1
CHAPTER OUTLINE
■ ADDICTION: A DEVELOPMENTAL DISORDER
■ NEUROBIOLOGY OF DRUGS OF ABUSE
■ NEUROBIOLOGY OF DRUG ADDICTION
■ STRATEGIES TO COMBAT ADDICTION
■ SUMMARY
Drug addiction manifests as a compulsive drive to take a drug despite serious adverse consequences. This aberrant behavior has traditionally been viewed as a bad “choice” that is made voluntarily by the addicted person, a view that engendered the lingering stigma of addiction as a moral failure. However, addiction researchers have collected converging evidence showing that chronic drug abuse changes the brain in ways that can lead to the profound behavioral disruptions seen in addicted individuals. This is because drugs of abuse impact many neuronal circuits, including those involved in the processing of response to rewarding and aversive stimuli, interoception, emotions, decision making, and cognitive control, turning drug use into an automatic compulsive behavior. The fact that these changes are long-lasting, persisting even after years of drug use discontinuation, is what makes addiction a chronic and relapsing disease. The new knowledge about drugs’ effects on the brain and the modulatory role of genetic, developmental, and environmental factors should bring about changes in our approaches to the prevention and treatment of addiction.
Drugs, both legal (e.g., alcohol, nicotine) and illegal (e.g., cocaine, methamphetamine, heroin, marijuana) as well as abused psychotherapeutics (opioid analgesics, stimulant medications, benzodiazepines) can be abused for various reasons, including the experiencing of pleasure, altered mental states, improved performance, or, in certain instances, as an attempt to self-medicate a mental disorder. The repeated use of a psychoactive drug, in vulnerable individuals, can result in addiction, which is characterized by an intense desire for the drug combined with an impaired ability to control that urge, even in the face of well known adverse, even catastrophic consequences (e.g. incarceration, loss of child custody, loss of medical license).
It is important to emphasize, from the beginning, the difference between a state of addiction and a state of dependence. Physical dependence results in withdrawal symptoms when drugs, such as alcohol and heroin, are discontinued, but the adaptations that are responsible for these effects are different from those that underlie addiction and that are described in detail in this chapter. Partly because this distinction has often led to confusion, the fifth edition of the Diagnostic and Statistical Manual of Mental Disorders (DSM) has done away with the categories of substance abuse and dependence and uses instead the category of “addiction and related disorders.” This nomenclature strategy, which will include substance use disorders (with each drug identified in its own category along with its severity), is bound to better capture the dimensionality of the disease and the complex progression of neural and behavioral impairments that afflict addicted individuals.
A growing body of imaging evidence provides critical insights that help explain the aberrant behavioral manifestations that characterize addiction. The convergent results suggest that addicted individuals suffer from a progressive structural and functional disruption in brain regions that underlie the normal processes of motivation, reward, and inhibitory control (1,2). This provides a compelling rationale for the argument that drug addiction is a disease of the brain and that the associated abnormal behaviors (such as those associated with cocaine addiction or acute alcohol intoxication) are the result of dysfunctional brain tissue, just as cardiac insufficiency is a disease of the heart and abnormal blood circulation is the result of impaired myocardial function (3) (Fig. 1-1). Therefore, although initial drug experimentation and recreational use may be voluntary in most cases, once addiction develops, behavioral control becomes markedly disrupted. Importantly, even though imaging studies consistently show specific abnormalities in the brain function of addicted individuals, not all addicted individuals show these abnormalities, nor is the severity the same across all addicted subjects. The dimensional and heterogeneous nature of this disease has implications for its prevention and treatment and for public health policy and highlights the need for further research to delineate the nature and diversity of genetic, neurobiologic, and social factors involved in addiction.
FIGURE 1-1 Drug abuse and addiction as a disease of the brain. Images of the brain in a healthy control and in an individual addicted to cocaine drug (top panel), in an individual acutely exposed to placebo or alcohol (middle panel), and parallel images of the heart in a healthy control and in an individual with a myocardial infarction (bottom panel). The images were obtained with positron emission tomography (PET) and [18F]fluoro-2-deoxyglucose (FDG-PET) to measure glucose metabolism, which is a sensitive indicator of damage to the tissue in the brain and the heart. Note the decreased glucose metabolism in the orbitofrontal cortex (OFC) of the addicted person and the decreased metabolism in the myocardial tissue in the person with a myocardial infarct. Damage to the OFC will result in improper inhibitory control and compulsive behavior, and damage to the myocardium will result in improper blood circulation. Although abnormalities in the OFC are some of the most consistent findings in imaging studies of addicted individuals (including alcoholics), they are not detected in all addicted individuals. This implies that disruption of this frontal region is not the only mechanism that underlies the addictive process. (Heart images courtesy of H. Schelbert, University of California at Los Angeles. Images of glucose metabolism during alcohol intoxication reprinted from Volkow ND, Kim sw, Wang GJ, et al. Acute alcohol intoxication decreases glucose metabolism but increases acetate uptake in the human brain. Neuroimage 2013;64:277–283.)
Chronic intermittent exposure to an addictive substance is required for drug addiction to develop, a process that involves complex interactions between biologic and environmental factors (4). This helps explain why some individuals become addicted and others do not, and why attempts to understand addiction as a purely biologic or environmental disease have been largely unsuccessful. Recently, important discoveries have increased our knowledge of how drugs affect the epigenome, the expression patterns of specific genes, their protein products, neuronal communication and plasticity, and neural circuitry (5) and how these biologic factors might conflate to affect human behavior. This also sets the stage for a better understanding of how different environmental factors influence molecular traits (e.g., through epigenetic modification (6)) and contribute to patterns of behavior that facilitate the establishment of an addiction.
Here, we summarize how new methodologies that allow us to study genes, their products, the human brain, and its emergent properties are providing us with a greater understanding of drug addiction and their implications for prevention and treatment of addiction.
ADDICTION: A DEVELOPMENTAL DISORDER
Normal developmental processes might result in a higher risk of drug use at certain times in life than others. Experimentation often starts in adolescence, as does the process of addiction (7,8) (Fig. 1-2). Normal adolescent-specific behaviors (such as risk taking, novelty seeking, and heightened sensitivity to peer pressure) increase the likelihood of experimenting with legal and illegal drugs (9,10), which might reflect incomplete development of brain regions (e.g., myelination of frontal lobe regions) (11) that are involved in the processes of executive control and motivation. In addition, studies indicate that drug exposure during adolescence might result in different neuroadaptations from those that occur during adulthood. For example, adolescent rats exposed to nicotine undergo (a) significant changes in nicotine receptors and an increased reinforcement value for nicotine later in life (12); (b) enhanced novelty-induced activation in a conditioned place preference paradigm (13); and (c) short-term increase and lasting reduction in synaptic metabotropic glutamate receptor type 2 in the medial prefrontal cortex (PFC), leading to attention deficits later in adulthood (14). An important item for future studies will be to investigate whether the epigenetic priming mechanisms that make a mouse pre-exposed to chronic nicotine more sensitive to the reinforcement properties of cocaine (15) are different or more robust in adolescent animals. Similarly, recent studies demonstrated that the adolescent period is distinctly sensitive to long-term alteration by chronic ethanol exposure (16,17) and may explain the greater vulnerability to alcoholism in individuals who start using alcohol early in life (18).
FIGURE 1-2 Mean age at first use for specific illicit drugs among past year initiates aged 12 to 49, in 2011. (Data from the National Survey of Drug Use and Health (SAMHSA. Results from the 2011 National Survey on Drug Use and Health: Summary of National Findings and Detailed Tables. Substance Abuse and Mental Health Services Administration, Office of Applied studies, 2012. Ref. (8).
NEUROBIOLOGY OF DRUGS OF ABUSE
Many neurotransmitters, including GABA (γ-aminobutyric acid), glutamate, acetylcholine, dopamine (DA), serotonin, and endogenous opioids and cannabinoids, have been implicated in the effects of the various types of drugs of abuse. Of these, dopamine has been consistently associated with the reinforcing effects of most drugs of abuse. Drugs of abuse increase extracellular dopamine concentrations in limbic regions, including the nucleus accumbens (NAc) (19,20). Specifically, it seems that the reinforcing effects of drugs of abuse are due to their ability to surpass the magnitude and duration of the fast dopamine increases that occur in the NAc when triggered by natural reinforcers such as food and sex (21). Drugs such as cocaine, amphetamine, methamphetamine, and ecstasy increase dopamine in the synaptic space by inhibiting dopamine reuptake and/ or promoting the release of intravesicular dopamine into the cytoplasm (22–24). Other drugs, such as nicotine, alcohol, opioids, and marijuana, work directly or indirectly to modulate dopamine cell firing through their effects on nicotine, GABA, opioid, and cannabinoid receptors, respectively (25,26). In addition, alcohol has many nonreceptor, intracellular targets that can influence neurotransmitter release.
It is common to describe the effects of dopamine in the NAc as one signaling “reward,” but this traditional concept is an oversimplification (27). Rather, dopamine is a versatile modifier of motivation and a predictor of reward. Psychopharmacologic studies show that, depending on the magnitude and time course of dopamine-mediated neuronal activity, the system can encode different kinds of information to subcortical and cortical brain structures that convey different messages about stimulus–response, approach behavior, learning, and decision making (28–30). For example, abrupt and large DA increases relate to reward-predicting stimuli, while slower responses inform about any uncertainty related to that reward. Slow responses that consist mostly of neuronal depression react to aversive events. Interestingly, imaging studies with cocaine-addicted individuals have shown not only the expected, drug-induced fast DA increases in striatum (including NAc) associated with the drug’s rewarding effect but also that such increases are markedly blunted compared with controls (31). The same addicted subjects, however, show significant DA increases in striatum in response to drug-conditioned cues that are associated with self-reports of drug craving and appear to be of a greater magnitude than the DA responses to the drug. We postulate that the discrepancy between the expectation for the drug effects (conditioned responses) and the blunted pharmacologic effects maintains drug taking in an attempt to obtain the expected reward. Also, whether tested during early or protracted withdrawal, addicted subjects show lower levels of dopamine D2 receptors in striatum (including NAc), which are associated with decreases in baseline activity in frontal brain regions implicated in salience attribution (orbitofrontal cortex [OFC]) and inhibitory control (anterior cingulate gyrus [CG]), whose disruption results in compulsivity and impulsivity. These results point to an imbalance between dopaminergic circuits that underlie reward and conditioning and those that underlie executive function (emotional control and decision making), an imbalance that we postulate contributes to the compulsive drug use and loss of control in addiction.
For example, increases in dopamine are likely to play a role in the prediction of error that is so important for stimulus-reward learning (32) and to the assignment of salience (29). Salience refers to stimuli or environmental changes that are arousing or that elicit an attentional–behavioral switch (33). Salience, which applies not only to reward but also to aversive, new, or unexpected stimuli, affects the motivation to seek the anticipated reward and facilitates conditioned learning (34,35). This provides a different perspective about drugs, because it implies that drug-induced increases in dopamine will inherently motivate further procurement of more drug (regardless of whether or not the effects of the drug are consciously perceived to be pleasurable). Indeed, some addicted individuals report that they seek the drug even though its effects are no longer pleasurable. Drug-induced increases in dopamine will also facilitate conditioned learning, so previously neutral stimuli that are associated with the drug become salient. These previously neutral stimuli then increase dopamine by themselves and elicit the desire for the drug (36). This explains why the addicted person is at risk of relapsing when exposed to an environment where he or she has previously taken the drug.
It is worth noting in this context the emergence of a powerful new tool for the precise manipulation of neuronal activation in space and time (i.e., optogenetic techniques), which offers unprecedented access to the circuitry associated with drug taking and relapse as well as the circuitry that opposes the prepotent tendencies to use the drug in rodent models of addiction. The results of this research so far point to a highly complex constellation of heterogeneous dopamine neurons in the instantiation of reward, aversion, and salience and of glutamatergic projections from PFC amygdala and hippocampus that modulate DA neuron firing and DA release (37).
Another important question can be posed as follows: If natural reinforcers increase dopamine, why would they not lead to addiction? The difference might be due to qualitative and quantitative differences in the increases in dopamine induced by drugs, which are greater in magnitude (by at least 5- to 10-fold as measured with microdialysis) and duration than are those induced by natural reinforcers (21). In addition, whereas the dopamine increases produced by natural reinforcers in the NAc undergo habituation, those induced by drugs of abuse do not (19). The nondecremental dopamine stimulation of the NAc from repeated drug use strengthens the motivational properties of the drug, an effect that is not seen typically with natural reinforcers (food being a special case being discussed in a later section and elsewhere in more detail) (38).
NEUROBIOLOGY OF DRUG ADDICTION
Drug and alcohol addiction have been conceptualized as a reward deficit disorder (39). More specifically, a defining characteristic of drug addiction is the transition from impulsive drug intake to compulsive intake that is mediated by positive and negative reinforcement, respectively. Once a person has transitioned to compulsive drug use, negative reinforcement mechanisms play a substantial role in continued, escalated drug use. Negative reinforcement is a behavioral mechanism whereby increased drug taking is strengthened by the alleviation of a negative emotional state that is precipitated by the absence of the drug. In recent years, attention has focused on understanding the neurobiologic mechanisms, including specific neuroadapatations that underlie this negative emotional state produced by drug withdrawal and abstinence because of its central role in compulsive drug use. Neuroadaptations in the brain reward and stress systems seem to be key in driving compulsion to continue drug intake despite adverse consequences. Decreases of dopamine and GABA in the ventral striatum are coupled with enhancement of corticotropin-releasing factor (CRF) in the extended amygdala as well as blunting of the hypothalamic–pituitary–adrenal axis. In addition, engagement of the dorsal striatum during addiction is thought to help to solidify habitual behaviors associated with drug seeking and taking. Namely, neuroadaptations in the dorsal striatum involve changes in glutamate, GABA, and the endocannabinoid system (40,41). Finally, compulsive drug consumption involves poor inhibitory control and poor executive functioning, which are mediated by prefrontal cortical regions of the brain. For example, for alcohol, regions of the PFC are selectively damaged by chronic intermittent use and result in poor decision making that can perpetuate the addiction cycle. However, adaptations also seem to occur in the mesolimbic circuit (including the NAc, amygdala, and hippocampus), which probably cause the enhanced saliency value of the drug and drug stimuli, and the decreased sensitivity to natural reinforcers (5). Finally, there is also evidence pointing to impairments in ancillary circuits that are likely to contribute to the stereotypic behaviors seen in addicted individuals. For example, insular dysfunctions can affect the ability to properly evaluate internal states (42), while impairments in the lateral habenula can compromise the ability to properly process and learn from disappointments and might disrupt mood (43). Taken together, neuroadaptations in the brain reward, stress, habit formation, and executive functioning centers coalesce to drive compulsive alcohol intake.
At a cellular level, drugs have been reported to alter the expression of certain transcription factors (nuclear proteins that bind to regulatory regions of genes, thereby regulating their transcription into mRNA), as well as a wide variety of proteins involved in neurotransmission in several key brain regions. There is growing evidence suggesting that epigenetic mechanisms mediate many of the drug-induced changes in gene expression patterns that lead to structural, synaptic, and behavioral plasticity in the brain (44). The dynamic and often long-lasting changes that occur in the transcription factors AFosB, cAMP-responsive element-binding protein (CREB), and nuclear factor kappa B after chronic drug administration are of particular interest because they appear to modulate the synthesis of proteins involved in key aspects of the addiction phenotype, such as synaptic plasticity (45). Indeed, chronic drug exposure can alter the morphology of neurons in dopamine-regulated circuits. For example, in rodents, chronic cocaine, alcohol, or amphetamine administration alter neuronal dendritic branching and spine density in the NAc and PFC—an adaptation that is thought to play a role in the enhanced incentive motivational value of the drug (a process that results in increased “wanting” in contrast to just “liking” the drug) in addiction (46–48).
At the neurotransmitter level, addiction-related adaptations have been documented not only for dopamine but also for glutamate, GABA, opiates, serotonin, and various neuropeptides (49). These changes contribute to the abnormal function of brain circuits. For example, in individuals who are addicted to cocaine, imaging studies have documented that disrupted dopamine activity in the brain (shown by reductions in dopamine D2 receptors in striatum) is associated with reduced baseline activity in the OFC and in the anterior CG—brain regions that are involved in salience attribution and inhibitory control (50) (Fig. 1-3). Abnormal function of these cortical regions has been particularly revealing in furthering our understanding of addiction, because their disruption is linked to compulsive behavior (OFC) and disinhibition (CG) (50). The combined research of the last decade reveals that drug-induced impairments in the PFC areas exert a twofold impact on addiction, first through its perturbed regulation of limbic reward regions and second through its involvement in higher-order executive function (e.g., self-control, salience attribution, and awareness) (52). Therefore, the abnormalities in these frontal regions could underlie both the compulsive nature of drug administration in addicted individuals and their inability to control their urges to take the drug when they are exposed to it (53). They are also likely to contribute to the impaired judgment and cognitive deficits seen in many addicted individuals. In addition, animal studies have shown that drug-related adaptations in these frontal regions result in enhanced activity in the glutamatergic pathway that regulates dopamine release in the NAc (54). The adaptations in this pathway seem to play a role in the relapse that occurs after drug withdrawal in animals previously trained to self-administer a drug when they are again exposed to the drug, a drug stimulus, or stress (54).
FIGURE 1-3 Dopamine D2 receptors and glucose metabolism in addiction. A, B: positron emission tomography (PET) images showing dopamine D2 receptors and brain glucose metabolism in the OFC (orbitofrontal cortex) in controls (A) and in individuals who abuse cocaine (B). Note that the individuals abusing cocaine have reductions in both D2 receptors and in OFC metabolism. C: correlation between measures of D2 receptors and brain glucose metabolism in the OFC and anterior cingulate gyrus (CG) of both cocaine and methamphetamine abusers. The lower the D2-receptor expression, the lower the metabolism in the OFC and CG. Decreased activity in the OFC, a brain region that is implicated in salience attribution and whose disruption results in compulsive behavior, could underlie the compulsive drug administration that occurs in addiction. Decreased activity in the CG, a brain region that is involved in inhibitory control, could underlie the inability to restrain from taking the drug when the addicted person is exposed to it Volkow ND, Fowler JS, Wang GJ, et al. Dopamine in drug abuse and addiction: results of imaging studies and treatment implications. Arch Neurol 2007;64:1575–1579 Ref. (51)
Finally, in addition to classic neurotransmitter systems, recent studies link neuroinflammatory signaling in the brain to drug abuse and addiction. For example, central immune signaling activation is associated with the abuse of alcohol, opioids, cocaine, and methamphetamine (55). Altered neuroimmune signaling regulates alcohol drinking behavior and may contribute to alcohol-induced negative affect and depression-like behaviors (56,57).
VULNERABILITY TO ADDICTION
Genetic Factors
It is estimated that 40% to 60% of the vulnerability to addiction is attributable to genetic factors (58). In animal studies, several genes have been identified that are involved in drug responses, and their experimental modifications markedly affect drug self-administration (59). In addition, animal studies have identified candidate genes and genetic loci for alcohol responses that overlap with genes and loci identified in human studies (60). For example, genes on mouse chromosome 1 and human chromosome 1q are associated with alcohol withdrawal responses. Genome-wide association studies (GWAS), which interrogate all of the common genetic variants for correlation with alcohol phenotypes, have proven to be a useful approach to identify novel variants (61). Case in point, a GWAS for alcohol consumption identified the autism susceptibility candidate 2 (AUTS2) gene in a large population-based sample (62). In addition, a family-based GWAS of frontal theta oscillations, an endophenotype for alcoholism, identified a potassium channel gene, KCNJ6 as responsible for a significant amount of variations in that measure (63). Progress in identifying candidate genes for alcoholism and alcohol-related responses continues at a rapid pace (64). However, identifying the biologic function of these new candidate genes will be a major challenge in the next decade. The hope is that a better understanding of the myriad interacting genetic factors and networks that influence addiction risk and trajectory will help increase the efficacy of addiction treatments and reduce the likelihood of relapse (65). A key example of moving from gene identification to biologic function is exemplified by the association between drug-metabolizing genes and protection against alcohol dependence. Some of these polymorphisms interfere with drug metabolism, influencing the amount of time a drug circulates through the body. For example, specific alleles for the genes that encode alcohol dehydrogenases ADH1B and ALDH2 (enzymes involved in the metabolism of alcohol) are reportedly protective against alcoholism (66). Similarly, polymorphisms in the gene that encodes cytochrome P-450 2A6 (an enzyme that is involved in nicotine metabolism) are reportedly protective against nicotine addiction (67). Furthermore, genetic polymorphisms in the cytochrome P-450 2D6 gene (an enzyme that is involved in conversion of codeine to morphine) seem to provide a degree of protection against codeine abuse (68). These polymorphisms in drug-metabolizing genes operate by modulating the accumulation of toxic metabolites that are aversive; therefore, if alcohol or drugs are consumed by individuals who carry variants that convert their substrate at high rates, then the accumulation of toxic metabolites serves as a negative stimulus to prevent further consumption.
Some polymorphisms in receptor genes that mediate drug effects have also been associated with a higher risk of addiction. For example, a number of convergent results support a CHRNA5/A3/B4 gene cluster association with nicotine dependence (69–72) and with the risk of such smoking-related diseases as lung cancer and peripheral arterial disease (73). An association has also been found between alcohol dependence and the genes for the GABA type A (GABAA) receptors GABRG3 (74) and GABRA2 (75). D2-receptor polymorphisms have been linked to a higher vulnerability to drug addiction, although some studies have failed to replicate this finding (58). Also, of particular interest in this context are the findings related to the association between DRD4 VNTR polymorphisms on one hand and attention deficit hyperactivity disorder (ADHD), personality traits influencing risk taking, and addiction and addiction-related phenotypes on the other (76). DRD4’s likely involvement in addiction trajectories is potentially very important in light of its alleged ability to moderate the impact of environments on behavior and health (77). Replication of many of the genetic findings in substance abuse and addiction is still pending. However, there has been some progress in replicating findings from the Collaborative Study on the Genetics of Alcoholism (COGA) (78). Finally, exome sequencing is being employed to identify novel candidate genes for alcoholism. By sequencing all of the protein-coding regions of the genome, exome sequencing will identify variants that will likely have a direct role in altering the function of the corresponding protein.
Environmental Factors
Environmental factors that have been consistently associated with a propensity to self-administer drugs include low socioeconomic class, poor parental support, within–peer group deviancy, and drug availability. Stress might be a common feature in a wide variety of environmental factors that increase the risk for drug abuse. The mechanisms responsible for stress-induced increases in vulnerability to drug use and to relapse in people who are addicted are not yet well understood. However, there is strong evidence that dysregulation of the stress-responsive neuropeptide CRF system can lead to a variety of psychiatric disorders, including substance use disorders (79), likely through its effects in the amygdala and the pituitary–adrenal axis (80). A recent study shows that social isolation during a critical period of adolescence increases addiction vulnerability (81). In addition, social isolation in adolescence increases anxiety and alcohol intake (82).
Imaging techniques now allow us to investigate how environmental factors affect the brain and how these, in turn, affect the behavioral responses to drugs of abuse. For example, in nonhuman primates, social status affects D2-receptor expression in the brain, which in turn affects the propensity for cocaine self-administration (83). Animals that achieve a dominant status in the group show increased numbers of D2 receptors in the striatum and are reluctant to administer cocaine, whereas animals that are subordinate have lower D2-receptor numbers and readily administer cocaine. Because animal studies have shown that increasing D2 receptors in NAc markedly decreases drug consumption (which has been shown for alcohol) (84), this could provide a mechanism by which a social stressor could modify the propensity to self-administer drugs.
Long-lasting changes in gene expression induced by an environmental event such as drug or alcohol exposure are now being studied as a means to identify how the environment can contribute to drug and alcohol addiction. These long-lasting changes in gene expression are mediated by epigenetic mechanisms including DNA methylation, histone modification, and microRNAs. For example, the acute anxiolytic effects of alcohol in rats were associated with a decrease in histone deacetylase (HDAC) activity and an increase in acetylation of histones H3 and H4. In addition, CREB-binding protein (CBP) and neuropeptide Y (NPY) expression levels were increased in the amygdala, a major brain region implicated in stress and anxiety. Conversely, the anxiety-like behaviors during withdrawal after chronic alcohol exposure were highly correlated with an increase in HDAC activity and decreases in acetylation of H3 and H4 and levels of CBP and NPY in the amygdala (85). Treatment of rats with trichostatin A (HDAC inhibitor) reversed the deficits in H3, H4, and NPY expression and prevented the development of alcohol withdrawal–related anxiety as measured by the elevated plus maze and light/dark box exploration tests. Based on the effect of trichostatin A, the authors suggested the possibility that neuroadaptations in the amygdala during chronic alcohol exposure may involve both histone acetyltransferases and HDACs in the dynamic process of chromatin remodeling (85).
An increasingly relevant example of an environmental factor negatively impinging upon brains hardwired to respond and seek immediate rewards can be found in the ubiquitous availability of high-calorie content (junk) food, which can hijack deeply entrenched (evolved) homeostatic mechanisms to easily override inhibitory controls in vulnerable individuals and facilitate obesogenic behaviors (38). A similarly deleterious relationship between increased availability and negative public health impact may also play a role in the increased nonmedical use of stimulant (ADHD) medications (86,87), the relationship between rates of opioid analgesics’ prescription and overdose death rates (88,89), and the steady increase in marijuana use among young people (90).
Comorbidity with Mental Illness
The risk for substance abuse and addiction in individuals with mental illness is significantly higher than for the general population (91). The high comorbidity probably reflects, in part, overlapping environmental, genetic, and neurobiologic factors that influence drug abuse and mental illness (92–94).
Alcoholism also often presents in combination with abuse of other drugs and with psychiatric disorders including mood, anxiety, sleep, and psychotic disorders. Among alcohol-dependent individuals, nearly 40% have at least one lifetime psychiatric diagnosis and more than 20% have another drug abuse disorder. Almost 30% of people with psychiatric disorders exhibit substance abuse with 25% abusing alcohol and 15% abusing other drugs. These cooccurrences are problematic because they can complicate treatment and lead to synergistic negative health effects that are worse than either disorder alone. For example, depression can deplete patients of the motivation required to maintain recovery from alcohol abuse, depressed alcohol-dependent individuals demonstrate 59% more severe suicidal symptoms than do depressed nondependent individuals, and depression is predictive of relapse to drinking.
It is likely that different neurobiologic factors are involved in comorbidity depending on the temporal course of its development (i.e., mental illness followed by drug abuse or vice versa). In some instances, the mental illness and addiction seem to co-occur independently (95), but in others, there might be a sequential dependency. It has been proposed that comorbidity might be due to the use of the abused drugs to self-medicate the mental illness in cases in which the onset of mental illness is followed by abuse of some types of drug. But, when drug abuse is followed by mental illness, the chronic exposure could lead to neurobiologic changes, which might explain the increased risk of mental illness (96). For example, the high prevalence of smoking that is initiated after individuals experience depression could reflect, in part, the antidepressant effects of nicotine as well as the antidepressant effects of monoamine oxidase A and B inhibition by cigarette smoke (97). On the other hand, the reported risk for depression with early drug abuse (98) could reflect neuroadaptations in dopamine systems that might make individuals more vulnerable to depression. Also in this category are the multiple observations suggesting that cannabis exposure may be a “component cause” that, in combination with other factors, like preexisting/genetic vulnerabilities could contribute to schizophrenia or other psychotic disorders (99).
The higher risk of drug abuse in individuals with mental illnesses highlights the relevance of the early evaluation and treatment of mental diseases as an effective strategy to prevent drug addiction that starts as self-medication.
STRATEGIES TO COMBAT ADDICTION
The knowledge of the neurobiology of drugs and the adaptive changes that occur with addiction is guiding new strategies for prevention and treatment and identifying areas in which further research is required.
Preventing Addiction
The greater vulnerability of adolescents to experimentation with drugs of abuse and to subsequent addiction underscores why prevention of early exposure is such an important strategy to combat drug addiction. Epidemiologic studies show that the prevalence of drug use in adolescents has changed significantly over the past 30 years, and some of the decreases seem to be related to education about the risks of drugs. For example, for marijuana, the prevalence rates of use in the United States in 1979 were as high as 50%, whereas in 1992, they were as low as 20% (100) (Fig. 1-4). This changing pattern of marijuana use seems to be related in part to the perception of the risks associated with the drug; when adolescents perceived the drug to be risky, the rate of use was low, whereas when they did not, the rate of use was high. Similarly, the significant decreases in ecstasy use as well as cigarette smoking in adolescents (100) seem to partly reflect effective educational campaigns. These results show that, despite the fact that adolescents are at a stage in their lives when they are more likely to take risks, interventions that educate them about the harmful effects of drugs with age-appropriate messages can decrease the rate of drug use (101–103). However, not all media campaigns and school-based educational programs have been successful (104,105). Tailored interventions that take into account socioeconomic, cultural, and age and gender characteristics of children and adolescents are more likely to improve the effectiveness of the interventions.
FIGURE 1-4 Use and risk perception of marijuana. The prevalence rate for marijuana use in the past 12 months and the perception of marijuana as a dangerous drug in 12th graders (18 to 19 years old) between 1975 and 2012 (From Johnston LD, O’Malley PM, Bachman JG, et al. Monitoring the future national survey results on drug use. 2012 Overview. Publication No. 07-6205. Bethesda, MD; National institutes of Health, 2012.). When teenagers perceived marijuana as dangerous, the prevalence of drug use was low and vice versa.
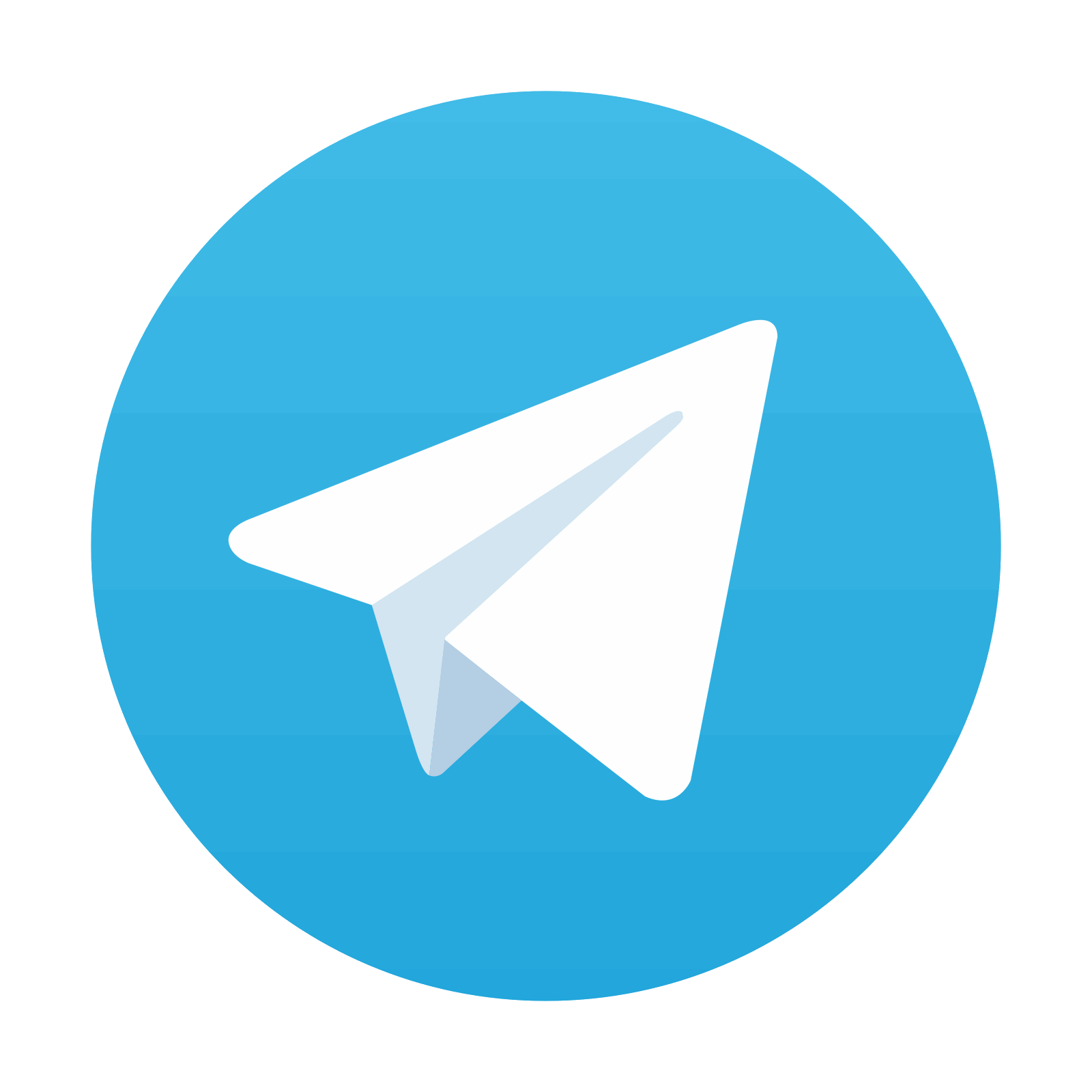
Stay updated, free articles. Join our Telegram channel

Full access? Get Clinical Tree
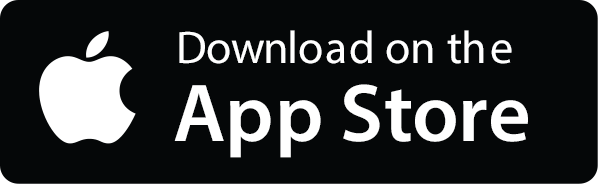
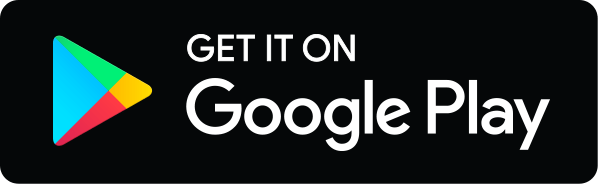