Endocrine Physiology and Mechanisms of Hypothalamic-Pituitary Regulation
Jacquelyn L. Banasik
Key Questions
• How does the lipid or water solubility of hormones affect their transport in the circulation?
• How do target cells regulate their responsiveness to endocrine hormones?
• How do feedback mechanisms control the secretion of hormones?
• How are thyroid and steroid hormones synthesized?
http://evolve.elsevier.com/Copstead/
The endocrine system is composed of cells and organs that are specialized to synthesize and secrete hormones into the bloodstream to act at distant target cells. The nervous and endocrine systems are closely integrated, and many of their actions are coordinated at the level of the hypothalamus. Endocrine systems are particularly suited for regulating complex functions that involve numerous tissues and organs such as growth, metabolism, fluid balance, responses to stress, and reproduction.1 It is now appreciated that all cells in an organism are involved in cell-to-cell communication, not just the specialized endocrine cells; therefore the term classical endocrine systems is sometimes used to designate specialized endocrine glands. This chapter describes general principles of endocrine communication systems and explores the details of hypothalamic-pituitary regulation of antidiuretic hormone, oxytocin, growth hormone, prolactin, gonadotropins, thyroid hormone, and corticosteroid hormones. Disorders of these systems are discussed in Chapters 40 and 41. Reproductive function and disorders can be found in Unit IX.
Hormone Structure and Action
An endocrine hormone may be defined as a blood-borne chemical messenger that has an effect on target cells anatomically distant from the secreting cell (Figure 39-1). Chemical messengers may also act in a paracrine or autocrine fashion; the hormone molecule is secreted by one cell and affects adjacent cells, or the original secreting cell. Paracrine and autocrine actions of hormones are not traditionally included in endocrine system physiology; however, the same signaling molecules can have autocrine, paracrine, and distant actions. As an example, estrogen acts locally within the ovary potentiating maturation of ova but is required systemically for outward female sexual differentiation.2 Secretion of hormones into the bloodstream by neurons may be termed neurocrine signaling, a process common to the hypothalamic-pituitary communication system.
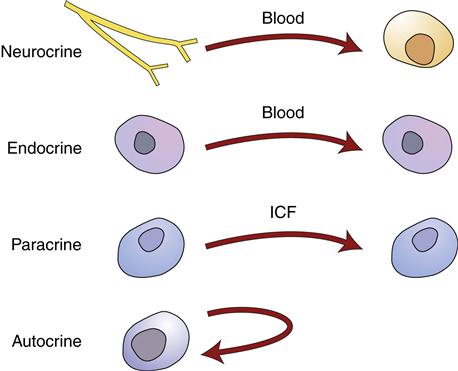
Chemical Structure of Hormone Classes
Hormones may be classified according to chemical structure as peptides, tyrosine-derived catecholamines and thyroid hormones, and steroids (Box 39-1). The great majority of endocrine hormones are peptides (small proteins) and are water-soluble and easily transported through the circulation to target cells.3 The catecholamines (dopamine, epinephrine, and norepinephrine) are also water-soluble. Water-soluble hormones usually travel free in solution in the plasma, although some are partially protein-bound. Water-soluble hormones are unable to cross the plasma membrane to enter cells and therefore must exert their actions by binding to receptors located on the surface of target cell membranes.1
Thyroid hormones (T3 and T4) are derived from tyrosine amino acids to which iodine has been attached. The steroid hormones are derived from cholesterol. Thyroid and steroid hormones require transport proteins (globulin) to convey them through the circulation because they are poorly soluble in the blood. In some cases these transport proteins are specialized to carry a certain hormone (thyroxine-binding globulin, cortisol-binding globulin), but hormones can also be carried by nonspecific proteins, such as albumin.1 Transport proteins are manufactured by the liver. At the target cell, the hormone detaches from the transport protein and moves through the cell membrane to activate intracellular receptors in the cytoplasm or nucleus.1
Mechanisms of Hormone Action
Hormones with Cell Membrane Receptors
Hormones exert their actions by binding to target cell receptor proteins. The target cell receptors for water-soluble hormones have a hormone-binding site located on the external portion of a specific cell-surface receptor. Once hormone-receptor binding takes place, a change in the conformation of the receptor protein conveys a signal to the interior of the cell. Some receptors generate second messengers, such as cyclic adenosine monophosphate (cAMP), within the cell whereas others become activated enzymes. The intracellular activities occurring after hormone-receptor binding may include changes in cell membrane permeability; activation or inactivation of enzymes; cellular maintenance, growth, and differentiation; protein synthesis; and genetic expression via RNA and DNA synthesis in the cell nucleus.4 A more detailed explanation of receptor mechanisms can be found in Chapter 3.
G-protein–linked receptors
Hormones can be described as “first messengers”; the hormone carries a message from the secreting cell to the target cell. A second messenger is generated within the cell in response to the first message. The term second messenger is usually applied to the products generated by a class of receptors called G-protein–linked (or G-protein–coupled) receptors. These receptors undergo a conformational change upon binding to a hormone that allows them to interact with a class of proteins, called G-proteins, located on the inner side of the cell membrane (Figure 39-2). G-Proteins have three subunits and when activated by the receptor, one of these subunits (α) binds to a GTP molecule and then dissociates from the other subunits (β, γ).3 The activated α subunit is able to move along the inner membrane and interact with target enzymes, inducing them to produce the second messenger. There are several different forms of G-proteins that have different target enzymes and produce different sets of second messengers (see Chapter 3). The most important of these for endocrine systems are the Gs and the Gq subtypes. Hormones that bind to receptors that are linked to Gs increase the production of cAMP within the cell. The usual downstream target of cAMP is an enzyme called protein kinase A. Kinases are enzymes that attach phosphates (phosphorylation) to target proteins to change their activity. Enzymes within the cell called phosphorylases work to remove the attached phosphates. Phosphorylation-dephosphorylation is a common strategy for controlling enzyme activity in cells. Activation of protein kinase A causes varied cellular effects depending on the cell type. Important examples of hormones that are linked to increased cAMP production through activation of the Gs pathway include adrenocorticotropic hormone (ACTH), thyroid-stimulating hormone (TSH), follicle-stimulating hormone (FSH), luteinizing hormone (LH), epinephrine (β receptors), parathyroid hormone, antidiuretic hormone (ADH), and glucagon.1
The Gq pathway is linked to production of two second messengers, diacylglycerol (DAG) and inositol trisphosphate (IP3). Norepinephrine and epinephrine can activate the Gq pathway by binding to α1 receptors on target cells. DAG and IP3 work together to increase the activity of protein kinase C, which then phosphorylates downstream target proteins to change cell behavior.
Protein kinase receptors
A second class of surface receptors important in endocrine signaling is the protein kinase receptor family. These receptors either become activated kinases themselves or are associated with cytoplasmic kinases on their inner domain (Figure 39-3). Both types of receptors phosphorylate their target proteins upon binding of the hormone to an external binding site. An important example of a receptor kinase is the insulin receptor that phosphorylates tyrosine amino acids in its targets and activates several different intracellular signaling cascades (Chapter 41). Growth hormone and prolactin receptors are associated with Janus kinase (JAK) on the cytoplasmic side and become activated when the hormone binds to the outside of the receptor, causing a conformational change that pulls two JAK enzymes closer together.1 The JAK enzymes become activated and phosphorylate their target proteins, initiating a signaling cascade.
Amplification of Hormone Activity
The process of intracellular activation by secondary signals occurs via a cascade effect. Progressively larger numbers of chemical reactions occur at each step, so that activation of one receptor at the cell surface can activate numerous G-proteins.3 Each activated G-protein may interact with 100 or more target enzyme molecules, such as adenylyl cyclase. Each activated enzyme produces many molecules of a second messenger (cAMP), and each second messenger molecule activates many molecules of protein kinase, and so forth. This mechanism of signal amplification explains why minute amounts of circulating hormone cause significant and rapid cellular and systemic effects.
Hormones with Intracellular Receptors
Receptors for thyroid and steroid hormones are located in the cytoplasm or the nucleus of the target cell (Figure 39-4). For decades the transport of thyroid hormone across the cell membrane was thought to be a passive process of diffusion through the bilayer; however, recent discoveries indicate that special protein carriers in the membrane are necessary to transport thyroid hormone into the cell.5 Steroid hormones are still believed to be able to pass directly across the plasma membrane to interact with intracellular receptors.6 However, they may also bind cell-surface receptors as evidenced by the discovery that estrogen can bind to a G-protein–linked receptor on some cells. These G-protein receptors achieve a more rapid change in cell behavior than those associated with intracellular receptors. Binding of the hormones to intracellular receptors causes a change in affinity of the receptor for binding sites on DNA in the cell nucleus.4 Gene expression is changed by binding of the hormone-receptor complex to specific DNA-binding sites. The events that result from the interaction between nuclear DNA and the receptor include messenger RNA transcription, processing, and translation into specific proteins (see Chapter 5 for a review of protein synthesis). In general, the onset of action of thyroid and steroid hormones is slow compared with that of the water-soluble hormones described previously, and there is no amplification cascade. Changes in DNA transcription and protein synthesis occur over hours to days.
Hormone Regulation
Hormone Synthesis, Secretion, and Metabolism
Hormone synthesis and secretion may take place in several different cell types, but one site is generally considered to be the primary endocrine tissue and is responsive to feedback about the need for the hormone or its effects. For example, endothelial cells throughout the vascular system produce small amounts of angiotensin II (AII); however, the primary endocrine control is through the renin-angiotensin-aldosterone cascade in which specialized cells in the kidney trigger AII production in response to changes in extracellular volume and pressure.
Most endocrine hormones are polypeptides manufactured on the rough endoplasmic reticulum and stored in vesicles within the cells. A review of protein synthesis can be found in Chapter 5. The initial forms of the polypeptide hormones are usually larger molecules called prohormones or pre-prohormones. These must be cleaved by specific enzymes to release the active form of the hormone.1,3 Sometimes this cleavage occurs after the hormone is packaged into vesicles and the fragments are released into the bloodstream along with the hormone. In some cases, such as with insulin secretion, this is clinically useful as a marker of hormone synthesis. Insulin and its C-peptide fragment are produced in a 1:1 ratio and the concentration of C-peptide, which is more stable in the circulation, can be used as a measure of insulin production.
Because they are water-soluble, peptide hormones can be contained within the lipid bilayer of the vesicles and stored until a trigger results in exocytosis of the hormone into the extracellular space. Catecholamines are also water-soluble and stored in vesicles until they are released by exocytosis. However, they are formed by enzymes within the cytoplasm that begin with tyrosine and through a series of steps convert it first to dopamine; depending on the cell type, dopamine may be the final hormone or it can be converted to norepinephrine and then to epinephrine.
Steroid and thyroid hormones are not stored within vesicles because they are lipid-soluble and might leak from the vesicle. The strategy for synthesis and storage is much different for each of these hormones. When a steroid hormone is needed, increased production of the hormone closely precedes hormone release into the circulation.2 Steroid hormones are formed on demand from cholesterol that is either stored in the cell or retrieved from the circulating lipoproteins. The stimuli that trigger steroid secretion induce the enzymes in the pathway to synthesize the necessary hormones from cholesterol. Once formed, steroid hormones can simply diffuse through the plasma membrane and into the circulation. The strategy for trapping thyroid hormones within the thyroid gland until they are needed is quite different. Hormone synthesis precedes secretion by weeks or months. Triiodothyronine (T3) and thyroxine (T4) are synthesized in the thyroid follicle, bound to a protein called thyroglobulin.7 Thyroglobulin is a large water-soluble protein that is trapped within the thyroid follicle. Secretion occurs via cleavage of the thyroid hormone from thyroglobulin in response to systemic needs determined by the hypothalamus and the pituitary gland. Free T3 and T4 are released from the thyroid cells through membrane carrier proteins. Thyroid and steroid synthesis and release are detailed later in this chapter.
Factors Affecting Hormone Secretion
Secretion of endocrine hormones is dependent on the interplay of many factors. Most of the hormones controlled by the anterior pituitary gland are secreted in cyclical patterns. These cycles are regulated by the hypothalamus and may occur in response to feeding-fasting cycles, light-dark cycles, sleep-wake cycles, or in a 24-hour (circadian) period. Cycles also occur over longer periods (e.g., the 28-day menstrual cycle) or over years (e.g., the hormones that control reproductive differentiation and maturity).1,2 Acute systemic needs or stressors can partially override cyclical patterns and modify hormone secretion. An example of this is activation of the stress response that alters the normal circadian pattern of cortisol secretion. Knowledge of the cyclical nature of hormone release is important when comparing measured serum concentrations to normal ranges and when attempting to provide hormone replacement therapy that mimics the normal physiologic pattern.
Feedback Control of Secretion
The most common mechanism regulating hormone production and secretion is negative feedback control in which some aspect of the secreted hormone is sensed and regulates further secretion.4 An example of negative feedback is the interaction between the hypothalamic-pituitary system and the respective hormone-releasing glands controlled by this system. For example, thyrotropin-releasing hormone (TRH) is secreted by the hypothalamus when the central nervous system senses an inadequate supply of thyroid hormones in the circulation. TRH stimulates the synthesis and release of thyroid-stimulating hormone (TSH) from specific cells in the anterior pituitary gland; TSH then stimulates the synthesis and release of the thyroid hormones T3 and T4 from the thyroid follicle. The resulting increase in plasma thyroid hormone concentration exerts an inhibitory effect, or negative feedback, on the release of TSH by the anterior pituitary gland and TRH from the hypothalamus.7,8 The negative feedback loop is a mechanism for maintaining hormone activity within a normal range or set point of normal activity. The set point is genetically determined and influenced by age, gender, circadian cycles, and current internal and environmental conditions. The regulatory influences are multiple and complex, but often there is a particular variable that is influenced by the hormone’s activity and provides most of the feedback regulation. These feedback systems are detailed for the hormones of the pituitary system in later sections of this chapter. Knowledge of feedback controls is helpful when evaluating patients for endocrine diseases (see Chapter 40).
Hormone Metabolism and Excretion
The plasma concentration of a hormone depends not only on the rate of synthesis and release of the hormone but also on how rapidly the hormone is metabolized and excreted. Like other compounds, hormones are frequently degraded and excreted by the liver and kidneys. Water-soluble hormones may be excreted in the urinary filtrate. Lipid-soluble hormones, which are bound to plasma proteins and stored in adipose tissue, are less readily metabolized and remain in the circulation for a more prolonged period.4 In addition to metabolism by the kidneys and liver, hormones are often degraded by the target cell after binding to receptors. Half-life is a term used to describe the duration of hormone activity in the circulation and may be expressed in minutes, hours, or days. The half-life is the time for a hormone to reach half of its original concentration in the blood and is influenced by the rate of uptake by cells, degradation, and excretion.
Pharmacologic Hormone Concentrations
It is important to differentiate between physiologic and pharmacologic hormone activity. Physiologic hormone concentrations are extremely low in most cases, and pharmacologic levels of hormones are usually several-fold greater than would normally be secreted by endocrine tissues. Pharmacologic levels occur as a consequence of either pathologic processes or the administration of large doses of hormones. The tissue response to pharmacologic hormone concentrations may be significantly different from that caused by physiologic levels of hormones.4
Regulation of Receptor Responses
Tissue response to circulating hormone is only partially determined by the amount of hormone present in the circulation. Although virtually all body tissues are exposed to circulating hormones, specific hormones elicit a response only from certain cells and tissues. The ability of a cell to respond to a particular hormone depends on the presence of specific receptors for that hormone on or in the cell. Target cells are able to regulate their responsiveness to hormones by altering the receptor number, affinity, and efficiency of coupling to intracellular responses.3
Receptor Specificity and Affinity
The concept of receptor specificity is important for understanding the targeted responses of the endocrine system. Specificity refers to the molecular “fit” of a hormone within a receptor-binding pocket. Specificity cannot easily be separated from the concept of affinity. Affinity describes the degree of “tightness” of the hormone-receptor bond, or the inclination of the hormone to remain bound to the receptor. Specificity and affinity determine whether a cell with a receptor will respond to a hormonal stimulus and the hormone concentration at which the receptor effectively can bind the hormone. The potency of the hormone, or the amount of hormone required to elicit a cellular response, is dependent in part on the affinity of the receptor for a particular hormone. The higher the affinity of the receptor for a hormone, the lesser the amount of hormone needed to produce a response.3 Therefore, hormones that circulate in very minute amounts may have a large effect on cellular activity because of the tightness of the hormone-receptor bond.
“Cross-specificity” between hormones of similar structure may occur. For example, prolactin and growth hormone both can bind to the prolactin receptor, but with differing affinities. Under normal circumstances, the plasma concentration of growth hormone is not adequate to bind a significant number of prolactin receptors. However, in the condition called acromegaly, in which excessive concentrations of growth hormone lead to massive bone and tissue overgrowth, milk may be secreted from the mammary glands secondary to growth hormone stimulation of prolactin receptors.9,10
Receptor Down-Regulation and Up-Regulation
A factor determining the degree of response to a circulating hormone is the number of cell receptors available for hormone binding. When cells are exposed to high concentrations of hormone for a prolonged period, a common result is that the cell decreases the number of receptors. This phenomenon is known as down-regulation. An example of down-regulation occurs with insulin receptors in obese individuals. An increase in the plasma insulin level commonly occurs in obese individuals. This increased concentration of insulin does not result in an increase in cellular activities in most cells because the number of insulin receptors is down-regulated in response to the high plasma insulin concentration.3 Down-regulation probably serves a protective function: the cells are protected against excessive activity despite pathologic processes that cause excessive hormone levels. Up-regulation, or an increase in the number of receptors in response to chronically low hormone concentrations, may also occur. Up-regulation would make the cell more sensitive to the hormone, and hormone-dependent cellular activity could occur at normal or nearly normal levels despite a lower than normal hormone concentration.3
Another mechanism for altering hormone responsiveness is through regulation of the coupling mechanism between receptor activation and its intracellular responses.3 For example, the cytoplasmic domain of a receptor can be phosphorylated or otherwise altered so that it cannot effectively interact with its target G-protein. Additionally, enzymes such as phosphorylases, which remove phosphates from proteins, and phosphodiesterases, which degrade cyclic nucleotides, can be up-regulated or down-regulated to turn off the cellular responses more or less quickly. Many of these regulatory mechanisms are not fully understood.
Permissiveness
One effect that hormones may have on target cells is to increase the number of receptors for other hormones, thus enhancing the effect of the second hormone. This phenomenon is known as permissiveness. For example, one effect of thyroid hormone on adipose cells is to increase the number of receptors for epinephrine. When the adipose tissue is subsequently exposed to epinephrine, a greater release of fatty acids (used in providing energy for cellular processes) takes place than would occur in the absence of thyroid hormone.4 Permissiveness also allows cellular events to occur in sequence. One effect of estrogen, secreted early in the menstrual cycle, is to increase the number of uterine receptors for progesterone. The uterus is therefore sensitive to progesterone when it is present during the last part of the menstrual cycle, and normal proliferative changes take place.2,11
Hormone Agonists and Antagonists
To produce a cellular effect, a hormone must bind to the receptor and initiate a series of events that lead to a change in cellular activity. A chemical may bind to a receptor without initiating the typical intracellular changes; this chemical is described as a hormone antagonist or blocking agent. Agonists, on the other hand, bind hormone receptors and cause the same intracellular events that would occur with hormone-receptor binding. A large number of pharmacologic agents have agonist or antagonist effects on receptors for hormones. For example, hormone antagonists that compete with epinephrine and norepinephrine for receptor sites are frequently used to block the cardiac stimulatory properties of these hormones and thus decrease cardiac workload. Conversely, agonist medications that mimic hormone activity are frequently given as hormone replacement therapy in deficiency syndromes.