyngeal contraction, opening of the pharyngoesophageal segment, and degree of airway protection during swallowing. It readily identifies a diverticulum, stasis of the contrast medium in the valleculae, cricopharyngeal bar, or narrowing of the pharyngoesophageal segment. These are anatomic manifestations of neuromuscular disease and result from the loss of muscle compliance from the deinnervation of the skeletal muscle of the pharynx and the cervical esophagus.41
The assessment of bolus transport on video esophagography often adds to or complements the information obtained by esophageal manometry. Esophageal clearance is optimally assessed by observing several individual swallows of barium with the patient in both the upright and supine positions; the study can be performed with both liquid and solid bolus material. During normal swallowing, a primary peristaltic wave is generated that completely strips the bolus out of the esophagus and into the stomach. Residual material rarely stimulates a secondary peristaltic wave; rather, an additional pharyngeal swallow is usually required.
The protocol developed and utilized at the authors’ institution has previously been reported.42 Normal subjects in the prone position should be able to clear at least three of five 10-mL liquid barium boluses with one swallow and have only one episode of proximal escape or distal retention of a barium bolus with the five swallows. Normal subjects can also clear a solid barium bolus with four or fewer swallows in the upright position. Motility disorders with disorganized or simultaneous esophageal contraction give a segmented appearance to the barium column. This can often give a beading or corkscrew appearance to the barium within the esophagus. In patients with dysphagia, the use of a barium-impregnated marshmallow, piece of bread, or hamburger can identify an esophageal transport disturbance that is not evident on the liquid barium study. A 13-mm barium tablet is another useful adjunct that can be used to determine how well solid material can clear the esophagus, and identify areas of clinically significant narrowing since solid food dysphagia typically is present with luminal narrowing below 13 mm.
A hiatal hernia is present in a high percentage of patients with gastroesophageal reflux.43 These are best demonstrated with the patient in the prone position; the increased intra-abdominal pressure produced in this position promotes displacement of the hernia above the diaphragm. The hiatal hernia is an important component of the underlying pathophysiology of reflux. A large (>5 cm) or irreducible hiatal hernia may be associated with chronic reflux and esophageal foreshortening. The diagnosis of reflux disease is not accurately made on video esophagram. Spontaneous reflux to the level of the thoracic inlet seems to correlate with a positive pH test, but evoked reflux and spontaneous reflux into the distal esophagus are not. Moreover, failure to observe reflux during a video esophagram does not indicate the absence of disease.
A full-column technique with distention of the esophageal wall can discern extrinsic compression of the esophagus, and a fully distended esophagogastric region is necessary to identify narrowing from a Schatzki ring, stricture, or obstructing lesion. Mucosal relief or double-contrast films can be obtained to enhance the detection of small neoplasms, esophagitis, and varices. Assessment of the stomach and duodenum during the barium study is helpful for the evaluation of the patient with esophageal symptoms (ESs). A gastric or duodenal ulcer, a neoplasm, or poor gastroduodenal transit can mimic many of the symptoms that are suggestive of an esophageal disorder.
Endoscopic Examination
Endoscopic evaluation of the esophagus is essentially the physical examination of the foregut. It is a critical part of the assessment of a patient with esophageal disease and is indicated in essentially every patient who is being evaluated for GERD. A barium study obtained before esophagoscopy is helpful to the endoscopist by directing attention to locations of subtle change and alerting the examiner to such potential danger spots as a cervical vertebral osteophyte, an esophageal diverticulum, a deeply penetrating ulcer, or a carcinoma. Regardless of the radiologist’s interpretation of an abnormal finding, each structural abnormality of the esophagus should be examined visually with an endoscope.
During every endoscopic examination, the locations of three specific landmarks are routinely obtained relative to the front incisors: the squamocolumnar junction, gastroesophageal junction, and the diaphragmatic crura. The crura are usually evident by having the patient sniff. The gastroesophageal junction is the location at which the gastric rugal folds meet the tubular esophagus; it is normally aligned with the squamocolumnar junction. The squamocolumnar junction is the location at which the velvet and darker rose-colored columnar epithelium changes to the lighter squamous epithelium. When this junction is not clear, narrow band imaging (NBI), a feature that is standard on all modern endoscopic systems, is extremely helpful in distinguishing columnar from squamous mucosa. Particular effort should be made to detect any tongues, islands, or a circumferential segment of columnar-lined esophagus (CLE) in the distal esophagus.
When erosive esophagitis is found, an objective grading system should be utilized to communicate the severity of the findings. Currently, the Los Angeles classification is the most commonly utilized system.44 Grade A is defined as one or more mucosal break ≤5 mm that does not extend between the tops of mucosal folds. Grade B is defined as one or more mucosal break >5 mm that does not extend between the tops of the mucosal folds. Grade C is defined as one or more mucosal break that is continuous between the tops of two or more mucosal folds but involves <75% of the circumference. Grade D is defined as one or more mucosal break that involves ≥75% of the circumference.
Barrett esophagus is a pathologic condition in which the tubular esophagus is lined with columnar epithelium as opposed to the normal squamous epithelium. It is suspected at endoscopy when there has been separation of the squamocolumnar junction from the gastroesophageal junction. When columnar metaplasia is observed, it must be biopsied to confirm the presence of intestinal metaplasia in order to make the diagnosis of Barrett esophagus; the finding of cardiac metaplasia without goblet cells does not meet this criteria in the United States. On histologic examination, it appears as columnar mucosa with goblet cells and is called intestinal metaplasia. Early metaplasia is often manifest as an irregular or eccentric squamocolumnar junction. Routine four-quadrant biopsies with jumbo forceps are necessary every 2 cm of columnar metaplasia. In addition, the likelihood of identifying goblet cells is greatest at the cephalad portion of a columnar segment, especially at the squamocolumnar junction.45 Barrett esophagus is susceptible to ulceration, bleeding, stricture formation, and malignant degeneration. The earliest histologic sign of malignant degeneration is high-grade dysplasia (HGD) or intramucosal adenocarcinoma. These dysplastic changes have a patchy distribution, so a minimum of four biopsy specimens every 2 cm should be taken from the Barrett mucosa–lined portion of the esophagus. A study of intramucosal adenocarcinomas arising in Barrett esophagus showed that most of the tumors arose in the distal Barrett segment, closer to the stomach.46
Abnormalities of the cardia or gastroesophageal junction can be visualized by retroflexion of the endoscope and provide a complementary assessment regarding the competency of the gastroesophageal barrier. Hill et al. have graded the appearance of the gastroesophageal valve from I to IV according to the degree of unfolding or deterioration of the normal architecture (Fig. 42-17).47 This commonly used grading system has allowed endoscopists to maintain uniformity in their reporting. The Hill grade correlates well with the competence of the gastroesophageal barrier, with increasing acid exposure prevalent in patients with worsening Hill grade.
A hiatal hernia is diagnosed by observing separation of the gastroesophageal junction from the crura. By definition, a hiatal hernia is present when at least 2 cm of the top of the rugal folds has migrated above the pinch of the diaphragmatic crura. A prominent sliding hiatal hernia is frequently associated with GERD. When a hernia is observed, particular care is taken to exclude Cameron ulcers or gastritis within the herniated stomach.
As the endoscope is slowly withdrawn, the esophagus is again examined, and biopsy samples are taken. The location of the cricopharyngeus is identified, and the larynx and vocal cords are visualized. Acid reflux may result in inflammation of the larynx. Vocal cord movement should also be recorded, both as a reference for subsequent surgery and as an assessment of the patient’s ability to protect the airway.
Esophageal Manometry
Fundamental to the evaluation of a patient with benign esophageal disease is the assessment of esophageal contractility, coordination, and sphincter function. Manometry is indicated whenever an abnormality of the esophagus is suggested by the symptoms of dysphagia, odynophagia, chest pain, heartburn, and regurgitation. It is particularly necessary to confirm the diagnosis of specific primary esophageal motility disorders, such as achalasia, diffuse esophageal spasm, nutcracker esophagus, or hypertensive LES. It can also identify ineffective esophageal body motility as a result of GERD or systemic diseases such as scleroderma, dermatomyositis, polymyositis, or mixed connective tissue disorders. In patients with symptomatic GERD, esophageal manometry can identify a mechanically defective LES and evaluate the adequacy of the esophageal body contraction amplitudes and waveform. Finally, manometry is mandatory for accurate placement of an ambulatory pH monitor in relationship to the upper border of the LES.
High-resolution manometry (HRM) has now become standard technology over the past several years and represents an improvement in methodology that leads to a more detailed data collection and simpler data interpretation, especially with regard to the esophageal body. The concept behind this technology is that by vastly increasing the number of sensors and reducing the space between the sensors, it can provide representation of the entire pressure profile along the esophagus from the pharynx to the proximal stomach without the need to reposition or to pull back the catheter as in conventional manometry.
The most commonly used HRM system is a solid-state manometric assembly with 36 circumferential sensors spaced at 1-cm intervals and are available from different manufacturers. These transducers detect the pressure over a length of 25 mm in each of 12 radially dispersed sectors. The recorded pressure at each sector is then averaged, making each of the 36 sensors a circumferential pressure detector with the extended frequency response characteristic of solid-state manometric systems and free of the hydrostatic influence characteristic of water-perfused systems. The increased number of circumferential pressure sensors results in a vastly greater amount of data and detail. Interpretation of the data has been simplified by a sophisticated topographic plotting algorithm that converts the traditional linear waveform tracings into an esophageal pressure topography or Clouse plot (Fig. 42-18). Sphincter characteristics and esophageal motor function are represented by isocontour manometric plots, eliminating the need for the tedious analysis of the increased waveform data that are generated by the 36 sensors.
Figure 42-17. Endoscopic grading of the gastroesophageal junction during the retroflex view. (From Oberg S, Peters JH, DeMeester TR, et al. Endoscopic grading of the gastroesophageal valve in patients with symptoms of gastroesophageal reflux disease (GERD). Surg Endoscopy 1999;13(12):1184–1188, with permission.)
Figure 42-18. High-resolution manometry with line tracings and converted pressure topography or Clouse plot view. (From Pandalfino JE, Roman S. High-resolution manometry: an atlas of esophageal motility disorders and findings of GERD using esophageal pressure topography. Thor Surg Clin 2011;21:465–475, with permission.)
The manometry procedure is performed by passing the catheter into the stomach and esophagus to measure contraction pressures and waveform in the esophageal body and the sphincters’ resting pressure and response to swallowing. The lubricated catheter is passed through the nostril and into the esophagus. The catheter is advanced until a portion of the sensors are in the stomach. In patients who have a dilated or tortuous esophagus such as suspected achalasia or a large PEH, it is recommended that the catheter be passed endoscopically to ensure that it is properly positioned into the stomach and not coiled in the esophagus. The pressure topogram is assessed to ensure that the catheter is completely traversing the UES and the LES. Unlike with traditional manometry, a HRM study can be performed with 10 swallows without the need to repeatedly move the catheter back and forth for different portions of the examination. The patient is then asked to refrain from swallowing for 30 seconds with quiet breathing, which allows the capture of a landmark frame for assessment of the resting UES and LES. The patient is positioned supine with the head elevated and is asked to swallow 5 mL aliquots of water separated by a minimum of 30 seconds to prevent deglutitive inhibition. A complete manometric study includes the assessment of the structural characteristics of the LES, the degree of LES relaxation, the esophageal body coordination, contraction amplitude and waveform, and the UES function.
Lower Esophageal Sphincter
The resting LES is assessed during the landmark frame (Fig. 42-19). The lower (distal) border of the LES is the point at which the resting pressure rises above the gastric baseline; the upper border is the point at which sphincter pressure reaches the esophageal baseline. The respiratory inversion point is identified when the positive excursions that occur with breathing in the abdominal environment change to negative deflections in the thoracic environment. The respiratory inversion point is the functional division between the abdomen and thorax. The resting pressure of the LES is the pressure above gastric baseline measured during midrespiration at the respiratory inversion point. The overall length of the sphincter is the distance from the distal border to the proximal border. The abdominal length is the distance from the distal border to the respiratory inversion point and represents the portion of the LES that is subject to fluctuations in intra-abdominal pressure. The measurements for each of these components from each transducer are expressed as an average during the landmark frame, which extends for 30 seconds.
Figure 42-19. High-resolution esophageal manometry showing the landmark frame. The upper and lower esophageal sphincters are observed at the top and bottom of the frame, respectively. Resting pressure and length measurements of the sphincters can be determined in this portion of the study while the patient is refraining from any swallowing. (From Pandalfino JE, Roman S. High-resolution manometry: an atlas of esophageal motility disorders and findings of GERD using esophageal pressure topography. Thor Surg Clin 2011;21:465–475, with permission.)
A structurally defective sphincter is identified by one that has low resting pressure or short length. Based on conventional manometry in normal volunteers, the following characteristics have traditionally been used to identify a structurally defective LES: (1) an average LES pressure of less than 6 mm Hg, (2) an average abdominal length of less than 1 cm, and (3) an average overall length of less than 2 cm. Based on normative data, these values are below the 2.5 percentile. A defect in one or even two components of the LES may be compensated by good esophageal body function, but when all three components are defective, excessive esophageal acid exposure is inevitable. With the HRM technique, normal overall LES length should be 2.7 to 4.8 cm and mean basal resting pressure of the LES should be 13 to 43 mm Hg.
It should be noted that while HRM has revolutionized the assessment of the esophageal body due to improved resolution of contraction waves, the assessment of the LES has been less optimal. This is primarily due to the nature of the HRM catheter, which functions as a sleeve manometry catheter and results in circumferential averaging of pressure data. For this reason, length assessment of the LES and UES is believed to be less detailed than that obtained with conventional manometry with a manually pulled through catheter.48 It is believed that the newly developed 3D HRM catheter will allow more precise or detailed evaluation of the LES structure, but clinical experience is necessary to fully evaluate its additive value.
Lower Esophageal Sphincter Relaxation
The LES pressure normally drops to gastric baseline immediately after initiation of a swallow, and remains open until the oncoming peristaltic wave reaches the stomach (Fig. 42-18). A characteristic postrelaxation contraction of the LES is subsequently observed as the LES closes with passage of the bolus. Using HRM, relaxation is assessed during a 4-second segment of the time between initiation of the swallow and when the peristaltic wave reaches the LES. This 4-second window is often captured in a noncontiguous manner during the swallow to eliminate the effect of respiratory variation from the crura and is termed the integrated relaxation pressure (IRP). An IRP >15 mm Hg is considered elevated and may result in clinically significant outflow resistance through the LES.
Esophageal Body Motility
The esophageal body is evaluated by assessing contraction amplitude, duration, slope, velocity, and morphology (i.e., single, double, or triple peaked). A physiologic gap in contraction or proximal peristaltic break typically occurs in the upper one-third of the esophagus, and corresponds to the transition zone between the striated muscle above and smooth muscle below. Pathologic breaks are those that are >2 cm long or if a break occurs in the distal esophagus. HRM has had its greatest improvement in the assessment of esophageal body function. It is important to allow 30 seconds between swallows to prevent deglutitive inhibition of the body, a physiologic dampening or absence of peristalsis with repetitive swallowing.
Figure 42-20. High-resolution esophageal manometry during a swallow. The initiation of swallowing is reflected by transient relaxation of the UES followed by a peristaltic pressure wave progressing down the esophageal body. Note the LES relaxes at the initiation of swallowing. A characteristic peristaltic break is observed in the upper third of the esophageal body at the transition zone between the striated and smooth muscle. (From Pandalfino JE, Roman S. High-resolution manometry: an atlas of esophageal motility disorders and findings of GERD using esophageal pressure topography. Thor Surg Clin 2011;21:465–475, with permission.)
Further improvement in assessing esophageal body function with HRM has been the use of the distal contractile integral (DCI) which gives a global assessment of esophageal contractile function. This is an integrated summation of the contractile pressures generated between the proximal peristaltic break and the upper border of the LES and is calculated as the product of amplitude (mm Hg) × duration (seconds) × length (cm), with normal values ranging from 500 to 4,300 (Fig. 42-20). Based on conventional manometry, it was believed that a minimum of distal contraction amplitude of >20 mm Hg 5 cm above the LES was necessary to overcome the resistance of an antireflux procedure. With HRM it is currently believed that a DCI >500 mm Hg*sec*cm is necessary to minimize the possibility of postoperative dysphagia; however, clinical validation of this cutoff has not been performed.
Upper Esophageal Sphincter
The position, length, and resting pressure of the UES and its relaxation with swallowing are assessed with a technique similar to that used for the LES. The key features to be assessed are the adequacy of pharyngeal contraction and the timing and extent of UES relaxation. An indirect measure of UES stiffness or loss of compliance is the intrabolus pressure, which appears as a pressure rise or shoulder on the upstroke of the pharyngeal contraction.
High-Resolution Impedance
Even with the addition of high-resolution topography, manometric data often does not correlate with the effective passage of the swallowed bolus. To overcome this shortcoming, the barium video esophagram has been used to complement manometry; however, one drawback is that these tests cannot be performed simultaneously. Not infrequently, a discrepancy may exist between these studies with a normal video esophagram but poor esophageal body manometry characteristics, or vice versa. Currently, the authors are utilizing combined HRM with high-resolution impedance to gain additional information on bolus transport.
Ambulatory pH Monitoring
The development of ambulatory pH monitoring by DeMeester and Johnson49 was a major advance in the unraveling of the pathophysiology of GERD. All previous tests had relied on the identification of reflux by a provocative maneuver, which had little relevance to the patient’s daily activities. The 24-hour pH monitoring test allowed objective assessment of esophageal exposure to gastric juice in a patient in a continuous setting. It is considered the gold standard for the diagnosis of GERD because it has the highest sensitivity and specificity of all tests currently available. It is indicated in any patient with symptoms suggestive of GERD, unless the symptoms are trivial or permanently abolished by a short course of acid suppression therapy. The need for continued acid suppression should stimulate objective study. A 24-hour pH monitoring study is especially important in patients who are being considered for antireflux surgery. Atypical presentations of GERD are also a common indication; they include such symptoms as noncardiac chest pain (i.e., pain despite a normal cardiac evaluation) and respiratory symptoms (RSs) such as shortness of breath, cough, nocturnal wheezing, and chronic hoarseness. In such patients, 24-hour pH monitoring allows confirmation of the diagnosis of GERD and can relate the occurrence of the symptoms to an episode of reflux.
To perform the standard pH test, a thin catheter containing a pH electrode is passed transnasally into the esophagus and placed 5 cm above the upper border of the LES, a position that has been previously determined by manometry. Different probes are available, but bipolar glass electrodes are preferred for their greater reliability and their elimination of the need for an external reference electrode. The electrode is connected to an external portable digital storage device that is kept at the patient’s side, and pH values are continuously recorded at 6-second intervals for 24 hours (i.e., a complete circadian cycle). Precalibration and postcalibration of the system to pH levels of 1 and 7 is important to exclude electrode drift. A gastric “dipstick” maneuver is performed prior to securing the location of the catheter to confirm acid in the stomach and that the patient does not have atrophic gastritis. The patient is then instructed to carry out normal daily activities but to avoid strenuous exertion. He or she is asked to remain in the upright position while awake during the day, lying down supine only at night while sleeping, and to ingest two meals at the usual time. The diet is standardized only by its absence of food and beverages with a pH value of less than 5.0 and greater than 6.0. The patient notes in a diary the times of meals, retiring for sleep, and rising the following morning as well as the presence and duration of any symptoms. At the authors’ institution, patients are also asked to consume a challenge meal consisting of a hamburger, French fries, and a milk shake. It has been found that a refluxogenic meal can often uncover early acid reflux disease that would not ordinarily be detected during a typical 24-hour period.50 Figure 42-21 shows typical 24-hour pH tracings from a healthy subject and from a patient with GERD. Medications such as H2 blockers and prokinetics should be discontinued for 48 hours before the testing begins. Proton pump inhibitors (PPIs) (e.g., omeprazole) should be stopped for 2 weeks before pH monitoring because of their long-lasting action.
It is important to emphasize that 24-hour esophageal pH monitoring should not be considered a test for reflux; rather, it is a measurement of the esophageal exposure to gastric juice. The measurement is expressed as the percentage of time that the esophageal pH was below 4 during the 24-hour period. Just measuring the percentage of time that the pH is less than 4, although concise, does not reflect how the exposure has occurred; for example, it may have occurred in a few long or several short reflux episodes. Consequently, two other assessments are necessary: (1) the frequency of the reflux episodes and (2) their duration. For this reason, esophageal exposure to gastric juice is best assessed by the following measurements:
Figure 42-21. Dual-probe 24-hour pH tracing of patient. The top tracing is of the pH sensor located below the upper esophageal sphincter and the bottom tracing is of the pH sensor located 5 cm above the top of the lower esophageal sphincter. Episodes of pH <4 are considered acid events. In this example the patient has abnormal acid exposure in the distal esophagus with several reflux events extending into the proximal esophagus.
The cumulative time that the esophageal pH is below 4 expressed as the percentage of the total, upright, and supine monitored times;
The frequency of reflux episodes, when the pH drops below 4, expressed as the number of episodes per 24 hours;
The number of episodes during which the pH remained below 4 for longer than 5 minutes per 24 hours; and
The time in minutes of the longest recorded reflux episode, the longest time the pH consistently remained below 4.
Normal values for these six components of the 24-hour record were derived from 50 asymptomatic control subjects. The upper limits of normal were established at the 95th percentile.51 If the values of symptomatic patients are outside of the 95th percentile of normal subjects, they are considered to be abnormal for the component measured. There is a uniformity of normal values for these six components as reported by centers throughout the world. The normal values for the six components obtained from 50 healthy volunteers are shown in Table 42-1. A composite scoring system was developed by DeMeester and Johnson that integrates the different components of the pH record into a single measurement of esophageal acid exposure. This composite score is calculated from the six parameters with use of their standard deviations as weighing factors.51
Advances in technology have made pH testing more comfortable for the patient with the development of a catheter-free miniaturized pH electrode. The Bravo system (Medtronic, Minneapolis, MN) allows the transnasal or transoral deployment of a small capsule that attaches to the esophageal mucosa and transmitting pH data by radiotelemetry to a pager-sized receiver, thus eliminating the need for an unpleasant transnasal catheter. It may also provide a more accurate physiologic picture by allowing patients to perform their normal daily activities without the social and behavioral restrictions imposed by the catheter. Another major advantage of the Bravo capsule is the ability to record for prolonged periods, and routine monitoring of 48 hours is now performed with improved diagnostic capabilities.52 New normal thresholds have been defined and validated for the 48-hour Bravo pH test, with the normal composite score for the first 24 hours being 14.0, the second 24 hours being 14.0, and the combined 48-hour score being 16.0.51
Table 42-1 Normal Manometric Values of the Distal Esophageal Sphincter in 50 Subjects
In patients with symptoms of chronic cough, hoarseness, or pulmonary aspiration, placement of an additional pH electrode in the proximal part of the esophagus or pharynx can be helpful.53 Such dual-probe catheters are particularly helpful in determining if gastroesophageal reflux events extend proximally, which could give indirect evidence of acid exposure into pharyngeal area and subsequent irritation. If the accumulated acid exposure in the proximal esophagus is greater than 1% or the number of reflux episodes is more than 24 (particularly if there is a temporal relationship between the reflux episodes and the onset of the symptoms), reflux can be documented and assumed to be the cause of the patient’s RSs.53 A composite score has been developed for proximal pH monitoring, analogous to that developed for the distal probe, with the normal threshold composite score being 16.4.52
Additional testing is sometimes necessary if the standard methods of assessing esophageal function fail to yield conclusive results, particularly when investigating the relationship of acid reflux and extraesophageal symptoms. A novel pharyngeal pH system has been developed that allows a pH probe to be positioned directly into the pharynx just below the uvula (Restech pH probe, Respiratory Technology Corp, San Diego, CA, USA).53 This probe utilizes a novel antimony sensor that allows measurement of pH within the humidified air of that environment without drying out and creating artifacts. Early clinical experience with this technique indicates that it is a useful adjunct diagnostic test in patients suspected of having laryngopharyngeal reflux (LPR).
Testing for reflux that is nonacidic or weakly acidic can be gained by multichannel intraluminal esophageal impedance monitoring. This type of measurement determines the resistance to the flow of current through a given medium (impedance). The impedance to current changes as the composition of the medium in which the current is traveling changes (i.e., air, liquids, or solids). Coupled with a pH probe, it can differentiate acid reflux from nonacid reflux, which may be particularly useful in patients who remain symptomatic on acid suppression therapy.
Lastly, the assessment of gastric function can be important in many patients with ESs. Disorders of gastric emptying frequently can contribute to or be confused with esophageal disease, especially GERD. Nuclear medicine radioisotope with solid food matter such as scrambled eggs has been utilized for this purpose, and is indicated in any patient being evaluated for GERD who also has a history of nausea, early satiety, and vomiting. Such information may be invaluable to prevent performing an antireflux operation in the setting of gastroparesis. The most reliable results are obtained with the use of a 4-hour study rather than one that just determines a half-time of emptying.
CONCLUSIONS
Although seemingly simple, the anatomy and physiology of the esophagus is complex. Understanding the anatomic relationships is critical to enable safe surgery on the esophagus. Functional studies allow identification of abnormalities in the upper or lower esophageal sphincter as well as the esophageal body, and pH testing can determine the presence of increased exposure of the esophagus to refluxed gastric juice. An understanding of the relevant pathophysiology is critical to allow functional restoration of LES competence in patients with reflux disease, or to reduce the LES outflow resistance in patients with achalasia. While complex, restoration of esophageal function can bring tremendous improvements in quality of life and social satisfaction for patients, and is gratifying for esophageal surgeons.
GASTROESOPHAGEAL REFLUX DISEASE
Definition and Epidemiology
GERD is a very common disease, but developing an accurate definition of GERD is surprisingly difficult. In 2004, a group of experts came together in Montreal and concluded that GERD can be best defined as “a condition which develops when the reflux of stomach contents causes troublesome symptoms and/or complications.”54 Population-based studies have reported that one-third of Western populations experience the symptoms of GERD at least once a month, with 4% to 7% of the population experiencing daily symptoms.55,56 Its prevalence varies considerably around the globe and is highest in North America, Australia, and Western Europe and lowest in Africa and Asia.57 It is also likely that both the prevalence and severity of GERD are increasing in many parts of the world. Time trend analyses have shown that the prevalence of GERD symptoms has increased progressively in most longitudinal studies, including those from the United States, Singapore, and China (Fig. 42-22).58 Further, recent data reported by the Agency for Healthcare Research and Quality (AHRQ) indicate a marked increase (103%) in hospitalizations for treating disorders caused by GERD; a 216% increase in hospitalization of patients who, in addition to the ailment for which they were admitted, have milder forms of GERD; and a 39% increase in admission for GERD with severe symptoms including anemia, weight loss, and vomiting.59 These data suggest that the current therapeutic approach to GERD may be inadequate.
Figure 42-22. Time trends for the prevalence of weekly heartburn from 1980 to 2005. (Reproduced with permission from El-Serag H. Time trends of gastroesophageal reflux disease: a systematic review. Clin Gastroenterol Hepatol 2007;5:17–26.)
Most patients with mild symptoms self-medicate with over-the-counter antacids or antisecretory agents, whereas those with more severe and persistent symptoms seek out medical attention. In contrast to duodenal ulcer disease, where the prevalence has markedly decreased, the prevalence and severity of GERD seem to be increasing (Fig. 42-23).60 The diagnosis of a CLE is also increasing at a rapid rate, and deaths from end-stage benign esophageal disease are on an upward trend.61 These changes have occurred despite dramatic improvements in the efficacy of treatment options.
Two epidemiologic trends may be contributing to the increasing prevalence and severity of GERD over the past several decades. Population-based studies have shown that GERD is positively associated with obesity and negatively associated with gastric colonization with Helicobacter pylori. Over the past 20 to 30 years, the former has increased and the latter has decreased markedly in most Western countries. The relationship between GERD and body mass index (BMI) has been evaluated in a number of well-designed clinical studies. The frequency, duration, and severity of reflux symptoms were studied in 10,500 women of the Nurses’ Health Study and a dose-dependent relationship between increasing BMI frequency of GERD symptoms was identified.62 Compared to normal-weight women (BMI 20 to 22.4), underweight women (BMI <20) were one-third less likely and overweight women (BMI 25 to 27.4) two times more likely to have frequent GERD symptoms. Obese women (BMI >30) had a nearly three times higher risk of frequent GERD symptoms. Recent meta-analyses confirm these findings, with studies from the United States demonstrating an association between increasing BMI and the presence of GERD.63 High-resolution motility studies have shown a significant correlation with BMI and both intragastric pressure and gastroesophageal pressure gradients, providing a physiologic explanation for the BMI–GERD association.64 These studies suggest that obese subjects are more likely to have esophagogastric junction disruption and abnormal pressure gradients favoring the development of reflux. Finally, the risk of Barrett esophagus has been correlated with the presence of central obesity. Measures of central obesity, including waist circumference and waist-to-hip ratios, were associated with both short- and long-segment Barrett esophagus, with a 4.1 higher odds ratio of long-segment Barrett in patients with a high waist-to-hip ratio.65
Figure 42-23. Trends in hospitalization for duodenal ulcer and gastroesophageal reflux disease from the 1970s to 1990s in U.S. veterans. (Reproduced with permission from El-Serag HB, Sonnenberg A. Opposing time trends of peptic ulcer and reflux disease. Gut 1998;43:327–333.)
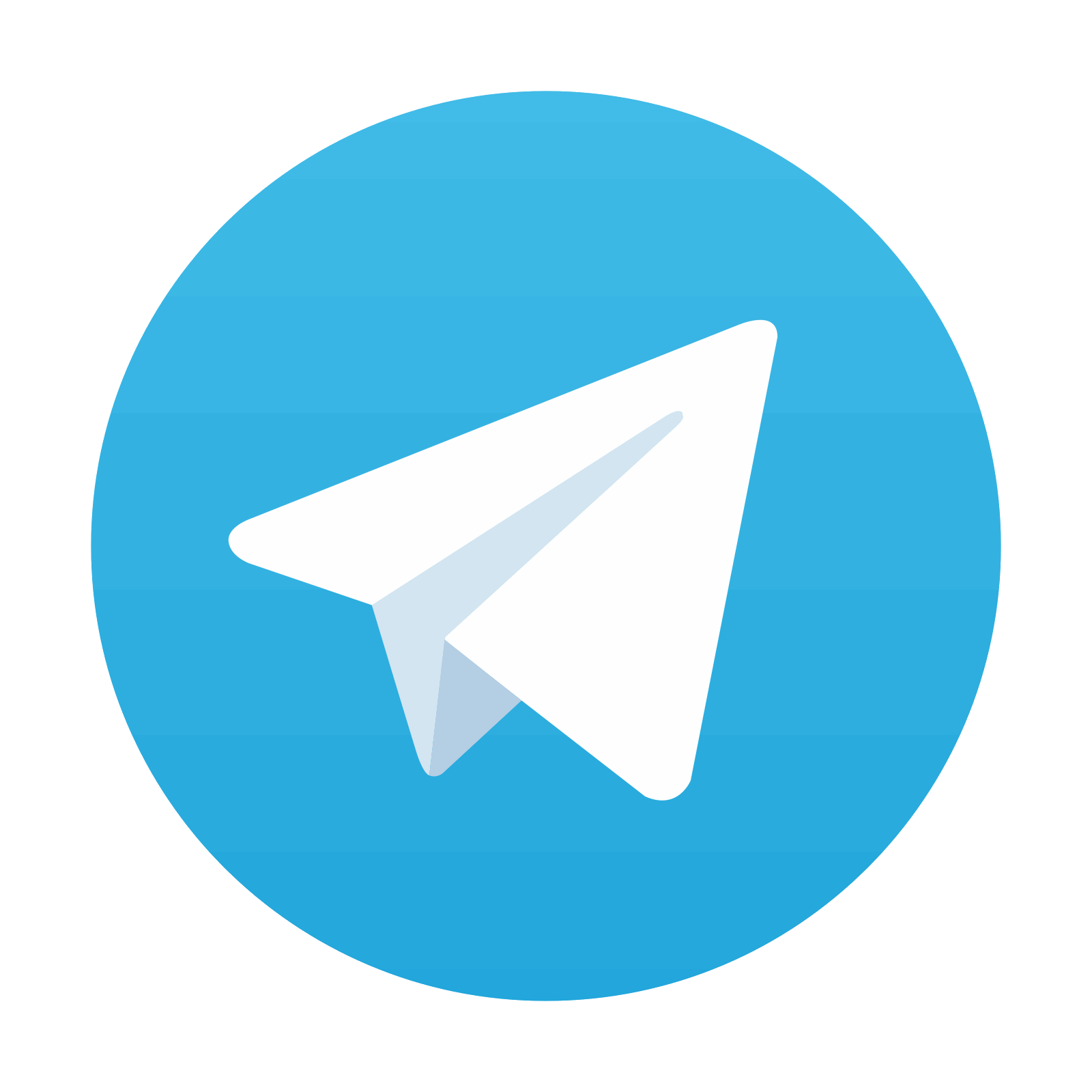
Stay updated, free articles. Join our Telegram channel

Full access? Get Clinical Tree
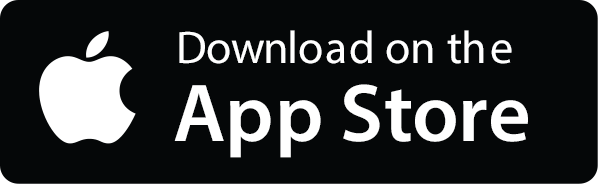
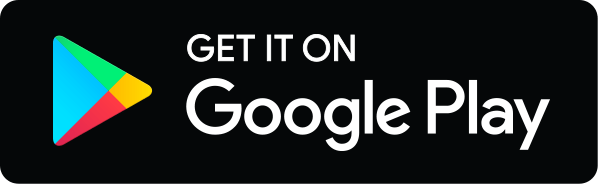