Objectives
- Describes the general organization of the respiratory control system.
- Localizes the centers that generate the spontaneous rhythmicity of breathing.
- Describes the groups of neurons that effect inspiration and expiration.
- Describes the other centers in the brainstem that may influence the spontaneous rhythmicity of breathing.
- Lists the cardiopulmonary and other reflexes that influence the breathing pattern.
- States the ability of the brain cortex to override the normal pattern of inspiration and expiration temporarily.
- Describes the effects of alterations in body oxygen, carbon dioxide, and hydrogen ion levels on the control of breathing.
- Describes the sensors of the respiratory system for oxygen, carbon dioxide, and hydrogen ion concentration.
Control of Breathing: Introduction
Breathing is spontaneously initiated in the central nervous system. A cycle of inspiration and expiration is automatically generated by neurons located in the brainstem; in eupneic states, breathing occurs without a conscious initiation of inspiration and expiration. Normal individuals do not have to worry about forgetting to breathe while they sleep.
This spontaneously generated cycle of inspiration and expiration can be modified, altered, or even temporarily suppressed by a number of mechanisms. As shown in Figure 9–1, these include reflexes arising in the lungs, the airways, and the cardiovascular system; information from receptors in contact with the cerebrospinal fluid; and commands from higher centers of the brain such as the hypothalamus, the centers of speech, or other areas in the cortex. The centers that are responsible for the generation of the spontaneous rhythmicity of inspiration and expiration are, therefore, able to alter their activity to meet the increased metabolic demand on the respiratory system during exercise or may even be temporarily superseded or suppressed during speech or breath holding.
Figure 9–1.
Schematic representation of the organization of the respiratory control system. A cycle of inspiration and expiration is automatically established in the medullary respiratory center. Its output represents a final common pathway to the respiratory muscles, except for some voluntary pathways that may go directly from higher centers to the respiratory muscles (dashed line). Reflex responses from chemoreceptors and other sensors may modify the cycle of inspiration and expiration established by the medullary respiratory center.
The output of the respiratory control centers in the brainstem controls breathing via a “final common pathway” consisting of the spinal cord, the innervation of the muscles of respiration such as the phrenic nerves, and the muscles of respiration themselves. Alveolar ventilation is therefore determined by the interval between successive groups of discharges of the respiratory neurons and the innervation of the muscles of respiration, which determines the respiratory rate or breathing frequency, and by the frequency of neural discharges transmitted by individual nerve fibers to their motor units, the duration of these discharges, and the number of motor units activated during each inspiration or expiration, which determine the depth of respiration or the tidal volume. Note that some pathways from the cerebral cortex to the muscles of respiration, such as those involved in voluntary breathing, bypass the medullary respiratory center described later in this chapter and travel directly to the spinal α motorneurons. These are represented by the dashed line in Figure 9–1.
The Generation of Spontaneous Rhythmicity
The centers that initiate breathing are located in the reticular formation of the medulla, beneath the floor of the fourth ventricle. If the brainstem of an anesthetized animal is sectioned above this area, as seen in the transection labeled III in Figure 9–2, a pattern of inspiration and expiration is maintained (although it is somewhat irregular) even if all other afferents to this area, including the vagi, are also severed. If the brainstem is transected below this area, as seen in the transection labeled IV in Figure 9–2, breathing ceases. This area, known as the medullary center (or medullary respiratory center), was originally believed to consist of 2 discrete groups of respiratory neurons: the inspiratory neurons, which fire during inspiration and the expiratory neurons, which fire during expiration.
Figure 9–2.
The effects of transections at different levels of the brainstem on the ventilatory pattern of anesthetized animals. Left: A schematic representation of the dorsal surface of the lower brainstem. Right: A schematic representation of the breathing patterns (inspiration is upward) corresponding to the transections with the vagus nerves intact or transected. PRG = pontine respiratory groups; DRG = dorsal respiratory group; VRG = ventral respiratory group. (From Physiology of Respiration by Michael P. Hlastala and Albert J. Berger, copyright © 1996 by Oxford University Press, Inc. Used by permission of Oxford University Press, Inc.)
Activity of the inspiratory neurons is transmitted to the muscles of inspiration, initiating inspiration; activity of the expiratory neurons is transmitted to the muscles of expiration, initiating expiration. It was thought that when the inspiratory neurons discharged, their activity was conducted to the expiratory neuron pool via collateral fibers and the activity of the expiratory neurons was inhibited. Similarly, when the expiratory neurons discharged, their activity was conducted to the inspiratory neuron pool via collateral fibers, and the activity of the inspiratory neurons was inhibited. The reciprocal inhibition of these 2 opposing groups of neurons was believed to be the source of the spontaneous respiratory rhythmicity. More recent studies of the medullary center have not entirely supported this early hypothesis. Furthermore, because expiration is passive in normal quiet breathing, the expiratory neurons may not discharge unless expiration is active.
The Medullary Respiratory Center
There are 2 dense bilateral aggregations of respiratory neurons in the medullary respiratory center known as the dorsal respiratory groups (DRG in Figures 9–2 and 9–3) and the ventral respiratory groups (VRG in Figures 9–2 and 9–3). Inspiratory and expiratory neurons are anatomically intermingled to a greater or lesser extent within these areas, and the medullary center does not consist of a discrete “inspiratory center” and a discrete “expiratory center.”
The DRG are located bilaterally in the nucleus of the tractus solitarius (NTS), as shown in Figure 9–3. They consist mainly of inspiratory neurons. These inspiratory neurons project primarily to the contralateral spinal cord. They are the principal initiators of the activity of the phrenic nerves and are therefore responsible for maintaining diaphragmatic activity. Dorsal respiratory group neurons send many collateral fibers to those in the ventral respiratory group, but the ventral respiratory group sends only a few collateral fibers to the dorsal respiratory group, as will be discussed in the next section. Reciprocal inhibition therefore seems an unlikely explanation of spontaneous inspiratory and expiratory rhythmicity.
The NTS is the primary projection site of visceral afferent fibers of the ninth cranial nerve (the glossopharyngeal) and the tenth cranial nerve (the vagus). These nerves carry information about the arterial ,
, and pH from the carotid and aortic arterial chemoreceptors and information concerning the systemic arterial blood pressure from the carotid and aortic baroreceptors. In addition, the vagus carries information from stretch receptors and other sensors in the lungs that may also strongly influence the control of breathing. The effects of information from these sensors on the control of breathing will be discussed in detail later in this chapter. The location of the DRG within the NTS suggests that it may be the site of integration of various inputs that can reflexly alter the spontaneous pattern of inspiration and expiration.
There are 2 populations of inspiratory neurons in the DRG: One population, called the I α cells, increase their activity if lung inflation is suppressed; the second population, the I β cells, decrease their activity if lung inflation is suppressed. These cells may play an important role in the Hering-Breuer reflexes described later in this chapter. A third population of cells, the P-cells (pump cells), appear to be interneurons involved in relaying afferent activity from pulmonary stretch receptors.
In summary, the DRG is probably responsible for driving the diaphragm and is probably the initial integrating site for many cardiopulmonary reflexes that affect the respiratory rhythm.
The VRG are located bilaterally in the retrofacial nucleus, the nucleus ambiguus, the nucleus para-ambigualis, and the nucleus retroambigualis, as shown in Figure 9–3. They consist of both inspiratory and expiratory neurons. The neurons in the nucleus ambiguus are primarily vagal motorneurons that innervate the ipsilateral laryngeal, pharyngeal, and tongue muscles involved in breathing and in maintaining the patency of the upper airway. Neurons in the nucleus para-ambigualis mainly innervate contralateral inspiratory muscles, including the external intercostals. In the nucleus retroambigualis, the inspiratory cells appear to be located more rostrally and the expiratory cells are located more caudally. There appear to be 2 populations of inspiratory cells in the nucleus retroambigualis: One group mainly projects contralaterally to external intercostal muscles, with some fibers also sent to the phrenic nerves, thus innervating the diaphragm; the second group appears to project only within the medulla to other inspiratory and expiratory cells. The expiratory neurons in the nucleus retroambigualis project to the contralateral spinal cord to drive the internal intercostal and abdominal muscles. The retrofacial nucleus, located most rostrally in the VRG, mainly contains expiratory neurons in a group of cells called the Bötzinger complex. This group of neurons has been shown to inhibit inspiratory cells in the DRG, as well as some phrenic motorneurons.
In summary, the VRG neurons consist of both inspiratory and expiratory cells. Their major function is to drive either spinal respiratory neurons, innervating mainly the intercostal and abdominal muscles, or the auxiliary muscles of respiration innervated by the vagus nerves. Many expiratory cells may not fire at all during the passive expirations seen in eupneic breathing (see Chapter 2); those that do discharge do not cause contraction of the expiratory muscles.
The mechanism and precise location of the generation of the inspiratory-expiratory cycle, the respiratory rhythm generator, is not firmly established. It may be generated by a network of neurons in the ventral medulla or cells in the pre-Bötzinger complex (Figure 9–3) may act as pacemakers of the respiratory rhythm.
If the brainstem is transected in the pons at the level denoted by the line labeled II in Figure 9–2, a breathing pattern called apneusis results if the vagus nerves have also been transected. Apneustic breathing consists of prolonged inspiratory efforts interrupted by occasional expirations. Afferent information that reaches this so-called apneustic center via the vagus nerves must be important in preventing apneusis because apneusis does not occur if the vagus nerves are intact, as shown in Figure 9–2.
Apneusis is probably caused by a sustained discharge of medullary inspiratory neurons. Therefore, the apneustic center may be the site of the normal “inspiratory cutoff switch”; that is, it is the site of projection and integration of various types of afferent information that can terminate inspiration. Apneusis is a result of the inactivation of the inspiratory cutoff mechanism. The specific group of neurons that function as the apneustic center has not been identified, but it must be located somewhere between the lines labeled II and III in Figure 9–2.
The Pontine Respiratory Groups
If the brainstem is transected immediately caudal to the inferior colliculus, as denoted by the line labeled I in Figure 9–2, the breathing pattern shows an essentially normal balance between inspiration and expiration, even if the vagus nerves are transected. As discussed in the previous section, transections made caudal to the line labeled II in Figure 9–2 lead to apneusis in the absence of the vagus nerves. A group of respiratory neurons known as the pontine respiratory groups, (formerly called the pneumotaxic center) therefore function to modulate the activity of the apneustic center. These cells, located in the upper pons in the nucleus parabrachialis medialis and the Kölliker-Fuse nucleus (shown in Figure 9–3), probably function to “fine-tune” the breathing pattern. Electrical stimulation of these structures can result in synchronization of phrenic nerve activity with the stimulus or premature switching from inspiration to expiration and vice versa. Pulmonary inflation afferent information can inhibit the activity of the pontine respiratory groups, which may in turn act to modulate the threshold for lung inflation inspiratory cutoff. The pontine respiratory groups may also modulate the respiratory control system’s response to other stimuli, such as hypercapnia and hypoxia.
Spinal Pathways
Axons projecting from the DRG, the VRG, the cortex, and other supraspinal sites descend in the spinal white matter to activate the diaphragm and the intercostal and abdominal muscles of respiration, as already discussed. There is also integration of descending influences and local spinal reflexes that can affect these respiratory motor neurons. Descending axons with inspiratory activity excite phrenic and external intercostal motorneurons and also inhibit internal intercostal motorneurons by exciting spinal inhibitory interneurons. They are actively inhibited during expiratory phases of the respiratory cycle.
Reflex Mechanisms of Respiratory Control
A large number of sensors located in the lungs, the cardiovascular system, the muscles and tendons, and the skin and viscera can elicit reflex alterations in the control of breathing. These are summarized in Table 9–1.
Stimulus | Reflex Name | Receptor | Afferent Pathway | Effects |
---|---|---|---|---|
Lung inflation | Hering-Breuer inflation reflex | Stretch receptors within smooth muscle of large and small airways | Vagus | Respiratory
|
Cardiovascular
| ||||
Lung deflation | Hering-Breuer deflation reflex | Possibly J receptors, irritant receptors in lungs, or stretch receptors in airways | Vagus | Respiratory
|
Lung inflation | Paradoxical reflex of Head | Stretch receptors in lungs | Vagus | Respiratory
|
Negative pressure in the upper airway | Pharyngeal dilator reflex | Receptors in nose, mouth, upper airways | Trigeminal, laryngeal, glossopharyngeal | Respiratory
|
Mechanical or chemical irritation of airways | Cough | Receptors in upper airways, tracheobronchial tree | Vagus | Respiratory
|
Sneeze | Receptors in nasal mucosa | Trigeminal, olfactory | Sneeze; bronchoconstriction | |
Cardiovascular
| ||||
Face immersion* | Diving reflex | Receptors in nasal mucosa and face | Trigeminal | Respiratory
|
Cardiovascular
| ||||
Pulmonary embolism | J receptors in pulmonary vessels | Vagus | Respiratory
| |
Pulmonary vascular congestion | J receptors in pulmonary vessels | Vagus | Respiratory
| |
Specific chemicals in the pulmonary circulation | Pulmonary chemoreflex | J receptors in pulmonary vessels | Vagus | Respiratory
|
Low Pao2, high Paco2, low pHa | Arterial chemoreceptor reflex | Carotid bodies, aortic bodies | Glossopharyngeal, vagus | Respiratory
|
Cardiovascular
| ||||
Increased systemic arterial blood pressure | Arterial baroreceptor reflex | Carotid sinus stretch receptors, aortic arch stretch receptors | Glossopharyngeal, vagus | Respiratory
|
Cardiovascular
| ||||
Stretch of muscles, tendons, movement of joints | Muscle spindles, tendon organs, proprioreceptors | Various spinal pathways | Respiratory
| |
Somatic pain | Pain receptors | Various spinal pathways | Respiratory
| |
Cardiovascular
|
Three respiratory reflexes can be elicited by stimulation of the pulmonary stretch receptors: the Hering-Breuer inflation reflex, the Hering-Breuer deflation reflex, and the “paradoxical” reflex of Head.
In 1868, Breuer and Hering reported that a maintained distention of the lungs of anesthetized animals decreased the frequency of the inspiratory effort or caused a transient apnea. The stimulus for this reflex is pulmonary inflation. The sensors are stretch receptors located within the smooth muscle of large and small airways. They are sometimes referred to as slowly adapting pulmonary stretch receptors because their activity is maintained with sustained stretches. The afferent pathway consists of large myelinated fibers in the vagus; as mentioned previously, these fibers appear to enter the brainstem and project to the DRGs, the apneustic center, and the pontine respiratory groups. The efferent limb of the reflex consists of bronchodilation in addition to the apnea or slowing of the ventilatory frequency (due to an increase in the time spent in expiration) already mentioned. Lung inflation also causes reflex effects in the cardiovascular system: Moderate lung inflations cause an increase in heart rate and may cause a slight vasoconstriction; very large inflations may cause a decrease in heart rate and systemic vascular resistance.
The Hering-Breuer inflation reflex was originally believed to be an important determinant of the rate and depth of ventilation. Vagotomized anesthetized animals breathe much more deeply and less frequently than they did before their vagus nerves were transected. It was therefore assumed that the Hering-Breuer inflation reflex acts tonically to limit the tidal volume and establish the depth and rate of breathing. More recent studies on unanesthetized humans have cast doubt on this conclusion because the central threshold of the reflex is much higher than the normal tidal volume during eupneic breathing. Tidal volumes of 800 to 1500 mL are generally required to elicit this reflex in conscious eupneic adults. The Hering-Breuer inflation reflex may help minimize the work of breathing by inhibiting large tidal volumes (see Chapter 2) as well as to prevent overdistention of the alveoli at large volumes. It may also be important in the control of breathing in babies. Infants have Hering-Breuer inflation reflex thresholds within their normal tidal volume ranges, and the reflex may be an important influence on their tidal volumes and respiratory rates.
Breuer and Hering also noted that abrupt deflation of the lungs increases the ventilatory rate. This could be a result of decreased stretch receptor activity or of stimulation of other pulmonary receptors, or rapidly adapting receptors such as the irritant receptors and J receptors, which will be discussed later in this chapter. The afferent pathway is the vagus, and the effect is hyperpnea. This reflex may be responsible for the increased ventilation elicited when the lungs are deflated abnormally, as in pneumothorax, or it may play a role in the periodic spontaneous deep breaths (“sighs”) that help prevent atelectasis. These sighs occur occasionally (approximately 6–8/hour) and irregularly during the course of normal, quiet, spontaneous breathing. They consist of a slow deep inspiration (larger than a normal tidal volume) followed by a slow expiration. This response appears to be very important because patients maintained on mechanical ventilators must be given large tidal volumes or periodic deep breaths or they develop diffuse atelectasis, which may lead to arterial hypoxemia. Yawns may also help prevent atelectasis, although there is no consensus on how they are initiated.
The Hering-Breuer deflation reflex may be very important in helping to actively maintain infants’ functional residual capacities (FRCs). It is very unlikely that infants’ FRCs are determined passively like those of adults because the inward recoil of their lungs is considerably greater than the outward recoil of their very compliant chest walls.
In 1889, Henry Head performed experiments designed to show the effects of the Hering-Breuer inflation reflex on the control of breathing. Instead of transecting the vagus nerves, he decided to block their function by cooling them to 0°C. As he rewarmed the vagus nerves, he noted that in the situation of a selective partial block of the vagus nerves, lung inflation caused a further inspiration instead of the apnea expected when the vagus nerves were completely functional. The receptors for this paradoxical reflex are located in the lungs, but their precise location is not known. Afferent information travels in the vagus; the effect is very deep inspirations. This reflex may also be involved in the sigh response, or it may be involved in generating the first breath of the newborn baby; very great inspiratory efforts must be generated to inflate the fluid-filled lungs.
As discussed in Chapter 2, negative pressure in the upper airway causes reflex contraction of the pharyngeal dilator muscles. The receptors appear to be located in the nose, mouth, and upper airways; the afferent pathways appear to be in the trigeminal, laryngeal, and glossopharyngeal nerves. This reflex may be very important in protecting the upper airways from collapsing, especially during sleep (See Chapter 11). Inhibition or interference with this reflex may be one of the causes of obstructive sleep apnea.
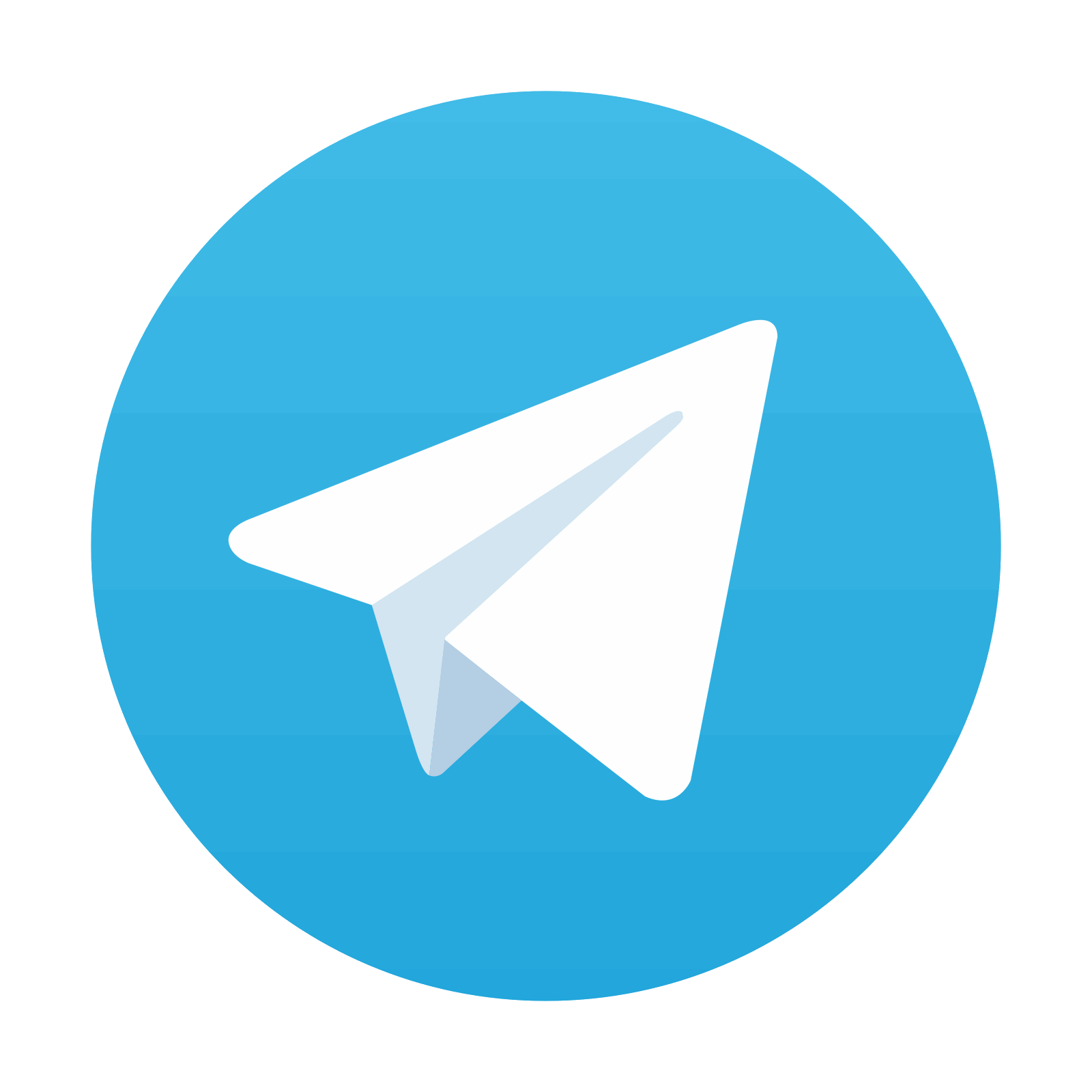
Stay updated, free articles. Join our Telegram channel

Full access? Get Clinical Tree
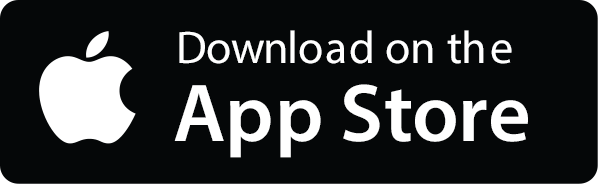
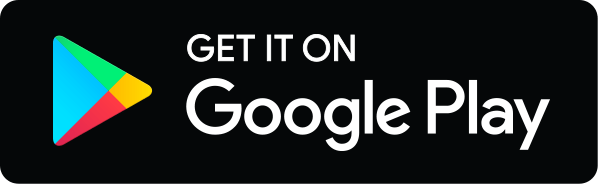