Figure 81-1. The anatomy of atrial septal defects. In the sinus venosus type (A), the right upper and middle pulmonary veins frequently drain to the superior vena cava or right atrium. B: Secundum defects generally occur as isolated lesions. C: Primum defects are part of a more complex lesion and are best considered as incomplete atrioventricular septal defects.
Failure of postnatal fusion of the septum secundum to the septum primum results in a persistent slit-like communication known as a patent foramen ovale (PFO). PFOs are extremely common in the general population, and autopsy studies have demonstrated a prevalence of 27%.8 PFOs are generally considered separate from other ASDs due to the absence of significant shunting, but they remain important clinically due to the occurrence of paradoxical embolization. A paradoxical embolus is a blood clot arising from a systemic vein that would normally pass to the lungs, but in the presence of a septal defect, may instead cross into the systemic circulation.
ASDs lead to increased pulmonary blood flow (PBF) secondary to left-to-right shunting. Shunting at the atrial level is determined by the size of the defect and by the relative ventricular compliance (i.e., blood preferentially fills the more compliant ventricle). At birth, both chambers are equally compliant, but as pulmonary vascular resistance (PVR) falls, the right ventricle remodels and becomes more compliant. Shunting across the atrial septum causes a volume load on the right heart. A volume load is created by additional venous return to a chamber during diastole.
The volume overload from an ASD is usually well tolerated, and patients are frequently asymptomatic. Symptoms tend to develop when the ratio of pulmonary to systemic blood flow (Qp/Qs) exceeds two. The most common symptoms are fatigue, shortness of breath, exercise intolerance, and recurrent respiratory infections. Older patients with untreated ASDs tend to develop atrial dysrhythmias, and adults may develop congestive heart failure (CHF) and right ventricular dysfunction. Pulmonary vascular obstructive disease may occur rarely as a late complication of an untreated ASD. Paradoxical embolization is also an important potential complication of an ASD.
The classic physical findings in patients with ASDs include fixed splitting of the second heart sound and a systolic ejection murmur at the left upper sternal border due to relative pulmonary stenosis (PS) (increased flow across a normal pulmonary valve). A diastolic flow murmur across the tricuspid valve is occasionally audible. A prominent right ventricular lift and increased intensity of the pulmonary component of the second heart sound may occur with pulmonary hypertension. Chest radiography shows cardiomegaly, with enlargement of the right atrium, right ventricle, and pulmonary artery. Electrocardiography (ECG) frequently demonstrates right axis deviation and an incomplete right bundle branch block. When right bundle branch block occurs with a leftward or superior axis, the diagnosis of AVSD should be considered. Echocardiography confirms the diagnosis of ASD and defines the anatomy. Cardiac catheterization is important in selected cases to assess PVR in older patients, but it is used more frequently with therapeutic intent for device closure of ASDs.
Due to the long-term complications associated with ASDs, repair is recommended for all patients with symptomatic defects and in asymptomatic patients in whom the Qp/Qs is greater than 1.5 or signs of right heart enlargement. Repair is usually performed in children prior to school age. Closure of ASDs may be performed surgically or using a device deployed in the cardiac catheterization laboratory.
Surgical repair is usually recommended for large secundum defects and for most other types of ASDs. The heart is exposed by median sternotomy. Other surgical approaches have been proposed, including minimally invasive techniques, but there are technical drawbacks associated with each of the alternative approaches. In most cases, a limited midline incision with a partial lower sternal split provides adequate exposure and a cosmetically acceptable scar. The heart is carefully inspected for anomalies of systemic and pulmonary venous return.
Cardiopulmonary bypass is required with minimal cooling. The superior and inferior vena cava are separately cannulated for venous drainage, and the arterial cannula is placed in the ascending aorta. Following aortic clamping and arrest of the heart with cardioplegia, the atrial septum is exposed through a right atriotomy. Small secundum defects or PFOs may sometimes be closed primarily by suturing the edge of the septum primum to the edge of the septum secundum. More commonly, larger defects are closed using a patch (polytetrafluoroethylene or autologous pericardium) and a running polypropylene suture. When anomalous pulmonary venous drainage is present, a baffle is created to redirect the flow across the ASD. In all cases, care is taken to de-air the left atrium to avoid the complication of air embolization. Surgical closure of an ASD can be accomplished with a mortality approaching zero and minimal morbidity.9 Common postoperative complications include atrial arrhythmias and postpericardiotomy syndrome.
The first transcatheter device closure of an ASD was performed in 1976.10 A number of devices are currently available for percutaneous closure of secundum ASDs.11 The contemporary success rate for device deployment is 96% with complete closure at 24 hours of 99% or greater.12,13 The presence of a deficient rim is the most common reason for failed implantation.13 Device closure has the advantages of fewer complications and a shorter hospitalization. Device closure of small to moderate secundum ASDs and PFOs has now become the standard of care at most large centers. Surgical closure remains the procedure of choice for large or multiple defects, insufficient rims, sinus venous–type defects, and primum ASDs.
The long-term survival for patients undergoing ASD repair in childhood is normal.14,15 The major long-term complication following surgical closure of ASD is the development of supraventricular arrhythmias, although the risk is lowered when the ASD is closed in childhood.15,16 The persistence of this risk despite relief of right-sided volume overload is thought to be related to incomplete atrial remodeling or due to the presence of the atriotomy scar. Longer follow-up will be required to determine whether device closure alters the risk of atrial dysrhythmias.
Occasionally, adults will present with a newly diagnosed ASD. Many studies have confirmed that ASD closure in adults over the age of 40 increases survival and limits the development of heart failure.17,18 When the Qp/Qs is less than 1.5 and the ratio of pulmonary to systemic vascular resistance (Rp/Rs) is greater than 0.7, significant pulmonary vascular obstructive disease is usually present. A PVR in excess of 10 to 12 Woods units/m2 represents a contraindication to ASD closure.
VENTRICULAR SEPTAL DEFECT
Ventricular septation is a complex process that requires accurate development and alignment of a number of structures including the muscular interventricular septum, the atrioventricular (AV) septum (arising from the endocardial cushions), and the infundibular septum (which divides the outflow tracts of the right and left ventricles). The membranous septum is a fibrous portion of the ventricular septum that is adjacent to the central fibrous body (where the mitral, tricuspid, and aortic valve annuli make contact).
3 Ventricular septal defects (VSDs) are the most common congenital heart anomalies (with the exception of bicuspid aortic valve, which occurs in about 1.3% of the population). VSDs are present in about 4 of 1,000 live births and represent about 40% of congenital heart defects.7 VSDs are classified based on their location in the ventricular septum (Fig. 81-2). The most common defects are perimembranous (80%), which are located in the area of the membranous septum. Inlet defects (5%) are located beneath the septal leaflet of the tricuspid valve and are sometimes called AV canal-type defects. Defects located high in the ventricular septum are outlet defects (10%). Outlet VSDs are typically adjacent to both the pulmonary and aortic valves. Outlet defects are also known by several other names, including supracristal, infundibular, or doubly committed subarterial. Outlet defects are more common in the Asian population. Trabecular (or muscular) VSDs (5%) are completely bordered by muscle. Trabecular VSDs are frequently multiple and may be associated with perimembranous or outlet defects. The size of VSDs varies. By definition, a VSD is nonrestrictive when its size (or the cumulative size of multiple defects) is greater than or equal to the size of the aortic annulus.
Figure 81-2. The anatomy of ventricular septal defects (VSDs) as seen through the right ventricle. A: Outlet, or subarterial, VSDs are generally bordered superiorly by the pulmonary valve annulus. B: Perimembranous VSDs are most common, extending from the membranous septum into the infundibular septum. C: Inlet defects are located predominantly beneath the septal leaflet of the tricuspid valve. D: Muscular VSDs are situated away from the valves, toward the cardiac apex.
A VSD causes increased PBF due to left-to-right shunting primarily during systole. This creates a volume load on the left heart (the left atrium and ventricle receive the increased venous return during diastole). The right ventricle is not volume loaded (blood is ejected from the left ventricle [LV] through the VSD and directly into the pulmonary circulation); however, it does experience a pressure load. The volume of shunt flow is determined by the size of the defect and by the ratio of pulmonary to systemic vascular resistance. After birth, the PVR is still high and shunting across a VSD is sometimes minimal. Over the first several weeks of life, shunting tends to increase as the PVR normally falls. Therefore, a patient with a large VSD may be asymptomatic at birth and develop severe CHF symptoms over the first 6 weeks of life.
The natural history for patients with isolated VSDs is highly variable. Most VSDs are restrictive and tend to close spontaneously during the first year of life. Large VSDs are nonrestrictive, resulting in right ventricular and pulmonary pressures that are systemic or nearly systemic, and high PBF with Qp/Qs ratios greater than 2.5 to 3. Moderate VSDs are restrictive, with pulmonary pressures that are one-half systemic (or less) and Qp/Qs ratios of 1.5 to 2.5. Small VSDs are highly restrictive; right ventricular pressures remain normal, and the Qp/Qs is less than 1.5. Patients with large VSDs tend to develop symptoms of CHF by 2 months of age. Patients with smaller VSDs may remain asymptomatic. In patients with outlet VSDs, prolapse of the aortic valve may occur, producing aortic insufficiency.19 Untreated, excessive PBF leads to pulmonary vascular obstructive disease by the second year of life. The histologic changes associated with pulmonary vascular obstructive disease have been classified by Heath and Edwards based on severity.20 Grade 1 consists of medial hypertrophy; grade 2 reflects intimal proliferation; grade 3 is characterized by intimal fibrosis and vascular occlusion; and grades 4 through 6 describe progressive vessel dilatation, angiomatoid malformation, and necrotizing arteritis. Grades 1 and 2 are considered reversible, whereas the latter stages are irreversible.
Signs of heart failure in infants with large VSDs include tachypnea, hepatomegaly, poor feeding, and failure to thrive. On physical examination, there is a holosystolic murmur at the left sternal border. Usually, the murmur is louder with smaller defects. The precordium is active. The pulmonary component of the second heart sound is accentuated in the presence of pulmonary hypertension. Chest radiography shows increased pulmonary vascular markings and cardiomegaly. ECG is significant for right ventricular hypertrophy. Patients with small VSDs have little shunting and are usually asymptomatic, having only a pansystolic murmur. Patients with moderate VSDs manifest symptoms and signs that are proportional to the degree of shunting. In patients who have developed significant pulmonary vascular obstructive disease, the volume of left-to-right shunting is decreased, and the murmur may disappear. Eisenmenger physiology results when the shunt flow reverses to right to left, creating cyanosis.
The diagnosis of VSD is confirmed by echocardiography, which accurately defines the anatomy and excludes the presence of associated defects. Cardiac catheterization is used selectively in older children and adults in whom elevated PVR is suspected. PVR is calculated by the following formula:
PVR = (PAmean – LA)/Qp
where PAmean is the mean pulmonary artery pressure and LA is the left atrial pressure. The units of resistance by this formulation (using pressures in millimeters of mercury and pulmonary flow in liters per minute) are Woods units (which can be expressed in dynes/s per cm5 by multiplying by 80). In the pediatric population, vascular resistance is frequently calculated using the cardiac output indexed to the body surface area (in square meters) with the resulting indexed resistance units of Woods units/m2. PVR may be fixed or reactive, and, at the time of cardiac catheterization, response to nitric oxide or 100% fraction of inspired oxygen (FiO2) may be assessed.
The management of a patient with a VSD depends on the size of the defect, the type of defect, the shunt volume, and the PVR. In general, patients with large defects who have intractable CHF or failure to thrive should undergo early surgical repair. If the congestive symptoms can be moderated by medical therapy, then surgery may be deferred until 6 months of age. Patients with moderate VSDs may be safely followed. If closure has not occurred by school age, then surgical closure is indicated. Small VSDs with a Qp/Qs ratio of less than 1.5 do not require closure unless there is evidence of left-sided chamber enlargement. There is a small long-term risk of endocarditis for these patients, but this can be minimized with the appropriate use of prophylactic antibiotics.21 Patients with outlet VSDs have a risk of developing aortic insufficiency due to leaflet prolapse, and, therefore, all of these patients should undergo surgical closure.22 Older children and adults must undergo catheterization to assess the pulmonary circulation. When there is a fixed PVR greater than 8 to 10 Woods units/m2, then surgery is contraindicated.
Surgical closure is performed through a median sternotomy. Cardiopulmonary bypass is employed with bicaval cannulation, and the patient is typically cooled to 32°C. After aortic cross-clamping, cold cardioplegia is delivered through the aortic root to arrest the heart. Exposure of the ventricular septum is most often achieved by making a right atriotomy and retracting the leaflets of the tricuspid valve. This provides access to perimembranous, inlet, and most trabecular VSDs. Outlet VSDs are frequently best exposed via a pulmonary arteriotomy because the defect lies just beneath the valve. Trabecular VSDs located near the ventricular apex can be very difficult to expose, and an apical ventriculotomy may be necessary. Once the defect is exposed, it is closed using a polytetrafluoroethylene patch and a running polypropylene suture, although other centers may prefer other patch material or interrupted suture technique. It is important to understand the anatomy of the conduction tissue when closing VSDs. The AV node is an atrial structure that lies at the apex of an anatomic triangle (known as the triangle of Koch) formed by the coronary sinus, the tendon of Todaro (a prominent band leading from the inferior vena cava and inserting in the atrial septum), and the septal attachment of the tricuspid valve. The node then gives rise to the bundle of His, which penetrates the AV junction beneath the membranous septum. The bundle of His then bifurcates into right and left bundle branches, which pass along either side of the muscular ventricular septum. In the presence of a perimembranous VSD, the bundle of His passes along the posterior and inferior rim of the defect, generally on the left ventricular side. In this critical area, sutures must be placed superficially on the right ventricular side a few millimeters from the edge of the defect. The bundle of His also tends to run along the posterior and inferior margin of inlet VSDs. The conduction tissue is usually remote from outlet and trabecular VSDs.
PA banding is a palliative maneuver that is used to protect the pulmonary circulation from excessive blood flow. PA banding is currently performed only in patients who are felt to be poor candidates for VSD closure, either due to associated illness or due to anatomic complexity, such as multiple trabecular VSDs (“Swiss cheese” septum). Banding is performed without the need for cardiopulmonary bypass. A band is placed around the main PA and tightened to achieve a distal PA pressure of about one-half systemic. The band is secured to the adventitia of the PA to prevent its migration. Distal migration may result in narrowing and poor growth of one or both branch pulmonary arteries, whereas proximal migration can cause deformity of the pulmonary valve. Later, when the patient is a candidate for VSD closure, the band must be removed. Repair of the main PA at the band site is also usually necessary and can typically be accomplished by scar resection and primary closure or patch repair.
Surgical closure of a VSD is associated with a mortality less than 1%.23 Potential complications include injury to the conduction tissue and injury to the tricuspid or aortic valves. Transient heart block may result from tissue swelling or injury from retraction, but permanent heart block occurs in less than 1% of cases. When heart block develops after surgery, patients are usually observed for a period of 7 to 10 days prior to permanent pacemaker implantation. Closure of perimembranous and inlet VSDs may result in distortion of the tricuspid valve, which may cause significant regurgitation. Aortic valve injury may occur as a result of inaccurate suturing, especially in perimembranous and outlet defects. A residual VSD is seen in about 5% of cases, and reoperation is indicated when significant shunting persists (Qp/Qs ratio >1.5). The Qp/Qs ratio can be calculated by measuring oxygen saturations and using the following formula derived from the Fick equation:
Qp/Qs = (Ao – SVC)/(PV – PA)
where Ao is the aortic (or systemic) saturation, SVC is the saturation in the superior vena cava, PV is the saturation in the pulmonary veins (which is usually estimated to be 95% to 100%), and PA is the saturation in the pulmonary arteries. Intraoperative echocardiography is used routinely to identify residual defects, which can then be repaired before the patient leaves the operating room.
Recently, transcatheter devices have been developed to allow closure of some VSDs in the cardiac catheterization laboratory or by periventricular deployment without the use of cardiopulmonary bypass.24,25 Device closure is optimal for large muscular defects with sufficient rims for securing the device. These defects are often difficult to visualize and close surgically. Periventricular closure allows the use of VSD device closure in smaller infants with symptomatic muscular VSDs with excellent results without the challenges of transvenous access in this population.25 The use of devices for perimembranous VSDs remains limited by the risk of damage to the conduction system or impingement on the function of the tricuspid or aortic valves. A recent series demonstrated a 22% rate of complete heart block, which is prohibitively high in comparison to the surgical rate of complete heart block of less than 1%.26
ATRIOVENTRICULAR SEPTAL DEFECT
4 Defects in the embryologic development of the endocardial cushions may result in a variety of morphologic abnormalities in the AV valves and the atrial and ventricular septa. These anomalies range from ostium primum ASD to complete AVSD (or AV canal defect), with a spectrum of intermediate forms. PDA and TOF are occasionally seen in association with these defects. A high percentage of patients with abnormalities of the AV structures have trisomy 21 (Down syndrome). All infants with trisomy 21 should have an echocardiogram to rule out congenital heart disease given a prevalence of 50%.
Complete AVSD is an anomaly characterized by a common AV orifice, rather than separate mitral and tricuspid orifices, and a deficiency of endocardial cushion tissue, which results in a primum ASD and an inlet type of VSD. Complete AVSDs were subclassified by Rastelli et al.27 into three types according to the morphology of the anterior leaflet of the common AV valve (Table 81-1). Incomplete AVSDs are variants in which there is partial fusion of the AV valves, resulting in separate right and left AV valve orifices. The ostium primum ASD is a type of incomplete AVSD in which the VSD component is absent.
Table 81-1 Rastelli Classification of Atrioseptal Ventricular Defects
When both left and right AV valves equally share the common AV valve orifice, the AVSD is termed balanced. Occasionally, the orifice may favor the right AV valve (right dominance) or the left AV valve (left dominance). In marked right dominance, the left AV valve and LV are hypoplastic, and frequently coexist with other left-sided abnormalities, including aortic stenosis (AS), hypoplasia of the aorta, and coarctation. Conversely, marked left dominance results in a deficient right AV valve with associated hypoplasia of the right ventricle, PS or atresia, and TOF. Patients with severe imbalance require staged single-ventricle reconstruction.
The conduction tissue is displaced in an ASVD and is at risk during surgical repair. The AV node is located posteriorly and inferiorly of its normal position toward the coronary sinus in what has been termed the nodal triangle. This triangle is bounded by the coronary sinus, the posterior attachment of the inferior bridging leaflet, and the rim of the ASD. The bundle of His courses anteriorly and superiorly to run along the leftward aspect of the crest of the VSD, giving off the left bundle branch and continuing as the right bundle branch.
A number of other cardiac anomalies are associated with AVSDs including a PDA (10%) and TOF (10%).28 Important abnormalities of the left AV valve include single papillary muscle (parachute mitral valve) (2% to 6%) and double orifice mitral valve (8% to 14%).29 A persistent left superior vena cava with or without an unroofed coronary sinus is encountered in 3% of patients with an AVSD. Double-outlet right ventricle (DORV) (2%) significantly complicates or may even preclude complete surgical correction.28 Left ventricular outflow tract obstruction from subaortic stenosis or redundant AV valve tissue occurs in 4% to 7%.30,31
Patients with an incomplete AVSD generally present in a similar fashion as a patient with a large secundum ASD. Patients with a complete AVSD with both atrial and ventricular level shunting generally present early in infancy with signs and symptoms of CHF. In addition, moderate or severe left AV valve regurgitation occurs in approximately 10% of patients with an AVSD, worsening the clinical picture. On physical examination, the precordium is hyperactive, often with a prominent thrill. Auscultatory findings include a systolic murmur along the left sternal border, a high-pitched murmur at the apex from left AV valve regurgitation, and a middiastolic flow murmur across the common AV valve. In the presence of elevated PVR, there may be a split first heart sound. Significant cardiomegaly and pulmonary overcirculation are found on the chest radiograph. ECG reveals biventricular hypertrophy, atrial enlargement, prolonged PR interval, leftward axis, and counterclockwise frontal plane loop. Doppler/echocardiography is diagnostic, defining the atrial and ventricular level shunting, valvular anatomy, and any associated anomalies. Cardiac catheterization should be performed for patients older than 1 year of age, for patients with signs or symptoms of increased PVR, or in some cases to further evaluate other associated major cardiac anomalies. If the PVR is high, it is important to remeasure it while the child is breathing 100% oxygen with and without nitric oxide. If the pulmonary resistance falls, it implies that much of the elevated resistance is dynamic and can be managed in the perioperative period by ventilatory manipulation, supplemental oxygen, and nitric oxide. More recently, sildenafil has been shown to decrease elevation in PVR in children with CHF.32 Markedly elevated pulmonary resistance (>10 Woods units/m2) that does not respond to oxygen administration is generally considered a contraindication to repair.
Operative treatment is almost always necessary as soon as symptoms are observed to prevent further clinical deterioration. Even in the absence of symptoms, operation is best performed before 6 months of age. PA banding, which permits delaying the repair until the child is larger, is no longer used today except in select complex or single-ventricle cases, extremely low birth weight or prematurity, and very poor clinical condition. This approach exposes the child to the risks of two operations, and the overall mortality exceeds that of primary repair in infancy.
Correcting AVSDs requires patch closure of both septal defects, with any necessary valve reconstruction. Separate atrial and ventricular patches or a single patch for both chambers can be used.33 During closure of the ventricular defect, the surgeon must carefully avoid injury to the conduction system, which passes along the posterior and inferior rim of the ventricular septum.
The short- and long-term success of the operation is highly dependent on the status of the PVR and the surgeon’s ability to maintain competence of the mitral valve. Although earlier reports recommend that the cleft in the left AV valve should not be closed and the valve treated as a trileaflet structure, most surgeons now believe that closure of the cleft is an important mechanism in preventing postoperative left AV valve regurgitation. Significant AV valve regurgitation at the conclusion of surgery, severe dysplasia of the left AV valve, and failure to close the cleft of the left AV valve have been identified as important risk factors for reoperation.34 Significant postoperative left AV valve regurgitation is also a risk factor for operative and long-term mortality.30,34 The cleft should not be completely closed in the presence of a single papillary muscle to avoid causing left AV valve stenosis. In the case of a double-orifice valve, the bridging tissue should not be divided to create a single opening in the valve.
Operative mortality is related largely to associated cardiac anomalies and left AV valve regurgitation. Mortality for repair of uncomplicated incomplete AVSDs ranges from 0% to 0.6%, and the addition of left AV valve regurgitation increases mortality to 4% to 6%.30,35 For complete AVSDs, the mortality without left AV valve regurgitation is approximately 5%, compared with 13% when significant degrees of regurgitation are present.30
The majority of reoperations after repair of AVSD are due to left AV valve regurgitation or subaortic stenosis with reoperation rates at 5 years of 11% and 10%, respectively.36 Significant postoperative AV valve regurgitation occurs in 10% to 15% of patients, necessitating reoperation for valve repair or replacement in 7% to 12%.34,37,38 Long-term survival is excellent with rates at 1, 3, and 5 years of 98%, 95%, and 95%, respectively.36
TETRALOGY OF FALLOT
5 TOF is the most common cyanotic congenital heart defect. It occurs in 0.6 per 1,000 live births and has a prevalence of about 5% among all patients with congenital heart disease.7 The pathologic anatomy is frequently described as having four components: VSD, overriding aorta, PS, and right ventricular hypertrophy (Fig. 81-3). Embryologically, the anatomy of TOF is thought to result from a single defect: anterior malalignment of the infundibular septum.39 The infundibular septum normally separates the primitive outflow tracts and fuses with the ventricular septum. Anterior malalignment of the infundibular septum creates a VSD due to failure of fusion with the ventricular septum and also displaces the aorta over the VSD and right ventricle. Infundibular malalignment also crowds the right ventricular outflow tract, causing PS and, secondarily, right ventricular hypertrophy. Prominent muscle bands also extend from the septal insertion of the infundibular septum to the right ventricular free wall and contribute to the obstruction of the right ventricular outflow tract. The pulmonary valve is usually stenotic and is bicuspid in 58% of cases.40 Pulmonary atresia occurs in about 7% of cases of TOF. The branch pulmonary arteries in TOF may exhibit mild diffuse hypoplasia or discrete stenosis (most frequently of the left PA at the site of ductal insertion). Coronary artery anomalies are frequently present. The origin of the left anterior descending from the right coronary artery, which occurs in 5% of cases, is clinically important because the vessel crosses the right ventricular infundibulum and is vulnerable to injury at the time of surgery. A right aortic arch is present in 25% of patients with TOF. Associated defects include ASD, complete AVSD, PDA, or multiple VSDs.
Figure 81-3. The four anatomic features of tetralogy of Fallot. The primary morphologic abnormality, anterior and superior displacement of the infundibular septum, results in a malalignment ventricular septal defect, overriding of the aortic valve, and obstruction of the right ventricular outflow. Right ventricular hypertrophy is a secondary occurrence.
Patients with TOF develop cyanosis due to right-to-left shunting across the VSD. The degree of cyanosis depends on the severity of obstruction of the right ventricular outflow tract. Frequently, cyanosis is mild at birth and may remain undetected for weeks or months. Neonates with severe infundibular obstruction or pulmonary atresia will develop symptoms shortly after birth and will require a prostaglandin infusion to maintain ductal patency to ensure adequate PBF. In other patients, the right ventricular outflow tract obstruction is minor, and the predominant physiology is that of a large VSD with left-to-right shunting and CHF.
The occurrence of intermittent cyanotic spells is a well-known feature of TOF. The etiology of spelling is still controversial but is clearly related to a transient imbalance between pulmonary and systemic blood flow. A spell may be triggered by hypovolemia or peripheral vasodilation (e.g., after a bath or vigorous physical exertion). Spells may occur in neonates, but are most frequently reported in infants between the ages of 3 and 18 months. Most spells resolve spontaneously within a few minutes, but some spells may be fatal. Older children have been observed to spontaneously squat to terminate spells. The squatting position is thought to increase systemic vascular resistance, which thereby favors PBF.
Cyanosis is the most frequent physical finding in tetralogy. Auscultation reveals a normal first heart sound and a single second heart sound. A systolic ejection murmur is present at the left upper sternal border. Older children may develop clubbing of the fingers and toes. Chest radiography typically demonstrates a boot-shaped heart due to elevation of the cardiac apex from right ventricular hypertrophy. Pulmonary vascular markings are usually reduced. A right aortic arch may be present. An ECG shows right ventricular hypertrophy. Echocardiography is definitive, and catheterization is not necessary in most cases.
The medical management of TOF is directed toward the treatment and prevention of cyanotic spells. The immediate treatment of the spelling patient includes administration of oxygen, narcotics for sedation, and correction of acidosis. Transfusion is indicated for anemic infants. a-Agonists are useful for increasing systemic vascular resistance (which favors PBF). Some centers have used beta blockers as a form of long-term therapy to suppress the incidence of spells.
All patients with TOF should undergo surgical repair. Asymptomatic patients should be repaired electively between 4 and 6 months of age. Early repair is indicated for neonates with severe cyanosis and for infants who have had a documented spell or worsening cyanosis.
Classically, the repair of TOF was accomplished in two stages. During the first stage, PBF was augmented by creating a connection (or shunt) between a systemic artery and the PA. At the second stage, the shunt was taken down, and a complete repair was performed. The first shunt procedure was the Blalock–Taussig shunt, in which the subclavian artery was mobilized and divided distally, and an end-to-side anastomosis was created between the inferiorly deflected subclavian and the ipsilateral PA. Other shunt operations were subsequently developed and involved connections between the ascending aorta and right PA (Waterston shunt) or between the descending aorta and left PA (Potts shunt). The modified Blalock–Taussig shunt is the most common type of shunt used today and consists of an interposition graft (polytetrafluoroethylene) between the innominate or subclavian artery and the ipsilateral PA. Creation of a shunt may be accomplished with or without the use of cardiopulmonary bypass.
Currently, one-stage repair of TOF is preferred by most centers. Initial palliation with a shunt is still indicated for some patients who are at high risk for early complete repair, such as those with multiple congenital anomalies, severe concurrent illness, intracranial hemorrhage, or an anomalous coronary artery crossing a hypoplastic infundibulum. In the absence of these risk factors, complete repair in the symptomatic neonate can be performed with lower mortality and reoperation rate compared to staged palliation.41,42
Complete repair of TOF is performed using a median sternotomy and cardiopulmonary bypass with bicaval venous cannulation. By a transatrial approach, the right ventricular outflow tract can be examined through the tricuspid valve. Muscle bundles obstructing the right ventricular outflow tract are divided or rarely resected. The VSD is closed with a patch. Pulmonary valvotomy is performed, when indicated, via a vertical incision in the main PA. When the pulmonary valve annulus or infundibulum is severely hypoplastic, a transannular outflow tract patch may be necessary to relieve the obstruction. When an anomalous coronary artery crosses the infundibulum, a transannular incision may be contraindicated. In these cases, and in patients with pulmonary atresia, placement of a conduit (cryopreserved homograft, valved xenograft, or bioprosthetic heterograft) between the right ventricle (via a separate ventriculotomy) and main PA will be necessary. Patients who have a transannular patch develop pulmonary insufficiency as a consequence. This is surprisingly well tolerated in most infants and children, as long as the tricuspid valve is competent. As these patients grow older, some will develop right ventricular failure due to chronic pulmonary insufficiency, and pulmonary valve implantation may be necessary, often in the second or third decade of life.43,44
The early mortality following repair of TOF is between 1% and 5%.40,45 The results are worse for patients with TOF and pulmonary atresia. Long-term complications include recurrent obstruction of the right ventricular outflow tract and development of right ventricular dysfunction due to chronic pulmonary insufficiency. Actuarial survival at 20 years is 86% with excellent functional status.46
DOUBLE-OUTLET RIGHT VENTRICLE
Double-outlet ventricle includes a variety of malformations in which, by 50% or more, both great arteries arise from one ventricle. Although double-outlet LVs occur, a far more common anomaly is the DORV. A VSD is usually present in DORV, in addition to other defects, including discordant ventriculoarterial connections, valvar or subvalvar stenosis of the PA and aortic outflow, and single ventricle.
The physiologic consequences of DORV vary depending on the associated defects. The three most critical factors determining the net effects on the circulation are the size of the VSD, the presence or absence of PS, and the presence and degree of left-sided obstruction. As a result, DORV may clinically resemble an isolated VSD, TOF, or transposition of the great arteries (TGA).
The size and location of the VSD are important considerations in planning operative management. The VSD may be primarily directed toward the aorta, toward the PA, equally toward both arteries (doubly committed), or remote from both great vessels (noncommitted). The location of the VSD affects the direction of flow of oxygenated blood and thus affects the degree of cyanosis. VSDs in DORV seldom close spontaneously. This is fortunate, as closure would result in severe hemodynamic decompensation or death.
If the VSD is large, nonrestrictive, and committed to the aorta, it can be closed with a tunnel-like patch that directs left ventricular flow into the aorta. A restrictive VSD must be enlarged to avoid creating subaortic stenosis. For patients with DORV and PS, repair requires right ventricular outflow tract reconstruction with a patch or a valved allograft conduit, in addition to patch closure of the VSD.
DORV with transposition-type physiology (malposed great vessels with the VSD committed to the PA), also known as a Taussig–Bing anomaly, may be treated by a variety of methods, depending on the specific anatomic details. The preferred approach would be to perform an arterial switch operation, thus making the VSD committed to the aorta, and baffling the left ventricular outflow from the VSD to this neoaorta. Another preferred approach, which depends on a favorable orientation of the two great vessels and VSD, would be an intraventricular tunnel from the VSD to the aorta. A third approach utilizes the Damus–Kaye–Stanzel operation (DKS). During the DKS, the proximal PA is anastomosed end to side into the aorta. The VSD is baffled to both semilunar valves, which both connect to the aorta. An extracardiac conduit is then placed to reconstruct right ventricle-to-PA continuity. This approach may also be useful when the VSD is doubly committed or noncommitted, so that making a direct connection to either single great vessel is problematic.
The current results for correction of DORV with subaortic VSD are excellent, with a 15-year survival, including hospital mortality, of 96%.47,48 Mortality for the more complex repairs tends to be slightly higher. Hospital mortality for an arterial switch operation with VSD closure for DORV ranges from 3.7% to 14.3%.49,50
TRANSPOSITION OF THE GREAT ARTERIES
6 TGA is a congenital cardiac anomaly in which the aorta arises from the right ventricle and the PA originates from the LV (ventriculoarterial discordance; Fig. 81-4). Looping refers to the right or left looping of the primitive heart tube during fetal development, which determines whether the atria and ventricles are concordant (right atrium attaches to right ventricle and left atrium attaches to LV) or discordant. Levo-TGA (l-TGA) is associated with AV discordance (right atrium attaches to LV and left atrium attaches to right ventricle), and is also termed congenitally corrected TGA. l-TGA is a rare variant of TGA and is beyond the scope of this chapter, which will focus on dextro-TGA (d-TGA). The defect can be subdivided into d-TGA with intact ventricular septum (IVS) (55% to 60%) and d-TGA with VSD (40% to 45%), one third of which are hemodynamically insignificant. Pulmonic stenosis (PS), causing significant left ventricular outflow tract obstruction, occurs rarely with an IVS and in approximately 10% of d-TGA/VSD.51
d-TGA is a relatively common cardiac anomaly and is the most common form of congenital heart disease presenting as cyanosis in the first week of life. The malformation accounts for approximately 10% of all congenital cardiovascular malformations in infants.52 The degree of cyanosis depends on the amount of mixing between the pulmonary and systemic circulations. In d-TGA, oxygenated pulmonary venous blood is returned to the lungs and desaturated systemic blood is returned to the body. Because the two circulations exist in parallel, some mixing between them must occur to allow oxygenated blood to reach the systemic circulation and the desaturated blood to reach the lungs. Mixing may occur at a number of levels, most commonly at the atrial level through an ASD or a PFO. Generally, two levels of mixing are necessary to maintain adequate systemic oxygen delivery with a VSD or PDA serving as an additional site for cardiac mixing. In d-TGA, there can be no fixed shunt in one direction without an equal amount of blood passing in the other direction; otherwise, one circulation would eventually empty into the other. Therefore, the amount of desaturated blood reaching the lungs (effective PBF) must equal the amount of saturated blood reaching the aorta (effective systemic blood flow). Clinical characteristics are dependent on the degree of mixing and the amount of PBF. These factors relate to the specific anatomic subtype of d-TGA. Neonates with d-TGA with IVS (or small VSD) have mixing limited to the atrial level and PDA. The ASD may be restrictive and the PDA generally will close over the first days to week of life. As the degree of mixing decreases, the patient becomes increasingly cyanotic and will eventually suffer cardiovascular collapse. Fortunately, the majority of these neonates will manifest cyanosis early in life, which is recognized by a nurse or physician within the first hour in 56% and in the first day in 92%.53 In d-TGA with a large VSD, there is additional opportunity for mixing and increased PBF. The neonate with d-TGA/VSD may only manifest mild cyanosis, which may be initially overlooked. However, generally within 2 to 6 weeks signs and symptoms of CHF will emerge. Tachypnea and tachycardia become prominent, whereas cyanosis may remain mild. Auscultatory findings are consistent with CHF with increased PBF, including a pansystolic murmur, third heart sound, middiastolic rumble, gallop, and narrowly split second heart sound with increased pulmonary component. Neonates with d-TGA and significant PS present with severe cyanosis at birth. Lesser degrees of PS will result in varying levels of cyanosis. The ECG is normal at birth, demonstrating the typical pattern of right ventricular dominance. Although the classic chest radiographic appearance of an egg-shaped heart with a narrow superior mediastinum may be seen, this finding is often obscured by an enlarged thymic shadow. The abnormal ventriculoarterial connection is clearly seen on echocardiography, which demonstrates that the posterior great vessel arising from the LV is a PA that bifurcates soon after its origin. The anterior great vessel is the aorta and arises from the right ventricle. Associated lesions, including VSD, left ventricular outflow tract obstruction, and coarctation, may also be diagnosed. Although used less frequently, cardiac catheterization may be helpful to confirm the basic anatomy, discern associated lesions, define the coronary anatomy, and improve cardiac mixing by means of balloon atrial septostomy.
Figure 81-4. Anatomy of the most common type of transposition of the great arteries. The location of the ascending aorta is usually anterior to and to the right of the pulmonary artery.
The infant with d-TGA and severe cyanosis requires prompt diagnosis and treatment to improve mixing and increase the arterial oxygen saturation. The first intervention to improve mixing in a cyanotic newborn suspected of having d-TGA is to ensure ductal patency by beginning an infusion of prostaglandin E1 (PGE1). In the presence of a restrictive ASD, a balloon atrial septostomy, a technique developed by William Rashkind54 in 1966, is performed. The procedure involves inserting a balloon-tipped catheter across the foramen ovale into the left atrium. Inflation and forcible withdrawal of the catheter tears the septum primum and enlarges the ASD. Mixing generally increases immediately, with a substantial increase in arterial oxygen saturation.
Without intervention, d-TGA is universally fatal. Untreated, 30% of neonates will die in the first week of life, 50% by the first month, 70% within 6 months, and 90% by 1 year.55 The definitive surgical treatment of patients with d-TGA has changed dramatically in the past decade with the advent of the arterial switch operation. Before this procedure, repair of d-TGA was generally delayed until patients were at least 6 months of age. Historically, palliative procedures were often necessary to improve the systemic saturation or protect the pulmonary vascular bed before definitive repair. If balloon atrial septostomy failed to enlarge the ASD adequately, a Blalock–Hanlon septectomy was performed. Rarely used today, this operation is a method of surgically enlarging the ASD without cardiopulmonary bypass. In patients with large VSDs, significant CHF and pulmonary hypertension are present early in life. Historically, the main PA was banded to reduce distal PA pressure and prevent the development of pulmonary vascular occlusive disease. Changes of pulmonary vascular disease may develop in about 25% of patients with hemodynamically large VSDs by 3 months of age; therefore, early reduction of PA pressure was essential. In those cases of transposition with severe left ventricular outflow tract obstruction, total pulmonary flow is reduced and systemic-to-PA shunting was undertaken until definitive repair could be accomplished.
Historically, definitive repair was achieved by redirecting venous inflow at the atrial level. First successfully performed by Senning56 in 1959, the operation was further modified by Mustard57 in 1964 (Fig. 81-5). In both techniques, the atrial septum is repositioned such that superior and inferior vena caval blood is rerouted to the mitral valve and then to the LV and PA. Pulmonary venous blood is redirected to the tricuspid valve and right ventricle. The right ventricle then ejects the oxygenated blood to the systemic circulation. The Mustard operation uses a large patch of pericardium or prosthetic material to create the intra-atrial baffle. In the Senning procedure, the patient’s atrial tissue is used, and little or no foreign material is necessary. Although physiologic repair at the atrial level is associated with a low operative mortality rate (<5%), even in infants, a number of late problems have occurred. Obstruction to vena caval inflow, particularly at the junction of the superior vena cava and the right atrium, still occurs in about 5% of patients and may be more common when the procedure is performed in an infant. Additionally, pulmonary venous obstruction may develop and is often difficult to repair. Perhaps because of the complex atrial suture lines, atrial dysrhythmias are common and occur in more than half of patients observed on a long-term basis. In addition, pacemakers may be necessary for troubling bradyarrhythmias in as many as 10% of these patients.
Figure 81-5. The Mustard operation for transposition of the great arteries. In this procedure, the atrial septum is excised and replaced with a pericardial baffle, so that pulmonary venous blood is redirected over the baffle to the tricuspid valve. Superior and inferior vena caval blood then drains to the mitral valve.
The most serious long-term complication of repair by either the Senning or Mustard technique has been right ventricular dysfunction. Right ventricular failure with an enlarged, poorly contractile chamber and secondary tricuspid regurgitation has been found in a significant number of these patients in long-term follow-up studies. The true incidence of significant right ventricular failure in these cases remains difficult to define and is clearly influenced by an earlier era of operation with different methods of myocardial protection and surgical technique. The fact that many of these infants underwent definitive repair after many months of significant cyanosis may also have influenced right ventricular function.
The long-term complications of atrial repair prompted a reexamination of direct arterial repair for transposing the great arteries. The “arterial switch” operation, first successfully performed by Jatene in 1975, has become the optimal surgical procedure for infants with this condition.58 Current techniques have reduced the operative mortality to levels comparable with those of atrial repair. Additionally, because the operation is performed early in life, this approach has virtually eliminated the interim morbidity and mortality associated with postponement of surgery until at least 6 months of age. The operative technique involves transection of both great vessels and direct reanastomosis to reestablish ventriculoarterial concordance (Fig. 81-6). Additionally, the coronary arteries are removed from the anterior aorta and relocated to the posterior great vessel (neoaorta). The extensive experience gained with this procedure has confirmed that any variant of coronary artery anatomy can be successfully repaired. Certain coronary anatomy variants were previously felt to be associated with higher risk; however, this concern has been neutralized in recent series.59,60 Many patients with d-TGA have an IVS, and left ventricular pressure falls early in life as PVR decreases. In this situation, it is essential that the arterial repair be performed within the first 1 to 2 weeks of life, while the LV is still able to meet systemic workloads. In patients presenting later, the LV can be retrained with a preliminary PA banding and an aorticopulmonary shunt, if necessary, followed by the definitive arterial repair. Although patients with large VSDs do not require early repair because they maintain systemic left ventricular pressures, experience has indicated that, even in this subgroup, the operation is best performed within the first month of life, before secondary complications such as pulmonary hypertension, CHF, or infection develop.
Patients with fixed left ventricular outflow tract obstruction are not candidates for the arterial repair because correction would result in systemic ventricular outflow tract obstruction. Most of these patients also have large VSDs. Palliation early in life with systemic-to-PA shunting is an option, with definitive repair postponed until somatic growth results in cyanosis as the shunt is outgrown. At that time, the Rastelli procedure is performed, in which left ventricular blood is redirected through the VSD and to the anterior aorta by placement of an intraventricular patch (Fig. 81-7). The PA is ligated, and right ventricle–to–distal PA continuity is reestablished with a valved conduit. An increasing number of experienced centers currently recommend early complete repair in the neonatal period using a Rastelli procedure. Early repair eliminates the interim morbidity and mortality associated with a systemic-to-PA shunt and chronic cyanosis.
Current hospital survival for the arterial switch operation ranges from approximately 90% to 98%.51,59–62 In earlier eras, d-TGA/IVS had a lower operative mortality than d-TGA/VSD; however, recent studies have neutralized this difference.59,60 Complex d-TGA including d-TGA/VSD/PS and d-TGA/VSD with aortic arch hypoplasia are independent predictors of increased operative mortality.63,64 Coronary artery anatomy does not affect mortality.59 Long-term survival at 5 to 10 years and 15 years ranges from 87% to 93% and 86% to 88%, respectively.60–62 The most common cause for reintervention is supravalvar PS, occurring in 3.9% to 16%.61,62 Late follow-up of arterial switch operation patients has led to increased concern regarding coronary artery patency and neoaortic root dilation.65
Figure 81-6. Arterial switch procedure for transposition of the great arteries. A: Division of aorta and pulmonary artery. B: LeCompte maneuver; posterior translocation of the aorta. C: Mobilization of the coronary arteries. D: Placement of pantaloon-shaped pericardial patch. E: Proper alignment of the coronary arteries on the neoaorta. F: Completed repair.
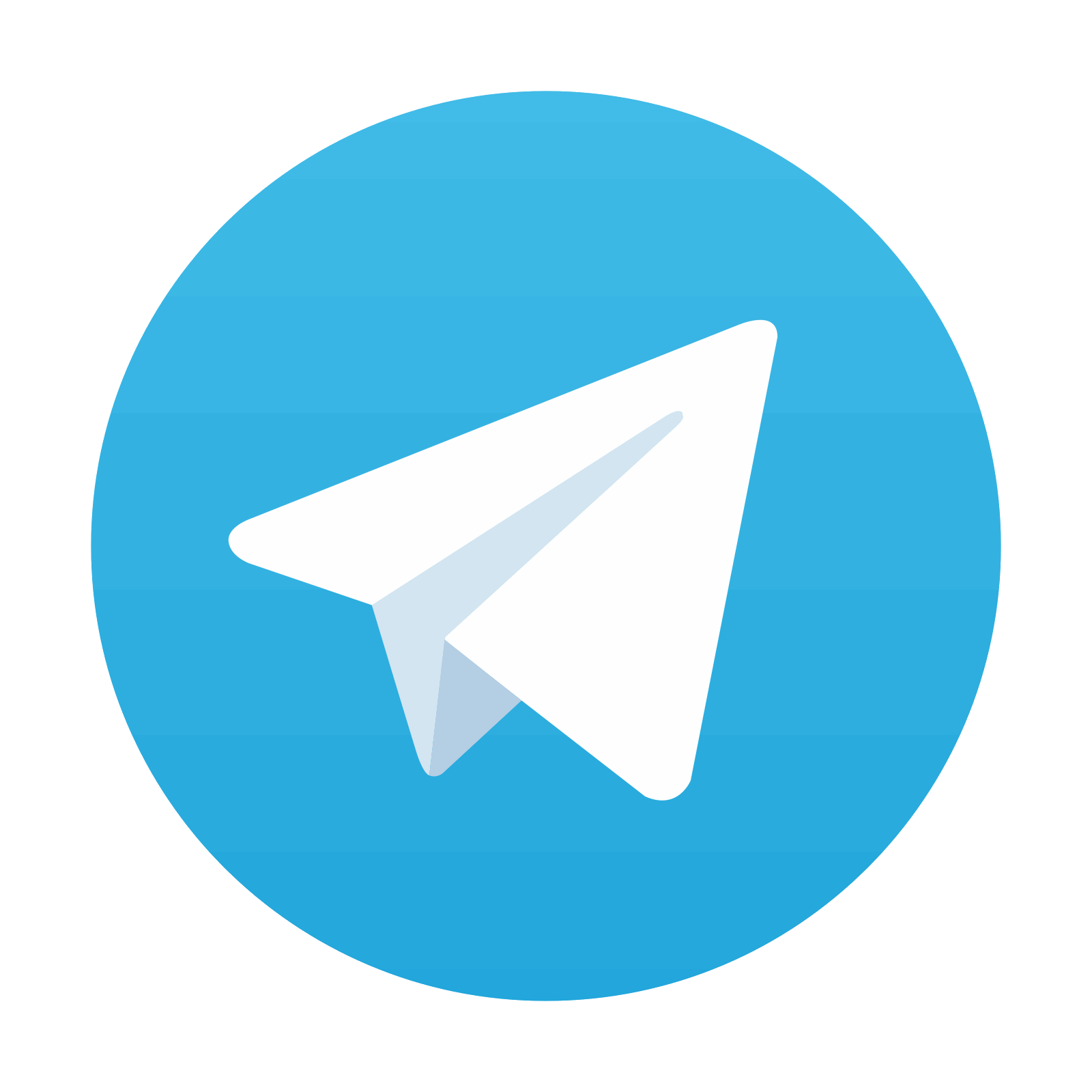
Stay updated, free articles. Join our Telegram channel

Full access? Get Clinical Tree
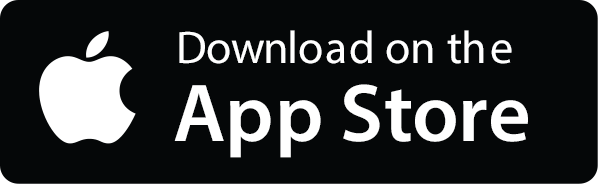
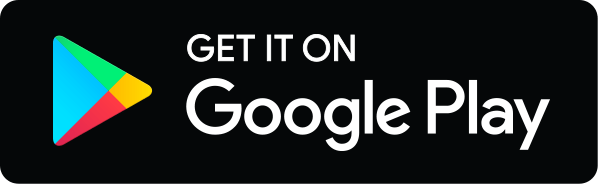