Class of modification
Modification to plasmid backbone
Resultant alterations
References
Increasing antigen expression
Gene promoter
Increased transcription
Intronic sequence
Increased transcription
Enhancer/transactivator
Increased transcription
Polyadenylation sequences
Increased transcription termination efficiency
Multiple stop codons
Increased transcription termination efficiency
[62]
Kozak sequence
Increased translation initiation
Codon optimization
Increased translation efficiency
Increasing antigen processing and presentation
Ubiquitination fusion
Increased degradation
PEST sequence
Increased ubiquitination and subsequent degradation
Amino-terminal residue
Increased degradation
Induced protein misfolding
Increased degradation
Signal sequence
Increased MHC class I presentation of minigenes
HSPs
Increased MHC I and II presentation, increased MHC expression
Target to endosome/lysosome
Increased MHC II processing and presentation
Intrinsic immunogenicity
CpG motifs and other DNA sensor motifs
Activation of innate immune responses
TH epitopes
Activation of CD4 + T cells, and subsequent production of immunostimulatory cytokines
Cytokines/chemokines
Enhanced T-cell activation
[147]
Co-stimulatory molecules
Enhance antigen-presentation capacity of APCs that take up plasmid
[147]
17.3 Engineering DNA Vaccines to Enhance Their Immune and Anti-tumor Efficacy
17.3.1 Increasing Antigen Expression
DNA vaccines are composed of a circular bacterial plasmid that normally contains a minimum of two components: the antigen expression unit (composed of promoter/enhancer sequences followed by the coding sequence for the targeted antigen) and the bacterial production unit (composed of bacterial sequences necessary for plasmid amplification and selection). The construction of bacterial plasmids with vaccine inserts is accomplished using recombinant DNA technology, where the cDNA of the tumor antigen is cloned into a bacterial DNA plasmid backbone under the expression of a eukaryotic promoter. Once constructed, the vaccine plasmid is transformed into bacteria, where bacterial growth produces multiple plasmid copies. The plasmid DNA is then purified from the bacteria by separating the circular plasmid from the much larger bacterial DNA and other bacterial impurities and then used for immunization studies. However, while this is the base construct for a DNA vaccine, several elements can be incorporated into this DNA backbone to enhance the expression of the encoded antigen when delivered to recipient APCs, and this increased expression can result in stronger antigen-specific immune responses.
17.3.1.1 Promoters and Transcriptional Enhancer Elements
While early DNA plasmids were designed to drive gene expression using strong promoters from oncogenic viruses such as SV40 or RSV, the vaccines that have been translated into human studies have predominantly utilized promoters from nononcogenic sources, such as the human cytomegalovirus immediate–early (CMV IE) promoter [92, 93]. Alternatively, groups have investigated the use of tissue-specific promoters to drive antigen expression in specific cell types, to both specifically target the cell of interest as well as avoiding potentially detrimental effects of antigen expression in irrelevant cell types. This technique has been used to specifically target myocytes or keratinocytes and could also be used to specifically drive antigen expression within dendritic cells, B cells, or macrophages, selectively targeting host APCs for expression of the antigen of interest [93, 94].
In connection with the promoter of interest, gene expression can be enhanced by including an intronic sequence. For example, when using the CMV IE promoter, the CMV intron A sequence is commonly incorporated into the plasmid backbone. Inclusion of introns has been shown to result in increased gene expression, which occurs due to increased gene transcription [95–97]. Similarly, the incorporation of various enhancer, transactivator, and leader sequences can further enhance gene transcription. One commonly used element is the human T-cell leukemia virus (HTLV) 5′ long terminal repeat (LTR), which can enhance transcription and the inclusion of which has been shown to enhance immune responses following DNA vaccination [62, 98]. Additionally, in studies evaluating an HIV DNA vaccine, the incorporation of an IgE leader sequence enhanced the immunogenicity of this vaccine [99, 100].
17.3.1.2 Transcription Termination Signals
While the use of strong promoters incorporated into DNA vaccines can help drive expression of the encoded antigen, the termination of gene transcription is also a process that needs to be enhanced to ensure that there is not a bottleneck that slows the overall gene expression process. Therefore, DNA vaccines commonly incorporate polyadenylation (poly-A) sequences downstream of the encoded antigen that can enhance the efficiency of transcription termination and export of mRNA from the nucleus. The selection of an appropriate poly-A signal is an important factor in the construction of an appropriate DNA vaccine backbone, as it can have a significant impact on the efficiency of the promoter in driving gene expression [101]. Commonly used poly-A sequences are from SV40, rabbit β-globulin, and especially bovine growth hormone, which has a synergistic effect on gene transcription when combined with the CMV promoter [62, 93, 101]. Another important method of ensuring efficient termination of transcription is including a second stop codon to the 3′ end of the gene of interest. This can prevent read through by RNA polymerase, which can potentially result in mRNA products that are the incorrect size and lead to RNA secondary structures which can decrease mRNA stability and impede efficient translation.
17.3.1.3 Kozak Sequence
In addition to adding DNA elements that can enhance the transcription of the encoded antigen, DNA vaccines can also be engineered to include factors that can drive translation of the gene transcript. One of these elements is a Kozak sequence, a small consensus sequence (GCCGCC(A/G)CC) located immediately upstream to the ATG start site that can serve as a eukaryotic ribosomal initiation sequence, slowing the rate of scanning by the ribosome and increasing the chance of recognition of the AUG start site [93, 102]. Interestingly, we have found that inclusion of this sequence can be required for efficient translation of a gene product encoded by a DNA vaccine [103 and Olson and McNeel, unpublished observations].
17.3.1.4 Codon Optimization
Another important factor that can affect the efficiency of protein translation is codon usage. All species have different ideal codon usage; therefore, optimizing the specific codons used can have a direct impact on how efficiently these codons are translated into protein. Doing so will optimize the transcribed product to the tRNAs that are most commonly available in the host organism, as well as avoiding secondary RNA structures that can have a detrimental impact on RNA stability. The impact of codon optimization has been shown in DNA vaccines for a variety of diseases, including studies using DNA vaccines encoding bacterial and parasite epitopes where the natural sequences were altered to encode optimized codons for expression in mammals, which led to enhanced expression and immune responses [93, 104, 105]. Codon optimization has also been shown to impact the immune and anti-tumor efficacy of DNA vaccines for cancer. In preclinical studies using a DNA vaccine targeting the oncogenic protein E7 from HPV16, a codon-optimized construct was able to induce protein expression several fold higher than the native codons, induced a stronger immune response, and protected immunize animals from tumor growth [106–108].
17.3.2 Increasing Antigen Processing and Presentation
After DNA vaccines are taken up by host APCs and the encoded gene is transcribed and translated, the next step in the antigen-presentation pathway is that the newly translated protein is degraded by the proteasome into small peptide fragments. These peptides are then shuttled to the endoplasmic reticulum (ER), where they bind to MHC class I molecules and are then sent to the surface of the cell and presented to circulating CD8+ T cells. Alternatively, for presentation on MHC class II molecules, an extracellular antigen (either the protein itself or a non-APC that has taken up the DNA plasmid and expressed the antigen) is taken up by professional APCs, and the antigen-containing endosome then degrades this antigen into short peptide fragments. Meanwhile, MHC class II molecules are synthesized in the ER and then secreted, where they will then fuse with antigen-containing endosomes. As a result, the antigen-derived peptides can bind to MHC class II and are then shuttled to the surface of the cell and presented to circulating CD4+ T cells. As this processing and presentation pathway plays a central role in the development of antigen-specific immunity, research has focused on engineering sequences into the plasmid DNA that can increase antigen degradation, processing, and presentation (reviewed in [109]).
17.3.2.1 Increasing Antigen Degradation
To enhance antigens being presented through the MHC class I pathway, a variety of approaches have been taken to increase the degradation of vaccine-encoded antigens by the proteasome. Some of this research has focused on increasing protein ubiquitination (ubiquitin being a post-translational modification that triggers transport to the proteasome) by directly fusing an ubiquitin moiety to the gene of interest, which can serve as a base for subsequent polyubiquitination (which is required for efficient proteasomal degradation [110]). This has been shown to enhance the proteasomal degradation of antigens encoded by DNA vaccines, as well as inducing stronger antigen-specific immune responses, in several disease models including cancer [111–115].
In addition to directly fusing ubiquitin moieties to the encoded antigen, increased protein degradation can be induced by incorporating specific sequences that target proteins for proteasomal degradation, such as a PEST sequence. A PEST sequence is a short region of amino acids rich in proline (P), glutamic acid (E), serine (S), and threonine (T) and are associated with decreased protein half-life [116]. This has been translated to studies using DNA vaccines in several disease models, including HIV and cancer [117, 118]. In a study evaluating immune responses to the tumor-associated antigen E7 from HPV16, it was found that a PEST sequence associated with the gene of interest was required for maximal immune and anti-tumor efficacy [117]. In addition to PEST sequences, increased protein degradation can be induced simply by altering the amino acid that is encoded at the amino terminus of the protein. This so-called N-end rule has shown that the amino acid that is exposed at the amino terminus following the removal of the initiating methionine can have a direct impact on the half-life of the protein [119]. This has been incorporated into DNA vaccines, where proteins whose amino termini are engineered to have shorter half-lives have been shown to induce stronger antigen-specific immune responses [120, 121].
In addition to these specific mechanisms to increase proteasomal degradation of encoded antigens, other characteristics of the antigen of interest can be engineered to enhanced protein degradation. For example, misfolded proteins are rapidly ubiquitinated and degraded by the proteasome. This can be utilized in DNA vaccines by incorporating protein sequences that trigger protein misfolding, which has been shown to increase the generation of MHC class I-restricted peptides [122]. Alternatively, misfolding can be induced by altering the sequence of the encoded antigen (for example, putting the carboxy terminal domain at the 5′ end of the coding sequence). We have observed increased proteasomal degradation in our own studies evaluating a DNA vaccine targeting a domain of the androgen receptor, which is quickly degraded via the proteasome [103 and Olson and McNeel, unpublished observations].
17.3.2.2 Increasing Antigen Presentation
While generating increased frequencies of MHC-restricted peptides can result in enhanced immune responses, it is necessary that these peptides are appropriately presented on MHC class I molecules on the surface of cells. This presentation process can be enhanced in a number of ways. One technique that is used to enhance the presentation of DNA vaccines encoding minigenes (specific T-cell epitopes, rather than the full-length protein) is to incorporate a signal sequence proximal to the 5′ end of the coding sequence. This triggers the gene product to enter the secretory pathway, first being transported to the ER, where it can subsequently bind to MHC class I and be presented on the surface of cells [123]. This has been shown to enhance antigen-specific T-cell responses and can increase the anti-tumor efficacy of vaccines [124–126].
In addition to directly routing peptides to the ER, another method that has been shown to increase antigen presentation is incorporating heat shock proteins (HSPs) into antigen-specific vaccines. These HSPs induce antigen-specific immune responses using a variety of mechanisms: HSP-derived peptides have been shown to be immunologically recognized, activating innate immunity by acting as a danger signal, increasing MHC class I expression, and triggering expression of immunostimulatory cytokines [127–130]. In addition, HSPs have been suggested to directly increase the presentation of antigen-derived peptides by facilitating their transport to the ER or to other APCs, and DNA vaccines fusing antigen to HSPs have been shown to increase antigen presentation and induce enhanced immune responses [131–134].
17.3.2.3 Increasing MHC Class II Antigen Processing and Presentation
While engineering DNA vaccines to enhance the degradation and presentation of intracellular antigens is useful when one is trying to elicit a CD8+ T-cell response against an intracellular antigen, sometimes it is desirable to focus on enhancing MHC class II-restricted immune responses. Some of the previously described methods, in particular fusion constructs encoding both the antigen of interest and HSPs, as well as the incorporation of signal sequences upstream of full-length antigens, can be used to enhance cross-presentation of antigens encoded by DNA vaccines. However, there are specific modifications that can be engineered into DNA vaccines that can enhance antigen presentation on MHC class II molecules. One such modification is to direct the antigen of interest to be trafficked to the endosomal/lysosomal pathway. This is commonly achieved by fusing the gene of interest to the cytoplasmic domain of lysosome-associated membrane protein 1 (LAMP-1), which contains an amino acid sequence that targets antigens to the endosomal/lysosomal pathway. This has been used in the context of DNA vaccines, where a vaccine targeting HPV16 E7 fused to this region of LAMP-1 enhanced the immune and anti-tumor efficacy of this vaccine [108, 135].
17.3.3 Intrinsic Immunogenicity of DNA Vaccines
While the primary goal of DNA vaccines for the treatment of cancer is to elicit and/or augment an antigen-specific immune response to a particular tumor-associated antigen, enhancing this antigen-specific response does not solely focus on making changes to the antigen of interest. Other antigen-nonspecific elements can be engineered into DNA vaccines that can activate innate and adaptive immunity, which can subsequently enhance the immune response directed against the antigen of interest.
17.3.3.1 CpG Motifs
While DNA vaccines are designed to elicit immune responses to the encoded antigen, plasmid DNA itself has been shown to be immunogenic. This is largely through the incorporation of Immunostimulatory sequences (ISS), which contain cytosine-guanine (CpG) nucleotide repeats. These repeats are unmethylated when the DNA plasmid is produced in bacteria, and this unmethylated DNA is then recognized as foreign when injected into mammals. As a result, these CpG repeats have been shown to activate innate immune responses (in part through signaling via TLR9) and can enhance the immune efficacy of DNA vaccines through the recruitment of immune populations and increased expression of immunostimulatory cytokines [136–139]. Additionally, other DNA sensors can recognize plasmid DNA and can lead to the activation of innate immune responses [140–143].
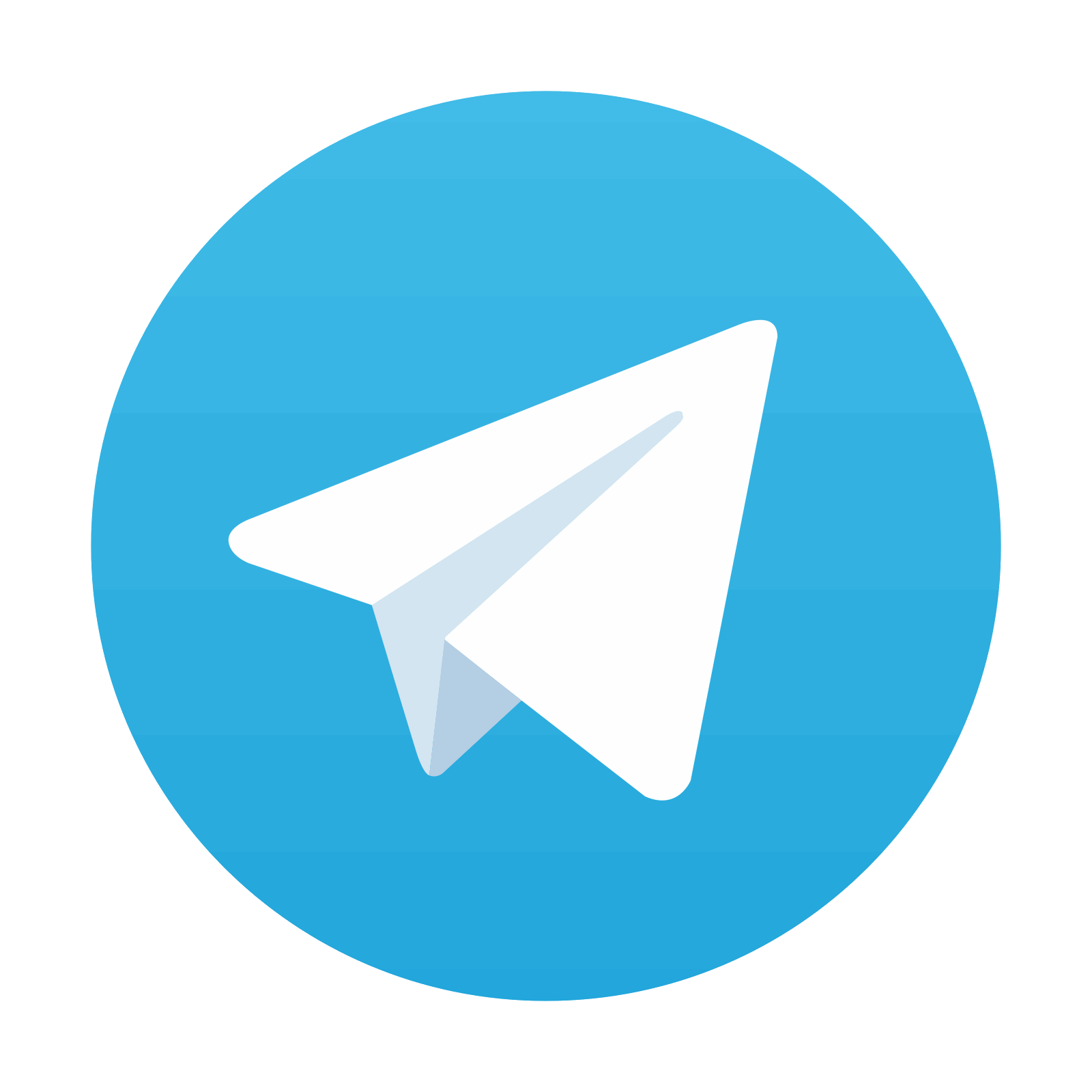
Stay updated, free articles. Join our Telegram channel

Full access? Get Clinical Tree
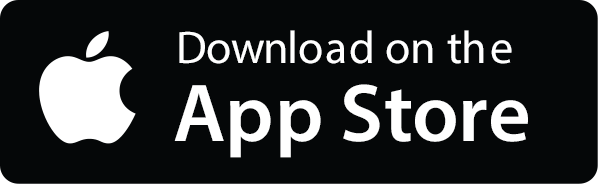
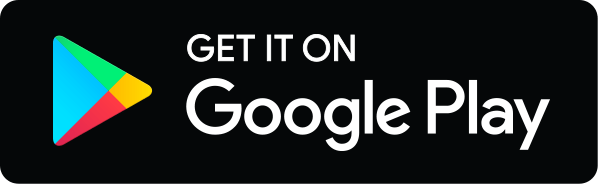