Introduction
The function of the immune system is to protect the host from invasion of foreign organisms by distinguishing “self” from “non-self.” Such a system is necessary for survival. A well-functioning immune system not only protects the host from external factors such as microorganisms or toxins but also prevents and repels attacks by endogenous factors such as tumors or autoimmune phenomena. A normal immune response relies on the careful coordination of a complex network of biological factors, specialized cells, tissue, and organs necessary for the recognition of pathogens and subsequent elimination of foreign antigens. Dysfunction or deficiency of components of the immune system leads to a variety of clinical diseases of varying expression and severity, ranging from atopic disease to rheumatoid arthritis, severe combined immunodeficiency, and cancer. This chapter introduces the intricate physiology of the immune system and abnormalities that lead to diseases of hypersensitivity and immunodeficiency.
Normal Structure & Function of the Immune System
The immune system consists of both antigen-specific and nonspecific components that have distinct yet overlapping functions. The antibody-mediated and cell-mediated immune systems provide specificity and memory of previously encountered antigens. The nonspecific or innate defenses include epithelial barriers, mucociliary clearance, phagocytic cells, and complement proteins. Despite their lack of specificity, these components are essential because they are largely responsible for natural immunity to a vast array of environmental threats and microorganisms. Knowledge of the components and physiology of normal immunity is essential for understanding the pathophysiology of diseases of the immune system.
The major cellular components of the immune system consist of monocytes and macrophages, lymphocytes, and the family of granulocytic cells, including neutrophils, eosinophils, and basophils. Derived from hematopoietic stem cells, these fully differentiated effector cells have membrane receptors for various chemoattractants and mediators, facilitating the activation or destruction of target cells.
Mononuclear phagocytes play a central role in the immune response. Tissue macrophages are derived from blood monocytes and participate in antigen processing, tissue repair, and secretion of mediators vital to initiation of specific immune responses. These cells, abundant near mucosal surfaces that internalize microorganisms and debris, travel to secondary lymphoid organs where they process and present that antigen in a form recognizable to T lymphocytes. In addition, they function as effector cells for certain types of tumor immunity. Circulating monocytes are recruited to sites of inflammation where they mature into macrophages. Both monocytes and macrophages contain receptors for C3b (activated bound complement) and the Fc portion of both immunoglobulin G (IgG) and IgE, which facilitate the activation of these cells through antigen-specific and nonspecific immune pathways. Activation of these cells occurs both after binding to immune complexes through exposure to various cytokines and after phagocytosis of antigen or particulates such as silica and asbestos. Proteolytic enzymes and proinflammatory mediators including cytokines, arachidonic acid metabolites, and oxidative metabolites are utilized in the monocytes and macrophages. Macrophages constitutively express toll-like receptor 4 (TLR4), which can bind bacterial endotoxin, triggering cytokine release and bridging innate and adaptive immune responses. It is hypothesized that macrophage-derived interleukin 12 (IL-12) and tumor necrosis factor (TNF) influence TH1 and TH2 differentiation, thereby affecting the expression of atopy and allergic disease. Many epithelial dendritic cells (eg, Langerhans cells, oligodendrocytes, Kupffer cells) may share a common hematopoietic precursor and function to process and transport antigen from skin, respiratory, and gastrointestinal (GI) surfaces to regional lymphoid tissues.
ADA | Adenosine deaminase |
ADCC | Antibody-dependent cell-mediated cytotoxicity |
AIDS | Acquired immunodeficiency syndrome |
APC | Antigen-presenting cell |
ART | Antiretroviral therapy |
BCR | B-cell receptor |
BTK | Bruton tyrosine kinase |
C1, C2, etc | Complement factor 1, complement factor 2, etc |
cAMP | Cyclic adenosine monophosphate |
CCR5 | CC-subfamily chemokine receptor 5 |
CD | Clusters of differentiation |
CD4 | Helper T-cell subset |
CD8 | Cytotoxic T-cell subset |
CGD | Chronic granulomatous disease |
CMV | Cytomegalovirus |
CNS | Central nervous system |
CTL | Cytotoxic lymphocyte |
CVID | Common variable immunodeficiency |
CXCR5 | CXC-subfamily chemoreceptor 5 |
F(ab) | Antigen-binding fragment |
Fc | Crystallizable fragment |
FcεRI | High-affinity IgE receptor |
FcγR | Fc gamma receptor |
FOXP3 | Forkhead box P3 |
GALT | Gut-associated lymphoid tissue |
GM-CSF | Granulocyte-macrophage colony-stimulating factor |
H1, H2, H3 | Histamine receptor type 1, 2, 3 |
HBV | Hepatitis B virus |
HCV | Hepatitis C virus |
HIV | Human immunodeficiency virus |
HPV | Human papillomavirus |
HSV | Herpes simplex virus |
HZV | Herpes zoster virus |
ICAM-1 | Intercellular adhesion molecule-1 |
IFN-γ | Interferon-γ |
Ig | Immunoglobulin |
IVIG | Intravenous immunoglobulin |
IL-1, IL-2, etc | Interleukin-1, interleukin-2, etc. |
JAK | Janus kinase |
JC | Jakob-Creutzfeldt |
LAK cell | Lymphokine-activated killer cell |
LPS | Lipopolysaccharide |
LT | Leukotriene |
MAC | Mycobacterium avium complex |
MBP | Major basic protein |
MHC | Major histocompatibility complex |
MSMD | Mendelian susceptibility to mycobacterial disease |
NADPH | Nicotinamide adenine dinucleotide phosphate |
NHL | Non-Hodgkin lymphoma |
NK | Natural killer cells |
PAF | Platelet-activating factor |
PCP | Pneumocystis pneumonia |
PGD | Prostaglandin D |
PNP | Purine nucleoside phosphorylase |
PTK | Protein tyrosine kinase |
RAG | Recombination-activating gene |
RANTES | Chemokine regulated on activation normal T expressed and secreted |
RAST | Radioallergosorbent test |
SCID | Severe combined immunodeficiency disease |
STAT | Signal transducer and activator of transcription |
TACI | Transmembrane activator and calcium modulator and cyclophilin ligand interactor |
TAME | N-α-p-tosyl-L-arginine methylester-esterase |
TCR | T-cell receptor |
TH1 | Helper T 1 subset |
TH2 | Helper T 2 subset |
TH17 | Helper T subset secreting IL-17 |
Treg | Helper T subset with regulatory function |
TGF-β | Transforming growth factor beta |
TLR | Toll-like receptor |
TNF | Tumor necrosis factor |
TSH | Thyroid-stimulating hormone |
TX | Thromboxane |
VCAM-1 | Vascular cell adhesion molecule-1 |
VIP | Vasoactive intestinal peptide |
XLA | X-linked agammaglobulinemia |
XSCID | X-linked severe combined immunodeficiency disease |
ZAP-70 | Protein tyrosine kinase ZAP-70 |
Lymphocytes are responsible for the initial specific recognition of antigen. They are functionally and phenotypically divided into B and T lymphocytes. Structurally, B and T lymphocytes cannot be distinguished visually from each other under the microscope; they can be enumerated by flow cytometric phenotyping or by immunohistochemical methods. Approximately 70–80% of circulating blood lymphocytes are T cells (CD3) and 10–15% are B cells (CD19); the remainder are referred to as natural killer (NK) cells (CD56, CD161, also known as NK cells or null cells).
The thymus-derived cells (T lymphocytes or T cells) are involved in cellular immune responses. B lymphocytes or B cells are involved in humoral or antibody responses. Precursors of T cells migrate to the thymus, where they develop some of the functional and cell surface characteristics of mature T cells. Through positive and negative selection, clones of autoreactive T cells are eliminated, and mature T cells migrate to the peripheral lymphoid tissues. There, they enter the pool of long-lived lymphocytes that recirculate from the blood to the lymph.
T lymphocytes are heterogeneous with respect to their cell surface markers and functional characteristics. Numerous subpopulations of T cells are now recognized. Helper-inducer T cells (CD4) help to amplify B-cell production of immunoglobulin and amplify T-cell (CD8)–mediated cytotoxicity. Activated CD4 T cells regulate immune responses through cell-to-cell contact and by elaboration of soluble factors or cytokines.
Subsets of CD4 T cells can be identified on the basis of their pattern of cytokine production. TH1 cells develop in the presence of IL-12, secreted from activated macrophages, especially in the presence of infection with intracellular microbes. TH1 cells elaborate interferon-γ (IFN-γ) and TNF but not IL-4 and IL-5. They participate in cellular immune responses to intracellular pathogens and type IV delayed hypersensitivity reactions. TH2 cells develop in the presence of IL-4 and secrete IL-4, IL-5, and IL-13, which facilitate humoral responses. Because IL-4 and IL-13 promote IgE production and IL-5 is an eosinophil proliferation and differentiation factor, TH2 cells have been implicated in response to allergens and helminthes.
Cytotoxic or “killer” T cells (CTLs) are generated after mature T cells interact with certain foreign antigens. They are responsible for defense against intracellular pathogens (eg, viruses), tumor immunity, and organ graft rejection. Most killer T cells express the CD8 phenotype, although in certain circumstances, CD4 T cells can be cytotoxic. CTLs may kill their target through osmotic lysis, by secretion of TNF, or by induction of apoptosis, that is, programmed cell death.
A number of additional T-helper subsets have been discovered that contribute to immune regulation. Mucosal dendritic cells control the generation of regulatory T cells, which modulate inflammatory responses through the secretion of regulatory cytokines. TH17 cell subsets appear to boost early phagocytic cell responses by recruiting neutrophils to sites of acute inflammation via elaboration of IL-17 and may play a role in autoimmune diseases. Treg cells express high-affinity receptors for IL-2 (CD25) and FOXP3, a transcription factor that may suppress autoimmune disease. Treg cells are inhibitory, suppressing activated T effector cells by their secretion of transforming growth factor-β (TGF-β), modulating responses to antigen, thereby regulating homeostasis and tolerance versus inflammation, allergy, and autoimmunity. Mutations of FOXP3 have been associated with inflammatory autoimmune disease, immune dysregulation, polyendocrinopathy, and X-linked syndrome (IPEX).
B-lymphocyte maturation proceeds in antigen-independent and antigen-dependent stages. Antigen-independent development occurs in the marrow where pre-B cells mature into immunoglobulin-bearing naive B cells (cells that have not been exposed to antigen previously). In peripheral lymphoid tissues, antigen-dependent activation produces circulating long-lived memory B cells and plasma cells found predominantly in primary follicles and germinal centers of the lymph nodes and spleen. All mature B cells bear surface immunoglobulin that is their antigen-specific receptor. The major role of B cells is differentiation to antibody-secreting plasma cells. However, B cells may also release cytokines and function as antigen-presenting cells (APCs).
Null cells probably include a number of different cell types, including a group called NK cells. These cells appear distinct from other lymphocytes in that they are slightly larger, with a kidney-shaped nucleolus, have a granular appearance (large granular lymphocytes), express distinct cell surface markers (CD56, CD161), but lack antigen-specific T-cell receptors (CD3, or TCRs). Recruited to sites of inflammation, NK cells possess membrane receptors for the IgG molecules (FcγR), facilitating antibody-dependent cell-mediated cytotoxicity (ADCC). Binding of an antibody-coated cell or foreign substance triggers release of perforin, a pore-forming protein that causes cytolysis. Other NK-cell functions include antibody-independent cellular killing, induction of apoptosis in Fas-expressing cells, and immunomodulation of responses to viruses, malignancy, and transplanted tissue through a potent release of IFN-γ, TNF, and other key cytokines.
Polymorphonuclear leukocytes (neutrophils) are granulocytes that phagocytose and destroy foreign antigens and microbial organisms. They are attracted to the site of antigen by chemotactic factors, including plasma-activated complement 5 (C5a), leukotriene B4 (LTB4), granulocyte colony-stimulating factor (G-CSF), granulocyte-macrophage colony-stimulating factor (GM-CSF), IL-8, and platelet-activating factor (PAF). The presence of receptors for complement C3b and invariant/constant regions of IgG molecules (Fcγ) on the surface of neutrophils also facilitates the clearance of opsonized microbes through the reticuloendothelial system. Smaller antigens are phagocytosed and destroyed by lysosomal enzymes. Locally released lysosomal enzymes destroy particles too large to be phagocytosed. Neutrophils contain or generate a number of antimicrobial factors, including oxidative metabolites, superoxide, and hydrogen peroxide, as well as myeloperoxidase, which catalyzes the production of hypochlorite, and proteolytic enzymes, including collagenase, elastase, and cathepsin B.
Eosinophils are often found in inflammatory sites or at sites of immune reactivity and play a crucial role in the host’s defense against parasites. Despite many shared functional similarities to neutrophils, eosinophils are considerably less efficient than neutrophils at phagocytosis. Eosinophils play both a proactive and a modulatory role in inflammation. They are attracted to the site of the antigen-antibody reactions by PAF, C5a, chemokines, histamine, and LTB4. They are important in the defense against parasites. When stimulated, they release numerous inflammatory factors, including major basic protein (MBP), eosinophil-derived neurotoxin, eosinophil cationic protein (ECP), eosinophil peroxidase, lysosomal hydrolases, and LTC4. MBP destroys parasites, impairs ciliary beating, and causes exfoliation of respiratory epithelial cells; it may trigger histamine release from mast cells and basophils. Eosinophil-derived products may play a role in the development of airway hyperreactivity.
Basophils play an important role in both immediate- and late-phase allergic responses. These cells release many of the potent mediators of allergic inflammatory diseases, including histamine, leukotrienes (LTs), prostaglandins (PGs), and PAF, all of which have significant effects on the vasculature and on the inflammatory response. Basophils are present in the circulation, possess high-affinity receptors for IgE (FcεRI), and mediate immediate hypersensitivity (allergic) responses.
Mast cells are basophilic staining cells found chiefly in connective and subcutaneous tissue. They have prominent granules that are the source of many mediators of immediate hypersensitivity and have 30,000–200,000 cell surface membrane receptors for the Fc fragment of IgE. When an allergen molecule cross-links two adjacent mast cell surface–associated IgE antibodies, calcium-dependent cellular activation leads to the release of both preformed and newly generated mediators. Mast cells also have surface receptors for “anaphylatoxins” (activated complement fragments, C3a, C4a, and C5a), cytokines, and neuropeptides, such as substance P. Activation by these non–IgE-mediated mechanisms may contribute to host immunity and provide ties between the immune and neuroendocrine systems. Mast cell–deficient mice display a particular vulnerability to sepsis and rapid death after peritonitis, possibly due to insufficient TNF production during bacterial infection. Mast cells also appear in areas of wound healing and in fibrotic lung disease. Experimentally, mast cell–derived mediators promote angiogenesis and fibrogenesis, suggesting their presence in these sites is pathologically relevant.
Several tissues and organs play roles in host defenses and are functionally classified as the immune system. In mammals, the primary lymphoid organs are the thymus and the bone marrow.
All cells of the immune system are originally derived from bone marrow. Pluripotent stem cells differentiate into lymphocyte, granulocyte, monocyte, erythrocyte, and megakaryocyte populations. In humans, B lymphocytes, which are the antibody-producing cells, undergo early antigen-independent maturation into immunocompetent cells in the bone marrow. Deficiency or dysfunction of the pluripotent stem cell or the various cell lines developing from it can result in immune deficiency disorders of varying expression and severity.
The thymus, derived from the third and fourth embryonic pharyngeal pouches, functions to produce T lymphocytes and is the site of initial T-lymphocyte differentiation. Its reticular structure allows a significant number of lymphocytes to migrate through it to become fully immunocompetent thymus-derived cells. Developing T cells in the thymic cortex are first positively selected for their ability to recognize self-peptides (ie, major histocompatibility complex, MHC). In subsequent negative selection, T cells that avidly recognize self-peptides are destroyed, thus removing deleterious self-reactive clones. In some murine models, autoimmune diseases such as systemic lupus erythematosus may develop in mice with defective apoptotic (programmed cell death) pathways in T cells recognizing self-antigen. The thymus also regulates immune function by secretion of multiple hormones that promote T-lymphocyte differentiation and are essential for T-lymphocyte–mediated immunity.
In mammals, the lymph nodes, spleen, and gut-associated lymphoid tissue are secondary lymphoid organs connected by blood and lymphatic vessels. Lymph nodes are strategically dispersed throughout the vasculature and are the principal organs of the immune system that localize antigen, promote cell-cell interaction and lymphocyte activation, and prevent the spread of infection. Lymph nodes have a framework of reticular cells and fibers that are arranged into a cortex and medulla. B lymphocytes, the precursors of antibody-producing cells, or plasma cells, are found in the cortex (the follicles and germinal centers) as well as in the medulla. T lymphocytes are found chiefly in the medullary and paracortical areas of the lymph node (Figure 3–1). The spleen filters and processes antigens from the blood and is functionally and structurally divided into B-lymphocyte and T-lymphocyte areas, similar to those of the lymph nodes.
Gut-associated lymphoid tissue includes the tonsils, Peyer patches of the small intestine, and the appendix. Like the lymph nodes and spleen, these tissues exhibit separation into B-lymphocyte–dependent and T-lymphocyte–dependent areas. Mucosal immune responses tend to generate antigen-specific IgA, and with some orally administered antigens, T-cell anergy or tolerance may occur rather than immune stimulation.
Mediators are released or generated during immune responses to coordinate and regulate immune cell activities to generate physiological or cytotoxic responses. They target many diverse cell types, can have antiviral, proinflammatory, or anti-inflammatory activities, act locally or systemically, and can be redundant in their actions (Table 3–1). Mediators may exist in a preformed state in the granules of mast cells and basophils or are newly synthesized at the time of activation of these and some other nucleated cells. Increased awareness of the immunologic and physiologic effects of mediators has led to a better understanding of immunopathology and provides potential targets for future pharmacotherapies.
Cytokine | Major Cellular Source | Principal Effect |
---|---|---|
IFN-α | Macrophages, dendritic cells | Inhibit viral replication |
IFN-β | Virally infected cells | |
IFN-γ | T cells, NK cells | Upregulation of adhesion and MHC molecules, increased macrophage and antigen-presenting cell (APC) activity |
IL-1 | Macrophage | Endogenous pyrogen, endothelial cell activation, induces acute-phase reactants |
IL-2 | T cells | T-cell growth factor and regulatory factor, B-cell and NK cell activation |
IL-3 | T cells | Hematopoietic growth factor |
IL-4 | T cells, mast cells | Induces IgE synthesis, TH2 responses |
IL-5 | T cells | Eosinophil activation and growth factor, B-cell activation factor |
IL-6 | Macrophages, T cells, endothelial cells | Induces Ig synthesis and acute-phase reactants |
IL-7 | Bone marrow | B-cell and T-cell growth and differentiation factor |
IL-8 | Macrophage, neutrophils, endothelial, and epithelial cells | Leukocyte chemotactic factor |
IL-10 | T cells, macrophages | Inhibits antigen presentation, cytokine responses |
IL-12 | Macrophage | Induces TH1 responses |
IL-13 | T cells, mast cells | Induces IgE responses |
IL-17 | TH17 | Activation of CD4 T cells |
GM-CSF | Macrophages, T cells | Hematopoietic growth factor for neutrophils, eosinophils, and macrophages |
TGF-β | Platelets | Immune modulator for leukocytes, tissue growth factor for wound healing |
TNF | Macrophage, T cell | Endogenous pyrogen; activates neutrophils, endothelial cells, and acute-phase reactants; promotes angiogenesis and coagulation |
Preformed mediators include histamine, eosinophil and neutrophil chemoattractants, proteoglycans (heparin, chondroitin sulfate), and various proteolytic enzymes. Histamine is a bioactive amine, packaged in dense intracellular granules, that when released binds to membrane-bound H1, H2, and H3 receptors, resulting in significant physiologic effects. Binding to H1 receptors causes smooth muscle contraction, vasodilatation, increased vascular permeability, and stimulation of nasal mucous glands. Stimulation of H2 receptors causes enhanced gastric acid secretion, mucus secretion, and leukocyte chemotaxis. Histamine is important in the pathogenesis of allergic rhinitis, allergic asthma, and anaphylaxis.
Newly generated mediators include kinins, PAF, and arachidonic acid metabolites, including LTs and PGs. In many immune cells, arachidonic acid, liberated from membrane phospholipid bilayers, is metabolized either by the lipoxygenase pathway to form LTs or by the cyclooxygenase pathway to form PGs and thromboxanes A2 and B2 (TXA2 and TXB2). LTB4 is a potent chemoattractant for neutrophils. LTC4, LTD4, and LTE4 constitute slow-reacting substance of anaphylaxis, which has bronchial smooth muscle spasmogenic potency 100–1000 times that of histamine, and which also causes vascular dilation and vascular permeability.
Almost all nucleated cells generate PGs. The most important members are PGD2, PGE2, PGF2, and PGI2 (prostacyclin). Human mast cells produce large amounts of PGD2, which causes vasodilatation, vascular permeability, and airway constriction. Activated polymorphonuclear neutrophils and macrophages generate PGF2α, a bronchoconstrictor, and PGE2, a bronchodilator. PGI2 causes platelet disaggregation. TXA2 causes platelet aggregation, bronchial constriction, and vasoconstriction.
Macrophages, neutrophils, eosinophils, and mast cells generate PAF, which causes platelet aggregation, vasodilatation, increased vascular permeability, and bronchial smooth muscle contraction. PAF is the most potent eosinophil chemoattractant described and also plays a role in anaphylaxis. The kinins are vasoactive peptides formed in plasma when kallikrein, released by basophils and mast cells, digests plasma kininogen. Kinins, including bradykinin, contribute to human angioedema and anaphylaxis by causing slow, sustained contraction of bronchial and vascular smooth muscle, vascular permeability, secretion of mucus, and stimulation of pain fibers.
The union of antigen with IgG or IgM antibody initiates activation of the classic complement pathway. Complement-fixing sites on these immune complexes are exposed, allowing binding of the first component of the complement sequence, C1q. Other components of the complement sequence are subsequently bound, activated, and cleaved, eventually leading to cell lysis. Important byproducts of the classic pathway include activated cleavage products, the anaphylatoxins C3a, C5a, and less-potent C4a. C5a is a potent leukocyte chemotactic factor and causes mediator release from mast cells and basophils. C4b and C3b mediate binding of immune complexes to phagocytic cells, facilitating opsonization.
Activation of the complement sequence by the alternative pathway is initiated by a number of agents, including lipopolysaccharides (LPSs), trypsin-like molecules, aggregated IgA and IgG, and cobra venom. Activation of the alternative pathway does not require the presence of antigen-antibody complexes or use the early components of the complement sequence, C1, C4, and C2. Ultimately, as a result of activation of the classic or alternative pathway, activation of the terminal complement sequence occurs, resulting in cell lysis and/or tissue inflammation. Soluble inhibitors regulate the complement pathway to prevent unchecked activation and prolonged inflammation. Deficiency of one factor, C1-esterase inhibitor, leads to recurrent, potentially life-threatening attacks of facial, laryngeal, and GI swelling in hereditary angioedema.
Many immune functions are regulated or mediated by cytokines, which are soluble factors secreted by activated immune cells. Cytokines can be functionally organized into groups according to their major activities: (1) those that promote inflammation and mediate natural immunity, such as IL-1, IL-6, IL-8, TNF, and IFN-γ; (2) those that support allergic inflammation, such as IL-4, IL-5, and IL-13; (3) those that control lymphocyte regulatory activity, such as IL-10, IL-12, and IFN-γ; and (4) those that act as hematopoietic growth factors, IL-3, IL-7, and GM-CSF (Table 3–1). A group of chemotactic factors (chemokines) regulate homing and migration of immune cells to sites of inflammation. Human immunodeficiency virus (HIV) may exploit certain chemokine receptors to infect host cells, and natural mutations in these same chemokine coreceptors may confer a susceptibility or resistance to infection.
Checkpoint
What are the specific and nonspecific components of the cellular and noncellular limbs of the immune system?
What is the role of macrophages in the immune system, and what are some of the products they secrete?
What are the categories of lymphocytes, and how are they distinguished?
What is the role of lymphocytes in the immune system, and what are some of the products they secrete?
What is the role of eosinophils in the immune system, and what are some of the products they secrete?
What is the role of basophils in the immune system, and what are some of the products they secrete?
What is the role of epithelial cells in the immune system, and what are some of the products they secrete?
What are the primary and secondary lymphoid organs, and what roles do they play in the proper functioning of the immune system?
Living organisms exhibit two levels of response against external invasion: an innate system of natural immunity and an adaptive system that is acquired. Innate immunity is present from birth, does not require previous antigenic exposure, and is nonspecific in its activity. The skin and epithelial surfaces serve as the first line of defense of the innate immune system, whereas enzymes, the alternative complement system pathway, acute-phase proteins, phagocytic, NK cells, and cytokines provide additional layers of protection. Microbial cell walls or nucleic acids contain nonmammalian patterns or motifs that can bind to TLRs on innate immune cells including macrophages and dendritic cells. Their structure is highly conserved and each TLR binds to specific microbial products, such as LPS (or bacterial endotoxin), viral RNA, microbial DNA and yeast wall mannon proteins. Binding of TLR and ligand triggers transcription of proinflammatory factors and cytokine synthesis prior to adaptive responses. Through a series of proteolytic activations, the serum and membrane components of the complement cascade amplify and regulate microbial killing and inflammation. Despite the lack of specificity, innate immunity is largely responsible for protection against a vast array of environmental microorganisms and foreign substances.
Higher organisms have evolved an adaptive immune system, which is triggered by encounters with foreign agents that have evaded or penetrated the innate immune defenses. The adaptive immune system is characterized both by specificity for individual foreign agents and by immunologic memory, which makes possible an intensified response to subsequent encounters with the same or closely related agents. Primary adaptive immune responses require clonal expansion, leading to a delayed response to new exposures. Secondary immune responses are more rapid, larger, and more efficient. Stimulation of the adaptive immune system triggers a complex sequence of events that initiate the activation of lymphocytes, the production of antigen-specific antibodies (humoral immunity) and effector cells (cellular or cell-mediated immunity), and ultimately, the elimination of the inciting substance. Although adaptive immunity is antigen-specific, the repertoire of responses is tremendously diverse, with an estimated 109 antigenic specificities.
Foreign substances that can induce an immune response are called antigens or immunogens. Immunogenicity implies that the substance has the ability to react with antigen-binding sites on antibody molecules or TCRs. Complex foreign agents possess distinct and multiple antigenic determinants or “epitopes,” dependent on the peptide sequence and conformational folding of immunogenic proteins. Most immunogens are proteins, although pure carbohydrates may be immunogenic as well. It is estimated that the human immune system can respond to 107–109 different antigens, an amazingly diverse repertoire.
The primary role of the immune system is to discriminate self from non-self and to eliminate the foreign substance. The physiology of the normal immune response to antigen is summarized in Figure 3–2. A complex network of specialized cells, organs, and biologic factors is necessary for the recognition and subsequent elimination of foreign antigens. These complex cellular interactions require specialized microenvironments in which cells can collaborate efficiently. Both T and B cells need to migrate throughout the body to increase the likelihood that they will encounter an antigen to which they have specificity. Soluble antigens are transported to regional lymph tissues through afferent lymphatic vessels, while other antigens are carried by phagocytic dendritic cells. Regional, peripheral lymphoid organs and the spleen are sites for concentrated immune responses to antigen by recirculating lymphocytes and APCs. Antigens encountered via inhaled or ingested routes activate cells in the mucosa-associated lymphoid tissues. The major pathways of antigen elimination include the direct killing of target cells by cytotoxic T lymphocytes (CTLs; cellular response) and the elimination of antigen through antibody-mediated events arising from T- and B-lymphocyte interactions (humoral response). The series of events that initiate the immune response includes antigen processing and presentation, lymphocyte recognition and activation, cellular or humoral immune responses, and antigenic destruction or elimination.
Figure 3–2
The normal immune response. Cytotoxic T-cell response is shown on the left side of the figure and the helper T-cell response on the right side. As depicted on the left, most CD8 T cells recognize processed antigen presented by MHC class I molecules and destroy infected cells, thereby preventing viral replication. Activated T cells secrete interferon-γ that, along with interferon-α and interferon-β secreted by infected cells, produces cellular resistance to viral infection. On the right and at the bottom, CD4 helper cells (TH1 and TH2 cells) recognize processed antigen presented by MHC class II molecules. TH1 cells secrete interferon-γ and interleukin-2, which activate macrophages and cytotoxic T cells to kill intracellular organisms; TH2 cells secrete interleukin-4, -5, and -6, which help B cells secrete protective antibodies. B cells recognize antigen directly or in the form of immune complexes on follicular dendritic cells in germinal centers.
Most foreign immunogens are not recognized by the immune system in their native form and require capture and processing by professional APCs, which constitutively express class II MHC molecules and accessory costimulatory molecules on their surfaces. Such specialized cells include macrophages, dendritic cells in lymphoid tissue, Langerhans cells in the skin, Kupffer cells in the liver, microglial cells in the nervous system, and B lymphocytes. Dendritic cells in the spleen and lymph nodes may be the primary APCs during a primary immune response. Following an encounter with immunogens, the APCs internalize the foreign substance by phagocytosis or pinocytosis, modify the parent structure, and display antigenic fragments of the native protein on its surfaces in association with MHC class II molecules (see later discussion). T-cell–independent antigens such as polysaccharides can activate B cells without assistance from T cells by binding to B-cell receptors (BCRs, or surface-bound antibody), leading to rapid IgM responses, without generation of memory cells or long-lived plasma cells. Most antigens, however, require internalization and processing by B cells or other APCs with subsequent recognition by CD4 T cells.
The recognition of processed antigen by specialized T lymphocytes known as helper T (CD4) lymphocytes and the subsequent activation of these cells constitute the critical events in the immune response. The helper T lymphocytes orchestrate the many cells and biologic signals (cytokines) that are necessary to carry out the immune response. Activated CD4 T lymphocytes are mainly cytokine-secreting helper cells, whereas CD8 T lymphocytes are mainly cytotoxic killer cells.
Helper T lymphocytes recognize processed antigen displayed by APCs only in association with polymorphic cell surface proteins called the major histocompatibility complex (MHC). MHC genes are highly polymorphic and determine immune responsiveness. They are known as human leukocyte antigen (HLA). The genes encoding MHC distinguish self from non-self, thereby determining immune responsiveness to foreign agents, enabling graft rejection, and conferring susceptibility to certain autoimmune disorders. All somatic cells express MHC class I, whereas only the specialized APCs can express MHC class II. Exogenous foreign antigens are expressed in association with MHC class II structures, expressed only by specialized APCs.
During cell-cell contact between T helper cells and APCs, the process of dual recognition is referred to as MHC restriction. The antigen–MHC class II complex forms the epitope that is recognized by antigen-specific TCRs on the surface of the CD4 molecules. The TCR is composed of six gene products, TCR α- and β-subunits, CD3 (γ-, δ-, and two ε-subunits), and ζ2 chains. Besides binding to modified antigen, activation of T cells depends on the costimulation of accessory molecules. Accessory molecules on T cells bind to ligands found on APCs, epithelial cells, vascular endothelium, and extracellular matrix, controlling the subsequent T-cell function or homing (Table 3–2). In the absence of such signals, the T cell may be “tolerized” or may undergo apoptosis instead of being activated. Biologic products that block some of these costimulatory pathways are currently being investigated as potential therapeutic agents to prevent organ rejection in transplantation and in the management of some autoimmune diseases.
T-Cell Surface Receptor | APC Counter- Receptor | Function and Effect |
---|---|---|
T-cell receptor (CD3) | Processed antigen + MHC complex | Antigen presentation |
CD4 | MHC class II | Presentation of antigen to helper T cell by APC |
CD8 | MHC class I | Presentation of antigen to cytotoxic T cell |
CD40 ligand (CD154) | CD40 | T-cell–induced B-cell activation |
CD28 | B7 | T-cell proliferation and differentiation |
CTLA-4 | B7 | T-cell anergy |
LFA-1 | ICAM-1 | Adhesion |
Before an activated T cell can differentiate, proliferate, produce cytokines, or participate in cell killing, the activation signal must be transduced into the cytoplasm or nucleus of the cell. The principal signaling molecules in the TCR complex appear to be the CD3 and the ζ homodimer or heterodimer. The presence of immunoreceptor tyrosine activation motifs associated with each TCR complex facilitates amplification of signaling. The binding of ZAP-70 (zeta-associated protein 70), a Syk-family protein tyrosine kinase (PTK), to CD3ε and ζ-subunits after they are phosphorylated is critical for downstream signaling. Another important enzyme in the activation of T cells is CD45, a protein tyrosine phosphatase. The critical nature of these enzymes in lymphocyte development is underscored by the discovery of ZAP-70 and CD45 deficiency syndromes, disorders that result in various forms of severe combined immunodeficiency disease (SCID, see Primary Immunodeficiency Diseases).
Activation of T cells does not occur in isolation but is also dependent on the cytokine milieu. In true autocrine fashion, the APCs involved in antigen presentation release IL-1, which induces the release of both IL-2 and IFN-γ from CD4 cells. IL-2 feeds back to stimulate the expression of additional IL-2 receptors on the surface of the CD4 cells and stimulates the production of various cell growth and differentiation factors (cytokines) by the activated CD4 cells. Induction of IL-2 expression is particularly critical for T cells. Cyclosporine and tacrolimus (FK506), two immunosuppressive agents used for prevention of organ transplant rejection, function by downregulating IL-2 production by T cells.
CTLs eliminate target cells (virally infected cells, tumor, or foreign tissues), thus constituting the cellular immune response. CTLs differ from helper T lymphocytes in their expression of the surface antigen CD8 and by the recognition of antigen complexed to cell surface proteins of MHC class I. All somatic cells can express MHC class I molecules. Pathogenic microorganisms, whose proteins gain access to the cell cytoplasm (eg, malarial parasites) or by de novo gene expression in the infected cell cytoplasm (eg, viruses) stimulate CD8 class I MHC-restricted T-cell responses. Killing of target cells by CTLs requires direct cell-to-cell contact. Two major mechanisms for killing target cells have been described: (1) CTL secretion of a pore-forming protein (perforin) that inserts in the plasma membrane of target cells along with serine proteases called granzymes, leading to osmotic lysis; and (2) expression of the Fas ligand on the surface of CTLs that bind to Fas on the target cell membrane inducing programmed cell death (apoptosis). In addition to killing infected cells directly, CD8 T cells can elaborate a number of cytokines, including TNF and lymphotoxin. Memory CTLs may be long-lived to provide “recall” responses and immunity against latent or persistent viral infections.
The primary function of mature B lymphocytes is to synthesize antibodies. Like T-cell activation, B-lymphocyte activation is triggered after antigen binds to BCRs (ie, surface-bound immunoglobulin) and is regulated through concomitant coreceptor binding. In secondary lymphoid tissues, release of cytokines IL-2, IL-4, IL-5, and IL-6 by activated helper T lymphocytes promotes the proliferation and terminal differentiation of B cells into high-rate antibody-producing cells called plasma cells, which secrete antigen-specific immunoglobulin. If complement fragments bind B-cell surface complement receptors at the same time antigen engages BCRs, cellular responses are heightened. T cells also modulate humoral immunity through their activation-dependent membrane expression of CD40 ligand protein. Through direct T- and B-cell contact, CD40 ligand binds to the CD40 receptor on the surface of B cells, inducing apoptosis (programmed cell death) or activation of immunoglobulin synthesis, depending on the situation. The importance of CD40 ligand-CD40 binding in normal humoral immunity is highlighted by the congenital immunodeficiency, X-linked hyper-IgM syndrome. A defect in the synthesis of CD40 ligand on activated T cells results in impaired “isotype switching” and hyper-IgM, with subsequent deficient production of IgG, IgA, and impaired humoral immunity.
Although their primary function is synthesis of immunoglobulin, B lymphocytes may also bind and internalize foreign antigen directly, process that antigen, and present it to CD4 T lymphocytes. A pool of activated B lymphocytes may differentiate into memory cells, which respond more rapidly and efficiently to subsequent encounters with identical or closely related antigenic structures.
Antibodies (immunoglobulins) are proteins that possess “specificity,” enabling them to combine with one particular antigenic structure. Antigen-binding sites for immunoglobulin will recognize three-dimensional structures, whereas TCR will bind short peptide segments without tertiary structure. Humoral (antibody-mediated) immune responses result in the production of a diverse repertoire (estimated 109–1011) of antibody specificities, providing the ability to recognize and bind with a broad range of antigens. This diversity is a function of somatic recombination of gene segments within B lymphocytes early in ontogenetic development. Somatic mutations occurring after antigenic stimulation lead to affinity maturation, ie, the average affinity of antibody binding increases throughout the immune response. Somatic recombination, in both T cells and B cells, is dependent on recombination-activating genes (RAG1 and RAG2), the deficiency of which leads to a lack of T and B lymphocytes, an autosomal recessive form of SCID.
All immunoglobulin molecules share a four-chain polypeptide structure consisting of two heavy and two light chains (Figure 3–3). Each chain includes an amino terminal portion, containing the variable (V) region, and a carboxyl terminal portion, containing four or five constant (C) regions. V regions are highly variable structures that form the antigen-binding site, whereas the C domains support effector functions of the molecules. The five classes (isotypes) of immunoglobulins are IgG, IgA, IgM, IgD, and IgE and are defined on the basis of differences in the C region of the heavy chains. The isotype expressed by a particular B lymphocyte is dependent on the state of cellular differentiation and “isotype switching,” a process characterized by splicing of heavy chain mRNA prior to translation. Different isotypes contribute to different effector functions on the basis of the ability of the molecule to bind to specific receptors and their efficiency in fixing serum complement. IgG is the predominant immunoglobulin in serum with the longest half-life. Four subclasses—IgG1, IgG2, IgG3, and IgG4—differ in their relative quantities and targets (protein vs. carbohydrate antigens). IgA is the predominant immunoglobulin on mucous membrane surfaces. It exists predominantly as a monomer in serum and as a dimer or trimer when secreted on mucous membrane surfaces. IgA antibodies protect the host from foreign antigens on mucous membrane surfaces, but they do not fix complement by the classic pathway. IgM is a pentamer that is found almost exclusively in the intravascular compartment. IgM is expressed early in immune responses, providing rapid adaptive immunity and detection of antigen-specific IgM can be used diagnostically during certain infections. IgD is a monomeric immunoglobulin. Its biological function is unknown. IgE is the heaviest immunoglobulin monomer, with a normal concentration in serum varying from 20 to 100 IU, but the concentration may be 5 times normal or even higher in an atopic individual. The Fc portion of IgE binds to receptors on the surfaces of mast cells and basophils. IgE antibodies play an important role in immediate hypersensitivity reactions.
Figure 3–3
Structure of a human IgG antibody molecule. Depicted are the four-chain structure and the variable and constant domains. (V, variable region; C, constant region. The sites of pepsin and papain cleavage are shown.) (Redrawn, with permission, from Stites DP et al, eds. Basic & Clinical Immunology, 9th ed. Originally published by Appleton & Lange. Copyright © 1997 by The McGraw-Hill Companies, Inc.)
Antibodies induce the elimination of foreign antigen through a number of different mechanisms. Binding of antibody to bacterial toxins or foreign venoms may cause neutralization or promote elimination of these antigen-antibody immune complexes through the reticuloendothelial system. Antibodies may coat bacterial surfaces, enhancing phagocytosis by macrophages in a process known as opsonization. Some classes of antibodies may complex with antigen and activate the complement cascade (“complement fixation”), culminating in lysis of the target cell. Finally, the major class of antibody, IgG, can bind to NK cells that subsequently complex with target cells and release cytotoxins (see prior discussion of antibody-dependent cellular cytotoxicity). IgG passes transplacentally, providing passive immunization of neonates.
After the successful elimination of antigen, the immune system uses several mechanisms to return to basal homeostasis. IgG can switch off its own response to antigen through the binding of immune complexes that transmit inhibitory signals into the nuclei of B cells.
Elimination of foreign antigen by cellular or humoral processes is integrally linked to the inflammatory response, in which cytokines and antibodies trigger the recruitment of additional cells and the release of endogenous vasoactive and proinflammatory enzymatic substances (inflammatory mediators).
Inflammation may have both positive and deleterious effects. Tight control of inflammatory mechanisms promotes efficient elimination of foreign substances, killing of microbes, infected cells, and tumors. Uncontrolled lymphocyte activation and unregulated antibody production, however, can lead to tissue damage and organ dysfunction. Pathogenic immune dysfunction is responsible for hypersensitivity reactions, immunodeficiency, and many of the clinical effects of autoimmunity. Imbalances in the inflammatory system may result from genetic defects, infection, neoplasms, and exposure to environmental triggers, although precise mechanisms that promote abnormal regulation and persistence of inflammatory processes are complex and poorly understood.
Gell and Coombs classified the mechanisms of immune responses to antigen into four distinct types of reactions to allow for clearer understanding of the immunopathogenesis of disease.
Clinical allergy represents IgE-mediated hypersensitivity response arising from deleterious inflammation in response to the presence of normally harmless environmental antigens. Anaphylactic or immediate hypersensitivity reactions occur after binding of antigen to IgE antibodies attached to the surface of the mast cell or basophil and result in the release of preformed and newly generated inflammatory mediators that produce the clinical manifestations. Examples of type I–mediated reactions include anaphylactic shock, allergic rhinitis, allergic asthma, and allergic drug reactions.
Cytotoxic reactions involve the binding of either IgG or IgM antibody to antigens covalently bound to cell membrane structures. Antigen-antibody binding activates the complement cascade and results in destruction of the cell to which the antigen is bound. Examples of tissue injury by this mechanism include immune hemolytic anemia and Rh hemolytic disease in the newborn. Another example of the type II–mediated disease process without cell death is autoimmune hyperthyroidism, a disorder in which anti-thyroid antibodies stimulate thyroid tissue.
Antigen binding to antibodies with fixation of complement forms immune complex–mediated reactions. Complement-bound immune complexes facilitate opsonization by phagocytes and ADCC. Complexes are usually cleared from the circulation in the reticuloendothelial system. However, deposition of these complexes in tissues or in vascular endothelium can produce immune complex–mediated tissue injury through complement activation, anaphylatoxin generation, chemotaxis of polymorphonuclear leukocytes, mediator release and tissue injury. Cutaneous Arthus reaction, systemic serum sickness, some aspects of clinical autoimmunity, and certain features of infective endocarditis are clinical examples of type III–mediated diseases.
Cell-mediated immunity is responsible for host defenses against intracellular pathogenic organisms, although abnormal regulation of this system may result in delayed-type hypersensitivity. Type IV hypersensitivity reactions are mediated not by antibody but by antigen-specific T lymphocytes. Classic examples are tuberculin skin test reactions and contact dermatitis.
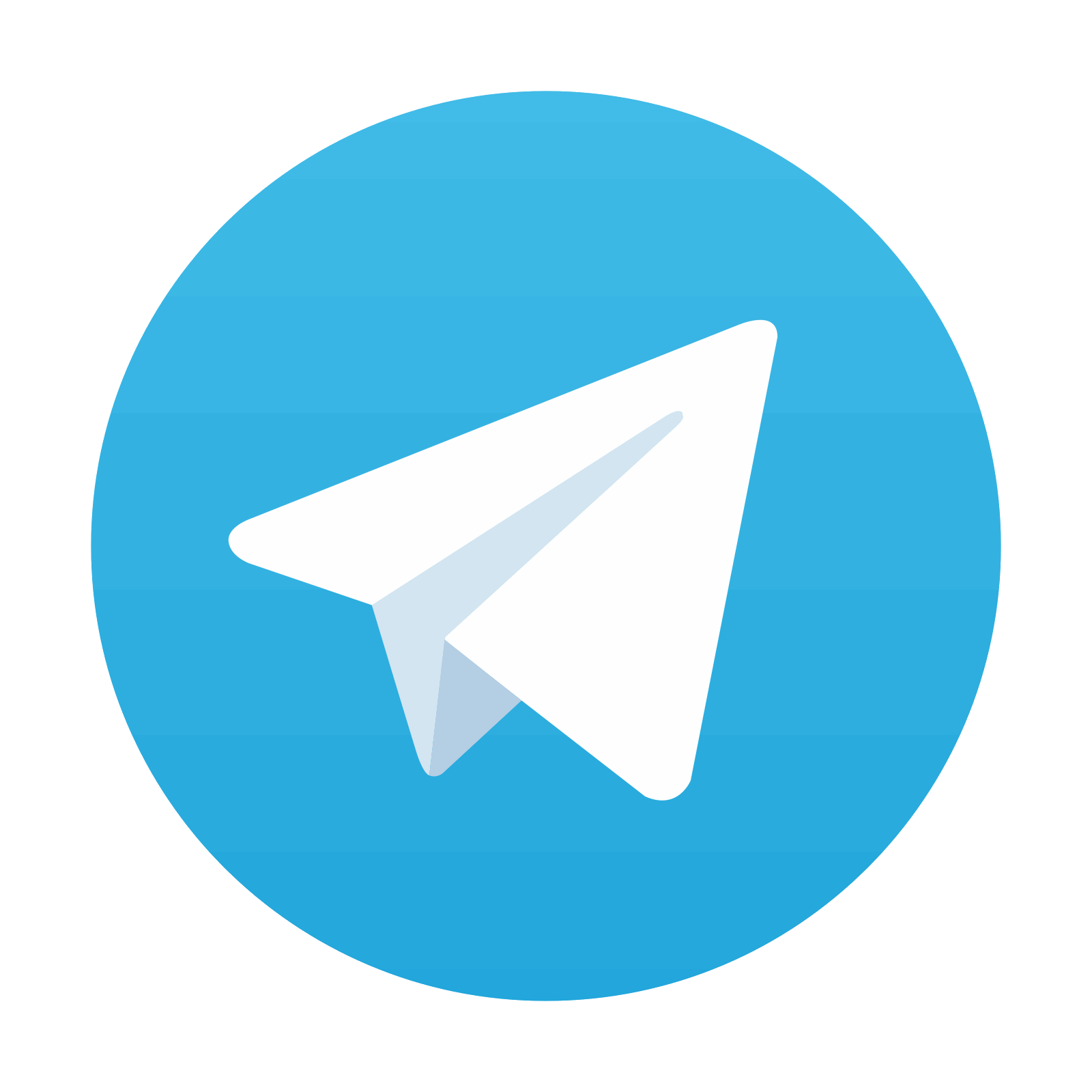
Stay updated, free articles. Join our Telegram channel

Full access? Get Clinical Tree
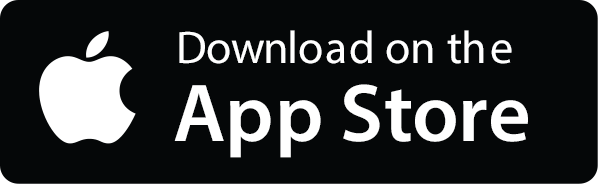
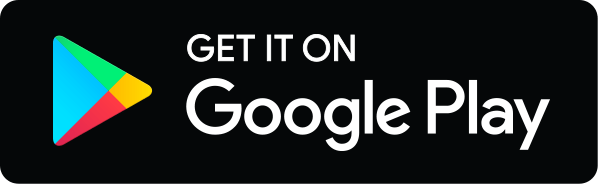