CHAPTER 24 Control of Respiration
We breathe without thinking, and we can willingly modify our breathing pattern and even hold our breath. Control of ventilation, which is discussed in this chapter, includes the generation and regulation of rhythmic breathing by the respiratory center in the brainstem and its modification by the input of information from higher brain centers and from systemic receptors. The goal of breathing, from a mechanical perspective, is to minimize work and, from a physiological perspective, to maintain blood gases and, specifically, to regulate arterial PCO2. Another goal of breathing is to maintain acid-base balance in the brain by regulating arterial PCO2. Automatic respiration begins at birth. In utero, the placenta, not the lung, is the organ of gas exchange in the fetus. Its microvilli interdigitate with the maternal uterine circulation, and O2 transport and CO2 removal from the fetus occur by passive diffusion across the maternal circulation.
RESPONSE TO CO2
Ventilation is regulated by PCO2, PO2, and pH in arterial blood. Arterial PCO2 is the most important of these regulators. Both the rate and depth of breathing are controlled to maintain PaCO2 close to 40 mm Hg. In a normal awake individual, there is a linear rise in ventilation as arterial PCO2 reaches and exceeds 40 mm Hg (Fig. 24-1). Changes in PaCO2 are sensed by central and peripheral chemoreceptors, and they transmit this information to the medullary respiratory centers. The respiratory control center then regulates minute ventilation and thereby maintains arterial PCO2 within the normal range. In the presence of a normal PAO2, ventilation increases by about 3 L/min for each millimeter rise in PaCO2. The response to an increase in PACO2 is further increased in the presence of a low PaO2 (Fig. 24-2). With a low Pao2, ventilation is greater for any given PACO2, and the increase in ventilation for a given increment in PACO2 is enhanced (the slope is greater).
The slope of the minute ventilation response as a function of the inspired CO2 is termed the ventilatory response to CO2 and is a test of CO2 sensitivity. It is important to recognize that this relationship is amplified by low O2 (Fig. 24-2, B). The enhanced responsiveness to low O2 occurs because different mechanisms are responsible for sensing PO2 and PCO2 in the peripheral chemoreceptors. Thus, the presence of both hypercapnia and hypoxemia (often called asphyxia when both changes are present) has an additive effect on chemoreceptor output and on the resulting ventilatory stimulation.
The ventilatory drive or response to changes in PCO2 can be reduced by hyperventilation and by drugs, such as morphine, barbiturates, and anesthetic agents, that depress the respiratory center and decrease the ventilatory response to both CO2 and O2 (Fig. 24-1). In these instances, the stimulus is inadequate to stimulate the motor neurons that innervate the muscles of respiration. It is also depressed during sleep.
In addition, the ventilatory response to changes in PCO2 is reduced if the work of breathing is increased, which can occur in individuals with chronic obstructive pulmonary disease (COPD) (Fig. 24-1). This effect occurs primarily because the neural output of the respiratory center is less effective in promoting ventilation as a result of the mechanical limitation to ventilation.
CONTROL OF VENTILATION: THE DETAILS
The Respiratory Control Center
When the brain is transected experimentally between the medulla and the pons, periodic breathing is maintained, thus demonstrating that the inherent rhythmicity of breathing originates in the medulla. Although no single group of neurons in the medulla has been found to be the breathing “pacemaker,” two distinct nuclei within the medulla are involved in generation of the respiratory pattern (Fig. 24-3). One nucleus is the dorsal respiratory group (DRG), which is composed of cells in the nucleus tractus solitarius located in the dorsomedial region of the medulla. Cells in the DRG receive afferent input from the 9th and 10th cranial nerves, which originate from airways and the lung and are thought to constitute the initial intracranial processing station for this afferent input. The second group of medullary cells is the ventral respiratory group (VRG), located in the ventrolateral region of the medulla. The VRG is composed of three cell groups: the rostral nucleus retrofacialis, the caudal nucleus retroambiguus, and the nucleus paraambiguus. The VRG contains both inspiratory and expiratory neurons. The nucleus retrofacialis and the caudally located cells of the nucleus retroambiguus are active during exhalation, whereas the rostrally located cells of the nucleus retroambiguus are active during inspiration. The nucleus paraambiguus has inspiratory and expiratory neurons that travel in the vagus nerve to the laryngeal and pharyngeal muscles. Discharges from cells in these areas excite some cells and inhibit other cells.
At the level of the respiratory control center, inspiration and exhalation involve three phases—one inspiratory and two expiratory (Fig. 24-4). Inspiration begins with an abrupt increase in discharge from cells in the nucleus tractus solitarius, the nucleus retroambiguus, and the nucleus paraambiguus, followed by a steady ramplike increase in firing rate throughout inspiration. This leads to progressive contraction of the respiratory muscles during automatic breathing. At the end of inspiration, an off-switch event results in a marked decrease in neuron firing, at which point exhalation begins. At the start of exhalation (phase I of expiration), a paradoxical increase in inspiratory neuron firing slows the expiratory phase down by increasing inspiratory muscle tone and expiratory neuron firing. This inspiratory neuron firing decreases and stops during phase II of exhalation. Although many different neurons in the DRG and VRG are involved in ventilation, each cell type appears to have a specific function. For example, the Hering-Breuer reflex is an inspiratory inhibitory reflex that arises from afferent stretch receptors located in the smooth muscles of the airways. Increasing lung inflation stimulates these stretch receptors and results in early exhalation by stimulating the neurons associated with the off-switch phase of inspiratory muscle control. Thus, rhythmic breathing depends on a continuous (tonic) inspiratory drive from the DRG and an intermittent (phasic) expiratory drive from the cerebrum, thalamus, cranial nerves, and ascending sensory tracts in the spinal cord.
< div class='tao-gold-member'>
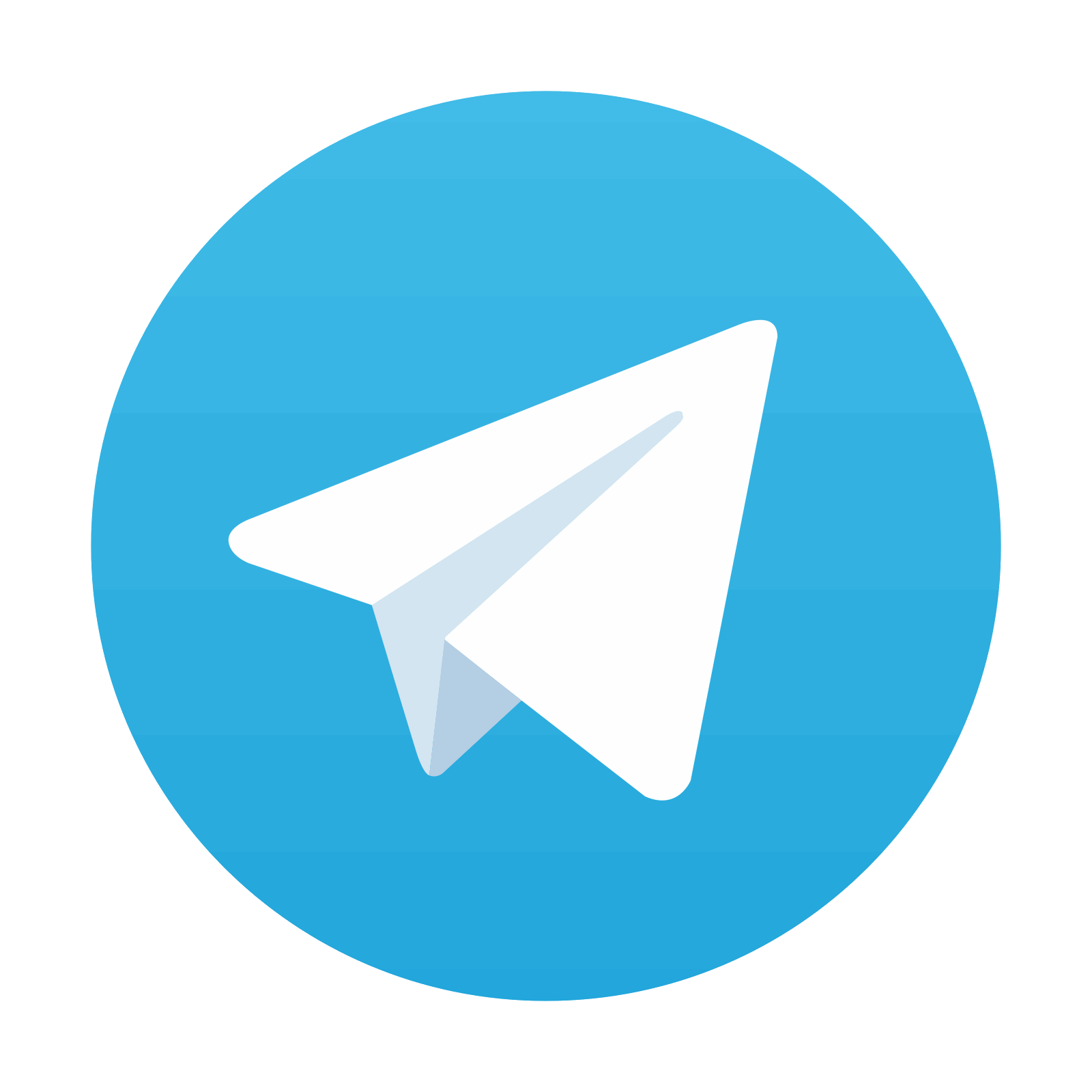
Stay updated, free articles. Join our Telegram channel

Full access? Get Clinical Tree
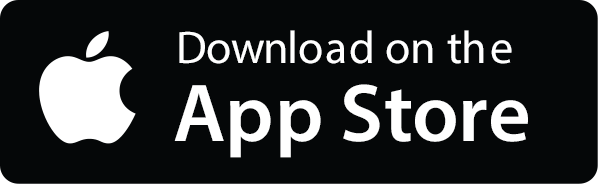
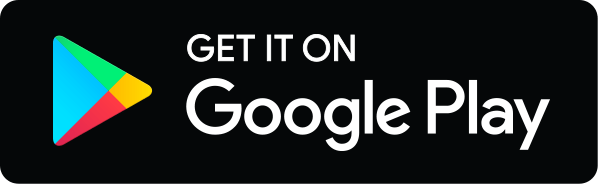