Fig. 16.1
Aptamers against cell surface proteins. Aptamers can act both as direct antagonist of the transmembrane receptor targets a and as delivery agents (b–g). b Aptamer–streptavidin–siRNA conjugates; c aptamer–siRNA chimeras; d aptamer–radionuclide/fluorescent agent conjugates; e aptamer–doxorubicin; f aptamer–protein conjugates; g aptamer–nanoparticles–drug conjugates
16.3 The Conjugation of Aptamers
Biomolecules can be conjugated with other biomolecules, forming bioconjugates, and with chemical entities of a different nature, giving rise to assemblies endowed with new properties. In the field of medicinal chemistry, the goal of conjugation is, for example, the enhancement of drug potency, a more precise and effective targeting, an improvement in absorption, distribution, metabolism, elimination, and toxicity, the development of new therapeutic systems, the development of novel diagnostics and biosensors. Common components of conjugates are small molecules, such as drugs, biotin, fluorescent dyes, and larger molecules, such as proteins, oligosaccharides, nucleic acids, synthetic polymers, and also nanomaterials.
Aptamers are commonly synthesized by solid-phase chemical synthesis; therefore, chemical conjugation is possible at any position in the molecule, differently from proteins and peptides that can accept conjugation only on specific residues.
Common strategies for the post-synthesis, covalent bioconjugation of aptamers exploit the coupling of a terminal group like an amino group with a reactive succinimidyl ester or of a sulfhydryl group with a reactive maleimide.
Common strategies for the post-synthesis, non-covalent bioconjugation of aptamers are mainly based on interactions regulating nucleic acids and proteins structural assembly and association, involving the use of post-synthetic linkers, of sticky ends, or similar ways.
16.3.1 Conjugation of Aptamers with siRNA/miRNA
Because of their nucleic acid nature, the rational design of structured conjugates of aptamers with siRNA, with miRNAs, or even with larger therapeutic RNA-based molecules is greatly simplified. Recently, different groups have constructed distinct aptamer–siRNA conjugates for successful delivery of siRNA into target cells by employing diverse strategies to realize the chimeric molecules. Since aptamers are commonly prepared by automated synthesis, they can be directly fused with their partner component in the chimeric molecule by the design and synthesis of single longer molecules. Additionally, particular labels, like many dyes or biotin, can be directly added as labeled phosphoramidite within the same process. In addition, aptamers and siRNA molecules can be assembled via a protein connector, or alternatively, completely RNA-based chimeric molecules can be generated by means of several approaches (see below).
16.3.1.1 Streptavidin–Aptamer–siRNA Conjugates
One of the first studies on the development of aptamer–siRNA conjugates involved the non-covalent conjugation of a 27-mer siRNA-targeting laminin A/C and GAPDH genes with the antiprostate-specific membrane antigen (PSMA) A9 RNA aptamer via a streptavidin bridge [32]. The siRNA aptamer and the RNA aptamer were chemically conjugated with biotin. Further, to enhance siRNA release in the cytoplasm, a reducible disulfide linker was designed between the sense strand of siRNA and the biotin group. In cell-based assays, the resulting conjugates were efficacious in silencing target genes at levels comparable with what observed with conventional lipid-based reagents.
16.3.1.2 Aptamer–siRNA/MiRNA Conjugates Consisting Only of RNA Components
Research has gone beyond with the aim to develop completely RNA-based delivery methods, thus reducing the various side effects associated with reagents such as proteins. The first report of a delivery system consisting only of RNA components was by McNamara et al. [33]. They described an approach in which the anti-PSMA A10 aptamer was covalently conjugated at the 3′ end to the 21-mer sequence complementary to the antisense strand of the siRNA and the chimeras were formed by annealing to the siRNA antisense strand. The resulting aptamer–siRNA molecules were shown to be selectively internalized into PSMA-positive cells and to effectively target the tumor survival genes polo-like kinase 1 and BCL-2. Further developments, including truncation of the aptamer portion, swapping of the sense and antisense strands of the siRNA portion, and addition to the chimera of a two-nucleotide 3′-overhang and of a PEG tail, produced analogous chimeric RNAs displaying enhanced silencing activity and specificity, and optimized in vivo kinetics [34]. By applying the same approach, PSMA aptamer–siRNA fusions were generated to target two key components of the nonsense-mediated mRNA decay (NMD) [35]. Tumor-targeted NMD inhibition forms the basis of a clinically feasible approach to enhance the antigenicity of disseminated tumors leading to their immune recognition and rejection.
Again by using the anti-PSMA A10 aptamer as a vector, Wullner et al. succeeded in generating bivalent aptamer–siRNA constructs in which the siRNA against the eukaryotic elongation factor was used as a linker to join the two aptamers, or alternatively, the siRNAs were appended onto the 3′ ends of each aptamer [36].
Most work in the generation of aptamer–siRNA chimeric molecules has been performed by the group of Rossi JJ by using the 2′-F-modified RNA aptamer targeting the HIV-1 envelope glycoprotein gp120 as a vector. The gp-120 aptamer was covalently linked to siRNAs that target the HIV-1 tat/rev common exon, thus generating a novel anti-gp120 aptamer–siRNA chimera in which both components function as anti-HIV agents, thus showing a dual inhibitory function [37]. Further, a highly versatile approach was developed by the same group by using a G-C-rich dsRNA of 16 nucleotides “stick” as the scaffold to link the aptamer and the siRNAs. The two complementary sticky sequences were chemically conjugated at the 3′ end of the aptamer and of one of the two siRNA strands, thereby allowing the aptamer and siRNA portions to be non-covalently conjugated via Watson–Crick base-pairing by simple mixing. Both aptamer and siRNA were separated from each sticky strand by a flexible seven-unit three-carbon linker. The assembly proved efficacious both in vitro and in vivo [38, 39]. The functional assembly “aptamer–stick–siRNA” displayed a great versatility offering the possibility to easily combine different siRNAs to the vector aptamer, thus envisaging the possibility of using these chimeras for an antiretroviral siRNA combinatorial therapy [26, 40].
Recently, by using the delivery stick approach, we have generated completely RNA-based chimeric molecules containing internalizing aptamers coupled to therapeutic miRNAs that are down-regulated in human tumors and whose expression results in selective tumor growth inhibition. We have shown that when applied to cells expressing the specific aptamer target, the chimeric molecules are internalized and processed by Dicer, thus increasing miRNA cellular level and inhibiting miRNA target protein (our personal communication).
Two approaches have been reported to generate RNA-based chimeric molecules by directly fusing the aptamer with its partner component in a single longer molecule. In the first construct, Ni et al. [41] linked a short hairpin RNA against the DNA-activated protein kinase to a truncated A10 aptamer generating a single intact nuclease-stabilized molecule. The 3′-terminus of the A10 aptamer was conjugated to the passenger (sense) strand, followed by a 10-mer loop sequence and then by the guide or silencing (antisense) strand of the siRNA. In the second construct, chimeric molecules containing internalizing antimucin 1 (MUC1) aptamer fused to therapeutic miR-29b palindromic sequence were generated by direct synthesis. Applied to cells expressing the specific aptamer target, the chimeric molecules proved to be internalized and to increase miRNA cellular level [42].
With the increasing development of the conjugation strategies, the list of aptamers against surface epitopes that are being used as delivery agents for siRNA/miRNA is growing rapidly and now includes, in addition to PSMA and gp120 aptamers, those against CD4 [43], epidermal growth factor receptor 2 (HER2) [22], MUC 1 [30, 42], B cell-activating factor (BAFF) receptor (BAFF-R) [44], transferrin receptor [45], (see Table 16.1).
Table 16.1
Aptamers as delivery agents for siRNA/miRNA
Aptamer composition | Target | siRNA/miRNA delivery | References |
---|---|---|---|
RNA, 2′-F-Py | PSMA | siRNA | |
RNA, 2′-F-Py | PSMA | miRNA | [51] |
RNA, 2′-F-Py | gp120 | siRNA | |
RNA, 2′-F-Py | CD4 | siRNA | [43] |
RNA, 2′-F-Py | HER2 | siRNA | [22] |
DNA | Mucin 1 | miRNA | |
RNA, 2′-F-Py | TfR | siRNA | [45] |
RNA, 2′-F-Py | BAFF-R | siRNA | [44] |
16.3.2 Covalent Conjugation of Aptamers with Drugs
Conjugation of aptamers with drugs can be used as a tool favoring specific cell targeting and internalization, in particular for drugs like antitumor drugs, which often lack cell specificity and produce life-threatening toxic side effects in patients.
Doxorubicin is the most utilized anticancer drug against neoplasms including acute lymphoblastic and myeloblastic leukemias, and malignant lymphomas, although producing heavy side effects among which cumulative cardiac damage. In order to improve its therapeutic potential, doxorubicin was covalently conjugated to DNA aptamer Sgc8c [46], selected for human T cell ALL CCRF-CEM cell lines, that can act as a drug carrier, targeting protein tyrosine kinase 7 (PTK7), a transmembrane receptor highly expressed in CCRF-CEM cells. To this aim, doxorubicin was reacted with N-(ε-maleimidocaproic acid) hydrazide to yield the C-13 (6-maleimidocaproyl) hydrazone derivative and then combined with the 5′-thiol-modified sgc8c DNA, prepared by automated synthesis. The linked sgc8c aptamer prevents the non-specific uptake of doxorubicin and decreases cellular toxicity to non-target cells.
However, despite the fact that anti-CEM/PTK7 aptamer has been reported to bind specifically to leukemia cell lines thus indicating PTK7 as a new biomarker specific for leukemia cells, Li et al. by examining how the aptamer performed with additional cell lines, which were not of hematopoietic origin, showed that it is possible that the aptamer instead of a specific binding to PTK7 may identify a propensity for adherence, thus needing further investigation [47].
16.3.3 Conjugation of Aptamers with Synthetic Polymers
Conjugation of biomolecules to synthetic polymers, such as polyethylene glycol (PEG), is used to increase the hydrodynamic volume to molecular weight ratio and thus improve their performance as therapeutics influencing their permanence in the body. Aptamers are relatively small, and charged molecules subject to rapid renal elimination and PEGylation of aptamers can overcome this potential limitation. PEG shows low toxicity, non-immunogenicity, and high solubility in water and is commercially available in various configurations (linear, branched, or comb-shaped) and molecular weights and with a large variety of terminal functional groups suitable for conjugation.
PEGylated aptamers have been prepared by coupling the activated polymer to amino-modified aptamers [48]. In addition, comb polymers of PEG acrylate containing a pyridyl disulfide terminus have been reversibly conjugated to siRNAs [49].
As an example of more recent PEGylation chemistry, a 3′-thiol-modified (disulfide protected) 25-nucleotide DNA aptamer, selected against the protein core of MUC1 glycoprotein, was successfully conjugated via a maleimide–thiol reaction to a range of maleimide-activated PEGs. The affinity of the PEG–aptamer conjugate for the target resulted to vary according to the structure and conformation of the synthetic polymer [50].
PEG was also used as a spacer and linker for a second polymer, polyamidoamine (PAMAM), to construct a multicomponent, multifunction aptamer conjugate. PAMAM, due to the positively charged amino groups present on its surface, can bind, transport, and deliver therapeutic nucleic acids like siRNA and miRNA. The multicomponent conjugate was accomplished by combining first PEG with PAMAM and then adding aptamer A10-3.2, a shortened version (39 nucleotides) of PSMA-specific aptamer A10, more easily synthesized, more stable, and more efficient than A10 itself. In details, PAMAM dendrimer was reacted with α-maleimidyl-ω-N-hydroxysuccinimidyl polyethylene glycol to give PAMAM/PEG conjugate further reacted with sulfhydryl A10-3.2. The conjugate PAMAM/PEG/aptamer was used as a vehicle for the safe and effective target delivery of miR-15a and miR-16-1, identified as tumor suppressor genes in prostate cancer. These miRNA/PAMAM/PEG/aptamer conjugates proved ca. fivefold more active than miRNA/PAMAM/PEG conjugates, lacking the aptamer, in viability assays on PSMA-positive human prostate adenocarcinoma (LNCaP) cells [51].
16.3.4 Conjugation of Aptamers with Nanoparticles
The integration of cell-type-specific aptamers with nanocarriers like liposomes, micelles, synthetic polymer nanoparticles, carbon nanotubes, quantum dots (QDs), and other nanoparticles can produce new, versatile, and multifunctional specific delivery vehicles. Aptamer-functionalized nanoparticles have a size in the mid-nanometer range, allowing preferential accumulation in target tissues and organs through an enhanced permeability and retention effect, facilitating cellular entry by endocytosis. Conjugation with nanocarriers can also reduce renal clearance and improve circulation half-life and biodistribution in vivo [26].
16.3.4.1 Conjugation of Aptamers with Synthetic Polymer Nanoparticles
Aptamer–nanoparticles were prepared by first synthesizing a poly(lactic acid) (PLA)-block-PEG copolymer, with a terminal carboxylic acid functional group (PLAPEG- COOH), by ring-opening polymerization of the D,L-lactic acid dimeric lactide and OH-PEG3400-COOH. Nanoparticles were then prepared using the water-in-oil-in-water solvent evaporation procedure (double-emulsion method). These nanoparticles (Mn = 10,500) carry carboxylic acid groups available for covalent conjugation to amino-modified aptamers, forming a negative charge surface, which may minimize non-specific interactions with the negatively charged aptamers. The presence of PEG on particle surface enhances circulating half-life. The nanoparticles were conjugated with 3′-NH2-modified A10 RNA aptamer-targeting PSMA and the resulting bioconjugates proved to efficiently target and take up by PSMA-expressing prostate LNCaP cells [52].
16.3.4.2 Conjugation of Aptamers to Drug Carrier Synthetic Polymer Nanoparticles
An aptamer–nanoparticles-based delivery system, targeting the transmembrane MUC1 protein, overexpressed in most malignant adenocarcinomas, has been realized for delivering the anticancer drug paclitaxel to MUC1-positive tumor cells. To this aim, the S2.2, 25-nucleotide MUC1 aptamer was synthesized as chimeric ON with a 3′-NH2-modified 73-nucleotide spacer and covalently conjugated to a nanoparticle made of poly(lactic-co-glycolic-acid) (PLGA, 50:50, MW = 16,000). The paclitaxel–Apt–NP assembly was realized using the emulsion/evaporation method. The MUC1 Apt–NP system proved to enhance the delivery of paclitaxel to MUC1-positive MCF-7 cells in vitro [53].
16.3.4.3 Conjugation of Aptamers to Self-assembling Nanoparticles
Self-assembling nanoparticles, able to deliver siRNA and other therapeutics to targeted cells, can be obtained by fusion with the packaging phi29 motor RNA (pRNA). For example, a CD4 aptamer and a siRNA against survivine were covalently fused with pRNA and assembled into dimers that proved to specifically bind to CD4-expressing cells and to be internalized, knocking down the target transcripts [54].
16.3.4.4 Conjugation of Aptamers to Form Micelles
An amphiphilic block copolymer made of a hydrophilic oligonucleotide and a hydrophobic polymer, in aqueous solution, can self-assemble into a spherical or a nanorod-like micelle. An aptamer with a hydrophobic polymeric tail attached to its end can form a highly ordered micelle-like structure, in which the aptamer not only acts as the building block for the nanostructure, but also performs the recognition of its specific target. Furthermore, the dense packing of the aptamer in the micelle can greatly improve binding affinity to its specific targets. Micelles are also considered to be dynamic and soft materials, which may interact with the dynamic lipid bilayer of the cell membrane favoring drug delivery.
Aptamer TDO5, specific to Ramos cells (a B-cell lymphoma cell line), generated by automated synthesis, has been directly fused to a PEG linker carrying a diacyl lipid tail. This TDO5 amphiphilic conjugate self-assembled into a spherical micelle structure, as demonstrated by transmission electron microscopy (TEM). The average diameter of TDO5–micelles, estimated to contain 1,000 copies of conjugate unit, resulted 68 ± 13 nm, consistent with the hydrodynamic diameter of 67.22 nm measured by dynamic light scattering. The TDO5–micelles demonstrated extremely rapid recognition of the target cells, were found to enhance the binding capability of otherwise low-affinity TDO5 aptamer at physiological temperature, and helped cell internalization. Additionally, the aptamer–micelles displayed low k off once on the cell membrane, high sensitivity, low critical micelle concentration values, great dynamic specificity in flow channel systems that mimic drug delivery in a flowing system, thereby appearing to function as an efficient detection/delivery vehicle in the biological living system [55].
16.3.4.5 Conjugation of Aptamers with Liposomes
Liposomes can be loaded with pharmaceuticals, in particular highly toxic or poorly soluble chemotherapeutics. A therapeutic aptamer–liposome (100 nm diameter) drug delivery system was molecularly engineered by assembling hydrogenated soy phosphatidylcholine, cholesterol, methoxy poly-(ethylene glycol)-distearoyl-phosphatidyl-ethanolamine and maleimide-terminated poly-(ethylene glycol)-distearoyl-phosphatidyl-ethanolamine, in a second step covalently linked to 3′-thiol-modified sgc8 aptamer, which has high binding affinity toward leukemia CEM-CCRF cells. The liposome system proved stabilized by PEG coating and able to bind target cells and deliver a model drug [56].
16.3.4.6 Conjugation of Aptamers with Gold Nanoparticles
Gold nanoparticles (AuNPs) hold great promise for biological and medicinal applications. AuNPs can be synthesized by reducing tetrachloroauric acid with trisodium citrate. AuNPs can be characterized by UV–vis spectroscopy and TEM. The concentration of the AuNPs can be calculated according to Beer’s law using an extinction coefficient of 2.4 × 108 M−1 cm− 1 at 520 nm for the 12 nm AuNPs [57].
They have unique colorimetric, conductivity, and nonlinear optical properties, easy surface conjugation with biological entities, high stability, biological compatibility, controllable morphology, and size dispersion. On the basis of their unique surface plasmon resonance (SPR) property, dispersed AuNPs appear red, whereas their aggregates appear purple allowing target-induced colorimetric assays generally based on their assembly or disassembly associated with target recognition [58].
Detection methods relying on the conjugation of ONs with AuNPs show more sensitivity than conventional assays based on ONs probes, so that AuNPs functionalized in particular with an aptamer (Apt) provide a powerful platform for targeted delivery, detection, and therapy. Some tests based on Apt-AuNPs have been commercialized [59].
AuNPs can be directly functionalized with thiolated aptamers and other thiolated ONs by chemisorption. With the introduction of the Apt-AuNPs platform, many kinds of analytes have been monitored on the basis of different mechanisms.
According to a bridging mechanism, AuNPs modified with aptamers for platelet-derived growth factors (PDGFs) produced a highly specific sensing system with detection limits of 3.2 nM [60]. Aggregation and redispersion of AuNPs connected to specific color changes can be achieved by inducing loss or screening of surface charges [61]. Assays based on the process of AuNPs disassembly can be performed with ODNs-AuNPs first cross-linked by an aptamer sequence to form aggregates that are forced to dissociate upon binding of a specific target [62].
Aptamers linked to the surface of solid AuNPs can result hindered in assuming the functional conformation with respect to free solution, and their target recognition ability can result impaired. To overcome this problem, AuNPs have been assembled with ONs complementary (cON) to a non-functional part of the aptamer sequence, allowing the subsequent hybridization of the aptamer, in this way leaving the functional part more exposed to solution and free to assume the appropriate conformation [63]. Taking advantage of the highly efficient fluorescence quenching properties of AuNPs for proximately fluorescent dyes through energy transfer processes (FRET) [64], this method of bioconjugation was applied to perform the multiplex detection of small molecules (adenosine, potassium, and cocaine) through the utilization of aptamer-based multicolor fluorescent AuNPs probes.
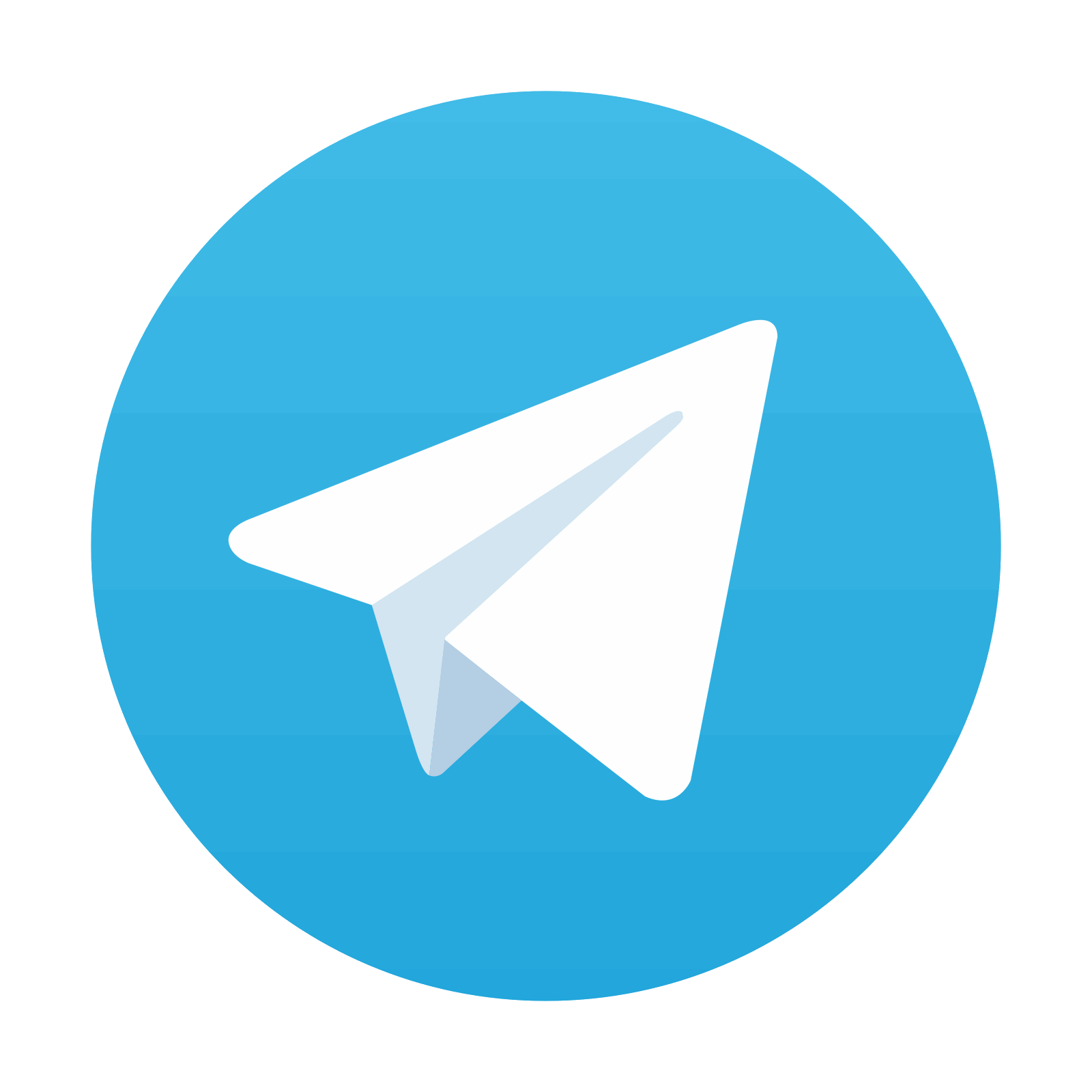
Stay updated, free articles. Join our Telegram channel

Full access? Get Clinical Tree
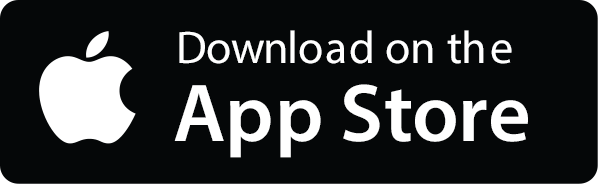
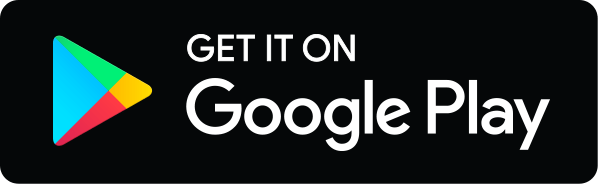