(1)
Canberra, Aust Capital Terr, Australia
Scientific Name
Matricaria chamomilla L.
Synonyms
Camomilla deflexa Gilib. (Inval.), Chamaemelum suaveolens E.H.L. Krause, Chamaemelum vulgare Bubani, Chamomilla chamomilla (L.) Rydb. (Illeg.), Chamomilla courrantiana (DC.), Chamomilla officinalis K. Koch, Chamomilla patens Gilib. (Inval.), Chamomilla recutita (L.) Rauschert, Chamomilla recutita subsp. bayeri, Chamomilla vulgaris Gray, Chrysanthemum chamomilla (L.) Bernh., Chrysanthemum suaveolens (L.) Cav., Courrantia chamomilloides Sch. Bip., Matricaria bayeri Kanitz, Matricaria capitellata Batt. & Pit., Matricaria chamomilla f. kochiana (Sch. Bip.) Fiori & Paol., Matricaria chamomilla subsp. pusilla (Willd.) Holmboe, Matricaria chamomilla var. recutita (L.) Grierson, Matricaria chamomilla var. recutita (L.) Fiori, Matricaria chamomilla f. suaveolens Fiori & Paol., Matricaria courrantiana DC., Matricaria exigua Tuntas, Matricaria kochiana Sch.Bip., Matricaria pusilla Willd., Matricaria recutita L., Matricaria recutita var. coronata (Boiss.) Halácsy, Matricaria recutita var. kochiana (Sch.Bip.) Greuter, Matricaria recutita var. recutita L., Matricaria salina (Schur) Schur, Matricaria suaveolens L.
Family
Asteraceae
Common/English Names
Annual Camomile, Blue Chamomile, Camomile, Chamomile, Chamomille, Common Chamomile, German Chamomile, Hungarian Chamomile, Pin-Heads, Scented Mayweed, Sweet Chamomile, Sweet False Chamomile, Sweet Feverfew, True Chamomile, White Chamomile, Wild Camomile, Wild Chamomile
Vernacular Names
Albanian: Maraqi
Arabic: Baabunaj, Babunej
Argentina: Manzanilla
Bolivia: Manzanilla
Brazil: Camomila, Camomila Comum, Camomila Vulgar, Camomilha-Verdadeira. Maçanilha, Matricária
Chinese: Yang Gan Ju
Colombia: Camomilla, Manzanilla Chiquita, Manzanilla Commun, Manzanilla Dulce
Czech: Heománek Pravý, Heřmánek Lékařský, Heřmánek Pravý
Danish: Ægte Kamille, Kamille, Lægekamille, Moderurt, Pigeurt, Velduftende Kamille, Vellugtende Kamille
Dutch: Echte Kamille, Roomse Kamille Sort
Eastonian: Chamomile, Teekummel
Egypt: Babounag
Esperanto: Kamomilo, Matrikario Refaldita, Vera Kamomilo
Finnish: Kamomillasaunio, Kamelinsaunio
French: Camomille, Camomille Allemande, Camomille Commune, Camomille Commune Ou d’Allemagne, Camomille Sauvage, Camomille Vraie, Herba De La Mera, Kamille, Matricaire, Matricaire Fausse Camomile, Matricaire Odorante, Matricaire Tronquée
Gaelic: Fíogadán Cumhra
German: Apfelblümlein, Apfelkraut, Echte Kamille, Feldkamille, Frauenblume, Ganille, Garnille, German Chamomile, Germeine Kamille, Gramillen, Haugenblume, Helmergen, Helmriegen, Hermel, Hermelin, Herminzel, Johannisköpfchen, Kamelle, Kamille, Kammerblume, Kummerblume, Kühmelle, Laugenblume, Mariamagdalenakraut, Muskatblume, Mutterkraut, Mägdeblume, Ramerian, Remi, Romerei
Guatemala: Manzanilla
Honduras: Manzanilla
Hungarian: Orvosi Székfű, Kamilla
Icelandic: Kryddbaldursbrá
India: Baboanh, Gul-Babunah, Roghan Babunah (Urdu)
Italian: Camomirra, Camomilla, Camomilla Commune, Capomilla
Japanese: Kamitsure, Kamiture
Mexico: Chamomille, Manzanilla
Nicaragua: Chamomille
Norwegian: Ettårig Kamille, Kamille, Kamilleblom, Kamomilleblom, Kannelblom, Kvitblom, Moderurt
Papiamento: Kanelublum
Persian: Baabunah
Polish: Rumianek, Rumianek Pospolity
Portuguese: Camomila, Camomila-Alemã, Camomila-Da-Alemanha, Camomila-Dos-Alemães, Camomila-Vulgar, Camomilla Legítima, Chamomilla, Macela, Macella, Mançanila, Margaça-Das-Boticas, Matricária
Russian: Romaška Aptečnaja
Slovašcina: Kamilica Prava, Prava Kamilica
Slovencina: Rumanček Kamilkový, Rumanček Pravý
Spanish: Amargaza, Bastardilla, Bonina, Camamila, Camamila Del Comercio, Camamilda, Camomilla, Chamomilla, Larrambillo, Magarza, Magarza Común, Magarza Montesina, Magarzuela Manzanilla, Manzanilla Alemana, Manzanilla Basta, Manzanilla Bastarda, Manzallina Blanca, Manzanilla Común, Manzanilla De Alemania, Manzanilla De Aragón, Manzanilla De Castilla, Manzanilla De Los Corrales, Manzanilla De Urgel, Manzanilla Del Huerto, Manzanilla Fina, Manzanilla Hedionda, Manzanilla Loca, Manzanilla Olorosa, Manzanilla Ordinaria, Manzanilla Real, Manzanilla Silvestre, Manzanilla Vera, Mazanilla Vulgar
Swedish: Äkta Kamomill, Kamomill, Sötblomster, Sötblomster Kamomill
Tunisia: Babunj, Matricaire
Turkish: Papatya
Origin/Distribution
Matricaria chamomilla is native to southern and eastern Europe and northern and western Asia. It has been introduced elsewhere and has naturalized in North America and Australia.
Agroecology
German chamomile is a cool climate species, growing in areas with temperatures of 7–26 °C and mean annual rainfall of 400–1,400 mm per season. It is frost tolerant down to −12 °C. It grows best in full sun and requires long summer days and high heat units for optimum essential oil yield (Alberts 2009). Optimum oil yields were found in temperature range of 20–26 °C, but increasing temperature had a negative impact on individual flower-head weight and days from buds to full-opened flowers (Bettray and Vömel 1992). Chamomile is not fastidious of soil types but thrives best on a well-drained, sandy or sandy-loam soils and tolerates pH from 4.8 to 8.5. It will also grow on clayey lime soils as it has a great tolerance to soil alkalinity.
Edible Plant Parts and Uses
German chamomile is cultivated for its essential oil and dried flowers. The oils are used as agents in alcoholic beverages, confections, desserts, perfumes, aromatherapy and cosmetics. Dried flowers are used for aromatic and soothing herbal teas and blend with other teas, in syrups and fruit jelly (Roberts 2000).
Botany
Chamomile is an erect, aromatic, herbaceous annual, 15–80 cm high with a much branched, light green stem. The leaves are alternate, bipinnate or tripinnate with long and linear pinna, mildy pubescent to glabrous (Plate 1). Flowers are borne in solitary terminal capitula, 10–20 mm across, on 15–25 cm grooved peduncle (Plate 1). The receptacle is 6–10 mm wide; is hollow, swollen and obovoid to subglobose; and lacks involucral scales and paleae. The ray florets are ligulate, white 6–10 mm by 2.5–3.5 mm. The central disc florets are bisexual, tubular with 5-teeth, 1.5–2.5 mm long, yellow. The fruit is a yellowish brown cypsela with 3–5 faint ribs.


Plate 1
Chamomile flowers and foliage
Nutritive/Medicinal Properties
Proximate nutrient composition per 100 g of brewed chamomile herbal tea was reported as follows: water 99.70 g, energy 1 kcal (4 kJ), carbohydrate 0.2 g, Ca 2 mg, Fe 0.08 mg, Mg 1 mg, K 9 mg, Na 1 mg, Zn 0.04 mg, Cu 0.015 mg, Mn 0.044 mg, F 13 μg, thiamine 0.010 mg, riboflavin 0.004 mg, pantothenic acid 0.011 mg, total folate 1 μg, total choline 0.4 mg, β-carotene 12 μg, vitamin A 1 μg RAE, vitamin A 20 IU, total saturated fatty acids 0.002 g, total monounsaturated fatty acids 0.001 g and total polyunsaturated fatty acids 0.005 g (USDA 2012).
Other Flower Phytochemicals
Five organic acids were isolated from chamomile flowers: tartaric acid, citric acid, malic acid, malonic acid and succinic acid (Olennikov and Tankhaeva 2005). Mann and Staba (1986) had listed an extensive range of phytochemicals found in chamomile. The main constituents of chamomile flowers included several phenolic compounds, primarily the flavonoids apigenin, quercetin, patuletin, luteolin and their glucosides (McKay and Blumberg 2006). The principal components of the essential oil extracted from the flowers were the terpenoids α-bisabolol and its oxides and azulenes, including chamazulene.
The following chemicals were reported in chamomile flower heads: ascorbic acid, thiamine, niacin, polyphenols (quercetin, rutin, geraniol, gallic acid, tannin, catechin tannic, caprylic acid, kaempferol, thujone) and fatty acids (oleic, linoleic, palmitic, sinapic) (Mann and Staba 1986). The terpenoids (−)-α-bisabolol oxides A, B and C were isolated from chamomile extract (Schilcher et al. 1976). Two spirocyclic polyines, the isomeric cis (Z)-enyne dicycloether cis-2-[hexadiyne)-(2,4)-ylidene]-1,6-dioxaspiro-[4,4]-nonene) and trans (Z)-enyne dicycloether cis-2-[hexadiyne)-(2,4)-ylidene]-1,6-dioxaspiro-[4,4]-nonene), were found in chamomile flowers (Bohlmann et al. 1961). The following phenyl carboxylic acids, synergic, vanillic, anisic and caffeic acids, were found in both ligulate and tubular chamomile florets (Reichling et al. 1979). Chamomile flower heads were also reported to contain cis/trans-en-in-dicycloethers (polyines) (Gasic et al. 1983), anthecotulid (37–120 mg/g) (Hausen et al. 1984) and up to 0.3 % choline (Bayer et al. 1958). In chamomile flowers from different origins, a range of 37.4–98.5 mg/100 g of herniarin and 6–17.8 mg/100 g of umbelliferone were determined (Schilcher and Kamille 1987). The average content in ligulate florets was significantly higher than in the tubular florets. The following coumarins, herniarin, umbelliferone, esculetin, isoscopoletin and scopoletin, were isolated from chamomile flowers (Kotov et al. 1991). A flavone 7-O-glucoside-specific glucosidase was purified and characterized from ligulate florets of Chamomilla recutita, and salicin, arbutin, naringenin 7-O-glucoside, luteolin 5-O-glucoside, and various p-nitrophenol compounds (-β-d-glucoside, -β-d-galactoside, -β-fucoside and a nitrophenyl-β-d-glucoside) were also isolated from the florets (Maier et al. 1993). The enzyme was confirmed to have a high affinity towards flavone 7-O-glucosides. Aqueous alcohol extracts of chamomile flowers were found to contain the following fat soluble compounds: β-farnesene (0.04–0.28 %), bisabolone oxide (<0.01–0.05 %), bisabolol oxide-A (0.15–0.59 %), spiroether (0.3–1.03 %) and pentacosane (0.08–0.11 %) (Kanamori et al. 1992). Two phenylpropanoids and one flavonoid glycosides were isolated from a 1-butanol-soluble portion of chamomile flowers (Kanamori et al. 1993). Their structures were elucidated as cis-2-β-d-glucopyranosyloxy-4-methoxy cinnamic acid (1) and trans-2-β-d-glucopyranosyloxy-4-methoxy cinnamic acid (2) and cosmosiin (apigenin-7-O-β-d-glucopyranoside) (3), respectively. All 3 compounds were found in higher concentration in ligulate flowers (compound (1) 4.32 %, compound (2) 1.32 %, cosmosiin 5.20 %) than tubular flowers (compound (1) 1.07 %, compound (2) 0.52 %, cosmosiin 0.02 %) or involucral scale (compound (1) 2.56 %, compound (2) 1.05 %, cosmosiin 0.17 %). Ahmad and Misra (1997) isolated oleonolic acid, β-sitosterol and β-sitosterol glucoside from the flowers by organic solvent extraction of chamomile flower oil. All three were also found in the capitulum receptacle (compound (1) 0.16 %, compound (2) 0.08 %, cosmosiin <0.01 %), stem (compound (1) 2.45 %, compound (2) 0.69 %, compound (3) 0.02 %) and leaf (compound (1) 1.75 %, compound (2) 0.67 %, cosmosiin 0.02 %). Two polyacylated spermines N1,N5,N10,N14-tetrakis[3-(4-hydroxyphenyl)-2-propenoyl]-1,5,10,14-tetraazatetradecane (tetracoumaroyl spermine) and N1,N5,N10-tris[3-(4-hydroxyphenyl)-2-propenoyl]-1,5,10,|14-tetraazatetradecane were found on chamomile flower extract (Yamamoto et al. 2002). Eleven bioactive phenolic compounds, namely, coumarins, herniarin and umbelliferone; phenylpropanoids, chlorogenic acid and caffeic acid; flavones, apigenin, apigenin-7-O-glucoside, luteolin and luteolin-7-O-glucoside; flavonols, quercetin and rutin; and flavanone, naringenin, were determined in chamomile extracts (Fonseca et al. 2007). The limits of detection and quantification for apigenin were 35.0 and 150.0 μg/ml, respectively.
The flavonoids quercetin-7-glucoside (quercimeritrin) and apigenin-7-glucoside (apigetrin) were isolated form chamomile florets (Hörhammer et al. 1963). Lipophilic flavonoids isolated from chamomile flowers included eupatoletin, eupalitin, chrysosplenol and chrysosplenitin (3,6,7,3′-tetramethyl-quercetagetin) (Hänsel et al. 1966). A new chamomile flavone apigenin 7-6″-O-(acetyl-)-glucoside was isolated from chamomile flowers and florets and the aglyca apigenin, luteolin, patulin, quercetin and isorhamnetin and the glycoside apigenin-7-glucoside, luteolin-7-glucoside, patulitrin and quercimeritrin were identified (Kunde and Isaac 1979). They further reported the presence of flavonoid and postulated a diacetylated apigenin-7-glucoside. They classified chamomile flavonoids according their polarity into (a) lipophlic aglyca (e.g., methoxylated compounds), (b) hydroxylated flavones aglyca, (c) acetylated flavones monoglycosides and (d) flavones diglycosides. Methylated flavonoid aglycones, eupatoletin, eupalitin and chrysosplenol, were found in the flowers (Exner et al. 1981). From ligulate flowers of Matricaria chamomilla were isolated a mixture of apigenin 7-O-β-glucoside diacetates, which was shown to be based on (2″, 3″)- and (3″, 4″)-diacetates (Redaelli et al. 1982). The flavones apigenin and its glucosides, apigenin-7-glucoside and apigenin-7-acetylglucoside, were found in ligulate florets but not in the tubular florets of Matricaria chamomilla (Redaelli et al. 1981b). Dried ligulate chamomile flowers contained 7–9 % glucosides of apigenin and 0.3–0.5 % free apigenin. Glucosides were identified as apigenin 7-glucoside and a 1:3 mixture of the 2″- and 6″-acetates (Redaelli et al. 1980). The most abundant flavonoid derivatives in chamomile flowers were apigenin 7-glucoside and its acetylated derivatives, as well as luteolin, quercetin and their glycosides (Carle and Isaac 1985). Beside hydroxylated aglyca, methoxylated flavonoids like chrysosplenol, jaceidin, chrysoeriol, patuletin, spinacetin, 6-methoxykaempferol, axillarin, chrysosplenetin, eupatoletin and eupalitin were identified in chamomile flowers (Carle and Isaac 1985).
Accumulation of two phenylpropanoid glycosides, (1) cis-2-β-d-glucophyranosyloxy-4-methoxy cinnamic acid and (2) trans-2-β-d-glucophyranosyloxy-4-methoxy cinnamic acid and one flavonoid glycoside, (3) cosmosiin (apigenin-7-O-β-d-glucopyranoside) glycosides in chamomile flower head, reach a maximum during flowering (Ohe et al. 1995). The content of (1) and (2) reached 2 % and 0.5 % in the head respectively during harvesting, and their content was higher in the ligulate flowers than tubular flowers. Coumarin, umbelliferone and its methoxy analogue herniarin were also found in M. chamomilla flowers (Redaelli et al. 1981a). The polyphenolic compounds identified in the methanolic flower-head extract by Mulinacci et al. (2000) included 5-caffeoylquinic acid, 3-caffeoylquinic acid, 4-caffeoylquinic acid, quinic acid, ferulic acid-l–O-glycoside, caffeoylquinic acid derivative, ferulic acid-7-O-glycoside, quercetin-3-O-galactoside, quercetin-7-O-galactoside, patuletin-7-O-glucoside, dicaffeoylquinic acid derivative, 1,3-dicaffeoylquinic acid, apigenin-7-O-glucoside, quercetin derivative, luteolin-4′-O-glucoside, quinic acid derivative, apigenin-7-O-glucosyl-2″-acetate, apigenin-7-O-glucosyl-6″-acetate, apigenin-7-O-glucosyl-diacetate, apigenin, luteolin-7-O-rutinose and ferulic acid derivative.
Free and total apigenin contents in chamomile extracts were, respectively, determined as 106 and 903 μg/g (methanolic extract), 77 and 817 μg/g (ethanolic extract) and 11.0 and 247 μg/g (glycolic extract) (Fonseca and Tavares 2004). The major flavonoids (apigenin glucosides) identified in the white florets of chamomile were apigenin 7-O-glucoside, apigenin-7-(6″-malonyl-glucoside), apigenin-7-(6″-acetyl-glucoside), apigenin-7-(6″-caffeoyl-glucoside), apigenin-7-(4″-acetyl-glucoside), apigenin-7-(4″-acetyl,6″-malonyl-glucoside) and a partially characterized apigenin-7-(mono-acetyl/mono-malonylglucoside) isomer (Svehlikova et al. 2004). The bioactive principles in the ethanol/water extract of chamomile flowers were determined as cis-2-β-d-glucopyranosyloxy-4-methoxy-cinnamic acid, trans-2-β-d-glucopyranosyloxy-4-methoxy-cinnamic acid, apigenin-7-O-β-d-(6″-O-rhamnosyl)glucopyranoside (isorhoifolin), apigenin-7-O-β-d-glucopyranoside (cosmosiin), apigenin-7-O-β-d-(6″-O-acetyl)glucopyranoside, 7-Methoxycoumarin (herniarin) and 4′,5,7-trihydroxyflavone (apigenin) (Weber et al. 2008). The following flavonoids, kaempferol, quercetin, myricetin and isorhamnetin, were isolated from the ethanol chamomile flower extract (Mohamed 2010).
The main secondary metabolites of Matricaria chamomilla ligulate flowers were apigenin-7-O-glucoside derivatives and (Z)-2-β-d-glucopyranosyloxy-4-methoxy cinnamic acid and (E)-2-β-d-glucopyranosyloxy-4-methoxy cinnamic acid (GMCAs), the precursors of herniarin (Repčák and Krausová 2009). The flavonoids detected were apigenin 7-O-glucoside, apigenin 7-O-(6″-malonyl)-glucoside, apigenin 7-O-(4″-acetyl)-glucoside, apigenin 7-O-(6″-caffeoyl)-glucoside, apigenin 7-O-(6″-acetyl)-glucoside, apigenin 7-O-(4″-acetyl, 6″-malonyl)-glucoside, and apigenin. The content of the apigenin glucoside and its main acylated derivatives in ligulate flowers of diploid plants were found to be significantly higher before the start of flowering in comparison with tetraploid plants. During the flowering and post-flowering phase, their content decreased and no difference between diploid and tetraploid plants was observed. The (E)-isomer was the dominant form of 2-β–d-glucopyranosyloxy-4-methoxy cinnamic acid. These secondary stress metabolite precursors were accumulated in higher concentrations in young growing ligulate flowers, but during flowering and post-flowering phases, their content decreased. Significantly higher content was found in tetraploid plants in comparison with diploid plants. Aglycones of glycosides were found in low concentrations.
Two new flavonoids quercetin and isorhamnetin besides myricetin and kaempferol were detected in the ethanolic extract of the flower head of Matricaria chamomilla growing in Iraq (Mohamed 2010). The most abundant phenolic compounds in both chamomile flowers and chamomile tea extracts were chlorogenic acid, umbelliferone, apigenin and apigenin-7-glucoside (Nováková et al. 2010). In chamomile tea extracts, there was greater abundance of flavonoid glycosides such as rutin or quercitrin, while the aglycone apigenin and its glycoside were present in lower amounts. The total content of polyphenols (in chlorogenic acid equivalents) in chamomile teas showed a significant variability as well as content of total flavonols (0.29–1.21 %) or total phenolic acids (7.7–91.4 mg/200 ml). (Raal et al. 2012). The major phenolic compounds in chamomile tea infusions were chlorogenic acids, ferulic acid glycosides, dicaffeoyl quinic acids and apigenin glycosides. Based on the amounts of essential oil, terpenoids, total flavonols and major phenolic compounds, the quality of the commercial chamomile teas was very variable, and the chamomile teas available in pharmacies should be preferred for the medical purposes. The major polyphenols detected were neochlorogenic acid; chlorogenic acid; cryptochlorogenic acid; ferulic acid glucoside 1; ferulic acid glucoside 2; quercetin galactoside; quercetin glucoside; luteolin glucoside; 3,4-dicaffeoyl quinic acid; 3,5-dicaffeoyl quinic acid; 4,5-dicaffeoyl quinic acid; apigenin glucoside; and apigenin acetylglucoside.
Farnesene, bisabolol, azulene (chamazulene), bisabolol oxide and terpene alcohol (farnesol) were the major constituents of the essential oil from dried chamomile flowers (Mishra et al. 1999). Oil from fresh flowers contained a high amount of terpene alcohol which decreased in shade-dried flowers and was the lowest in sun-dried flowers. The bisabolol content was the highest in sun-dried and minimum in fresh flowers. Farnesene content was higher in shade-dried flowers; bisabolol and azulene were higher in sun-dried flowers. The bisabolol oxide content was comparatively higher in fresh flowers (10.4 %) and was the least (8.4 %) in shade-dried flowers. The essential oil of chamomile inflorescence developed from callus cultures was found to contain chamomillol, gossonorol, cubenol, α-cadinol, (−)-α-bisabolol, 1-azulenethanol acetate and (−)-α-bisabolol acetate (Magiatis et al. 2001).
The Brazilian chamomile flower essential oil contained the following major components: bisabolol oxide B (23 %), bisabolol oxide A (17 %), (Z)-β-farnesene (16 %), α-bisabolol (13 %), chamazulene (8 %) and chamo-spiroether (5 %) (Matos et al. 1993). Studies in Yugoslavia found the chamomile essential oil content ranged from 0.24 to 0.50 % (Mimica-Dukic et al. 1993). The main compound in all samples was bisabolol oxide A (33.46–48.48 %); other major compounds were (−)-α-bisabolol, bisabolol oxide B, bisabolon oxide, farnesene, spathulenol, chamazulene, as well as en-in-dicycloether. Thirty-four compounds were identified in chamomile flower essential oil from Cuba, of which α-bisabolol oxide A (23.3 %), chamazulene (14.1 %), α-bisabolone oxide A (13.4 %) and β-caryophyllene (10.4 %) were the main constituents (Pino et al. 2000). Eighty compounds were identified in chamomile essential oil from Iran, of which α-bisabolol oxide A (43.8 %), α-bisabolone oxide A (13.6 %) and β-bisabolene (19.6 %) were predominant (Pino et al. 2002). Thirty-seven components were found in the essential oil distilled from the flower heads in Eastonia (Orav et al. 2001). Oxygenated sesquiterpenes were the most characteristic chamomile essential oil components comprising 50–70 % of the total oil, among which bisabol oxide A accounted for 20.2 %–33.1 %, bisabolon oxide A 6.7–13.6 %, bisabolol oxide B for 7.9–12.4 % and α-bisabolol 2.9–7.8 %. Chamazulene represented 5.3–7.2 % and en-yn-dicycloether contributed 17.1–21.7 % of the total oil. Sesquiterpenes (5–16 %) mainly comprised (E)-β-farnesene (4.3–12.6 %). Monoterpenes and oxygenated monoterpenoid compounds contributed very little (<1 %) to the chamomile oil composition. The minor components included α-pinene trace (tr), sabinene tr 0.1 %,6-methyl-5-hepten-2-one 0.1 %, myrcene tr 0.1 %, 1,2,4-trimethylbenzene tr 0.1 %, 3-octanol 0.1–0.2 %, p-cymene tr 0.1 %, 1,8-cineole 0.1 %, limonene 0.1 %, (E)-β-ocimene tr 0.1 %, Artemisia ketone 0.2–0.4 %, γ-terpinene tr 0.1 %, terpinolene tr-0.1 %, terpinen-4-ol tr 0.1 %, α-terpineol tr 0.1 %, carvone tr 0.1 %, γ-elemene 0.1–0.3 %, decanoic acid 1.1–2.1 %, β-caryophyllene 0.1 %, germacrene 0.5–1.7 %, γ-muurolene 0.5–1.7 %, α-farnesene 0.3–0.9 %, β-bisabolene tr 0.7 %, γ-cadinene tr 0.1 %, σ-cadinene tr 0.1 %, (E)-nerolidol 0.1 %, spathylenol 2.3–3.6 %, caryophyllene oxide tr 0.2 %, T-cadinol 0.2 %, unidentified 0.1 %, and unidentified 0.5–0.9 %. Total oil yield was 1.88–2.09 mg/g. Forty-one components were identified in chamomile grown in the lower region of the Himalayas, representing 97.5 % of the oil (Sashidhara et al. 2006). The main constituents were α-bisabolol oxide A (36.5 %) and B (8.6 %), (E)-β-farnesene (14.0 %), α-bisabolol (16 %) and chamazulene (5.6 %). The main constituents, except for α-bisabolol oxide B, were found in higher concentration in oil from the foothills of the Himalayas than in the oil from the northern Indian plain. Eighteen volatile components were identified in the Iranian chamomile flower essential oil (Owlia et al. 2007). The major components were guaiazulene 25.6 %, (E)-β-farnesene 20.1 %, chamazulene 12.4 %, α-bisabolol oxide B 7.3 %, α-bisabolol 7.3 %, hexadecanol 5.6 % and germacrene D 3.15 %. Other components included Z-γ-bisabolene 2.6 %, -bisabolol oxide A 1.9 %, spathulenol 1.7 %, n-nonadecane 1.4 %, caryophyllene oxide 1.2 %, α-muurolene 0.8 %, limonene 0.5 %, γ-terpinene 0.5 %, n-pentacosane 0.5 % and sclarene 0.4 %. The main compounds identified in chamomile flower essential oil were α-bisabolol (56.86 %), trans–trans-farnesol (15.64 %), cis-β-farnesene (7.12 %), guaiazulene (4.24 %), α-cubebene (2.69 %), α-bisabolol oxide A (2.19 %) and chamazulene (2.18 %) (Tolouee et al. 2010). Chamomile essential oil was found to contain 13 compounds, mainly bisabolol and its oxides, chamazulene, farnesene, germacrene and other sesquiterpenes (Hernández-Ceruelos et al. 2002). A total of 39 components were identified, representing over 92 % of the total oil yield of chamomile oils from European countries (Orav et al. 2010). The principal biologically active compounds in chamomile oils were bisabolol oxide A (3.1–56.0 %), α-bisabolol (0.1–44.2 %), bisabolol oxide B (3.9–27.2 %), cis-enyne-bicycloether (8.8–26.1 %), bisabolon oxide A (0.5–24.8 %), chamazulene (0.7–15.3 %), spathulenol (1.7–4.8 %) and (E)-β-farnesene (2.3–6.6 %). In 8 chamomile samples from 13, bisabolol oxide A (27.5–56.0 %) was predominant (among them in three Estonian samples). α-Bisabolol (23.9–44.2 %) was predominant in the samples from Moldova, Russia and the Czech Republic. The sample from Armenia was rich in bisabolol oxide B (27.2 %) and chamazulene (15.3 %). The oils were obtained in yields of 0.7–6.7 ml/kg and the minimum limit of 4 ml/kg stated by the European Pharmacopoeia was exceeded only in 13 samples from 13 analyzed drugs.
Both aqueous and methanolic chamomile flower extracts demonstrated the presence of mixture of several apigenin glucosides and parent glycone, apigenin (Srivastava and Gupta 2009). Other apigenin glucosides identified in chamomile extracts were mono-caffeoyl glucoside, mono-acetyl glucoside and mono-malonyl glucoside as well as mono-acetyl/mono-malonyl glucosides. The methanolic chamomile extract had high concentration of apigenin-7-O-glucoside along with several polyphenolic constituents which include caffeic acid, luteolin and luteolin-7-O-glucoside, among common flavonoids. The aqueous chamomile extract was found to contain a small fraction (5–7 % of total essential oil) content of the flower. The essential oil content in the methanolic extract consists of chamazulene, α-bisabolol, bisabolol oxides A and B, a cyclic ether and different hydrocarbons which were insoluble in the aqueous phase. Aqueous extracts obtained from both Lebanese and Egyptian chamomile varieties showed the abundance of apigenin-7-O-glucoside. Methanolic chamomile extract prepared from ray florets of Lebanese and Egyptian chamomile showed the presence of apigenin-7-O-glucoside (39 %) and the aglycone, apigenin, 6.7 % in the Egyptian and 2.3 % in the Lebanese variety. Disc florets of chamomile from Lebanese origin showed higher content of apigenin-7-O-glucoside (38.5 %) compared to Egyptian variety (36.1 %) but had no aglycone. Salamon et al. (2010) characterized Egyptian and Iranian chamomile landraces into 4 basic chemotypes based on the main components (%) in the essential oil:
Type A (South American collection): α-bisabolol oxide B (22.43–58.85 %) > α-bisabolol oxide A (4.74–15.68 %) > α-bisabolol (4.37–15.41 %), (en-yn-dicyloethers 2.61–11.27 %, chamazulene 2.70–17.69 %).
Type B (Egypt and central Europe): α-bisabolol oxide A (31.07–52.25 %) > α-bisabolol oxide B (5.27–18.79 %) > α-bisabolol (8.81–12.92 %), (en-yn-dicyloethers 4.08–9.90 %, chamazulene 5.40–7.95 %).
Type C (Spain/Catalonia, Malta, Crimea): α-bisabolol (24.18–77.21 %) > α-bisabolol oxide B (3.17–34.46 %) > α-bisabolol oxide A(2.13–18.50 %) (en-yn-dicyloethers 1.92–12.00 %, chamazulene 1.91–7.89 %).
Type D (southeast Europe, Turkey): α-bisabolol oxide B (10.43–24.20 %) > α-bisabolol oxide A (9.62–25.83 %) > α-bisabolol (8.49–19.58 %), (en-yn-dicyloethers 5.51–10.68 %, chamazulene 1.91–7.89 %). The highest contents of α-bisabolol oxide A and α-bisabolol oxide B in the flower anthodia were found in the Egyptian chamomile.
The total extract yield of chamomile oil obtained by solvent extraction (4.98 %) was much higher than the oil yield obtained by steam distillation even after 6 hours (0.31 %) (Falzari and Menary 2003). The oil yield increased with increasing duration of steam distillation but after 6 hours distillation the yield of solvent extract is still 16 times greater than the yield of steam distilled oil. The main volatile components of chamomile essential oil were E-β-farnesene(42.59 %), germacrene D (2.93 %), bicyclogermacrene (1.99 %), (E,E)-α-farnesene (8.32 %), α-bisabolol oxide B (4.43 %), α-bisabolone oxide A (4.53 %), chamazulene (1.18 %), α-bisabolol oxide A (21.16 %) and cis-ene-yne-dicycloether (5.94 %) (Heuskin et al. 2009). Other minor components included trans-ene-yne-dicycloether (0.99), (E)-phytol (0.23 %), globulol (0.23 %), spathulenol (0.63 %), dendrolasin (0.21 %), dehydronerolidol (0.09 %), trans-nerolidol (0.17 %), unidentified sesquiterpene (0.33 %), sesquirosefuran (0.18 %), δ-cadinene (0.18 %), (Z,E)-α-farnese (0,83 %), β-selinene (0.22 %), unidentified sesquiterpene (0.10 %), aromadendrene (0.07 %), β-caryophyllene (0.17 %), α-gurjunene (0.04 %), sativene (0.04 %), β-elemene (0.7 %), α-isocomene (0.26 %), β-maaliene (0.07 %), α-copaene (0.04 %), 4,8-dimethylnona-3,8-dien-2-one (0.04 %), isoborneol (0.03 %), artemesia alcohol (0.06 %), artemisia ketone (0.32 %), γ-terpinene (0.17 %), cis-β-ocimene (0.69 %), trans-β-ocimene (0.11 %), limonene (0.10 %), p-cymene (0.11 %), 2-pentylfuran (0.05 %), 6-methyl-5-hepten-2-one (0.03 %), sabinene (0.04 %) and α-pinene (0.03 %). Precocenes and piperitone (Yaguchi et al. 2006) and (E)- and (Z)-spiroethers (Yoshinari et al. 2008) were isolated from chamomile essential oil.
The constituents of chamomile flower oil comprised oxides (59.42 %), hydrocarbons (5.88 %), ethers (11.98 %), acids/esters (3.87 %), aldehydes/ketones (0.79 %), sesquiterpene lactones (6.18 %), alcohols (2.57 %), coumarins/miscellaneous compounds (0.35 %) and unknowns (5.62 %) (Tschiggerl and Bucar 2012). The major constituents were α-bisabolol oxide A (29.92 %), α-bisabolol oxide B (21.13 %), α-bisabolone oxide A (7.87 %), β-farnesene (3.90 %), cis-spiroether (11.67 %) and chamazulene (6.18 %). The major constituents of the flower infusion extract obtained by hydrodistillation were α-bisabolol oxide A (28.26 %), α-bisabolol oxide B (25.92 %), α-bisabolone oxide A (5.83 %), β-farnesene (1.10 %), cis-spiroether (4.69 %), chamazulene (19.11 %) and decanoic acid (1.56 %). The major constituents of the flower infusion extract obtained by solid phase extraction were α-bisabolol oxide A (22.80 %), α-bisabolol oxide B (8.09 %), α-bisabolone oxide A (1.59 %), cis-spiroether (25.62 %), trans-spiroether (6.40 %), chamazulene (1.77 %), achillin (2.88 %), matricarin/acetoxyachillin (5.20 %) and methylumbelliferone (5.55 %). Two sesquiterpene lactones leucodin (0.53 %) and acetoxyachillin were newly indentified together with achillin and matricarin in the chamomile infusion extract. They found that high amounts of spiroethers (ca. 30% in the infusion as compared to ca. 12 % in the genuine oil) and coumarins (ca. 7 %) and reduced amounts of bisabolol oxides (ca. 32 % in the infusion vs. ca. 60 % in the genuine oil) can be regarded as markers of the volatile fraction of chamomile tea. Their results demonstrated that solid phase extraction or other liquid extraction methods should be preferred over hydrodistillation when characterizing the aromatic composition of infusions.
The highest essential oil content was found in fully developed flowers approximately 1 week after beginning of flowering (Franz 1980). The composition of the essential oil depended on the stage of development. Flower buds contained more hydrocarbons and (−)-α-bisabolol, whereas with development of the flowers, chamazulene and the (−)-α-bisabolol oxides increased. The content of enin-dicycloethers increased in the receptacle. In another study, the quantity of α-bisabolol, α-bisabolol oxides A and B and α-bisabolon oxide A in chamomile flower reached a maximum at full bloom and then declined (Arak et al. 1980). The farnesene content of the flower decreased gradually with flower growth and development. The qualitative composition of essential oil remained stable at all stages of flower development. Accumulation of essential oil in chamomile flowers continued during drying, and its quantitative composition depended on the drying method. Harvesting at the early flowering phase and drying in a shaded place were recommended.
Among 30 compounds detected in dried chamomile flowers at two development stages, (E)-β-farnesene (49 %), artemisia ketone (10 %) and germacrene D (9 %) were the predominant volatile components in the HS-SPME (headspace solid-phase microextraction) extract, while α-bisabolol oxide A (42 %), chamazulene (21 %) and (Z)-spiroether (8 %) were the main essential oil constituents among the 13 compounds obtained by SDSE (steam distillation-solvent extraction) (Rafieiolhossaini et al. 2012). (E)-β-farnesene was the only compound which showed significant differences between the development stages of two flowers: stage I, when ligulate flowers start to develop and tubular flowers were still closed, and stage II, when tubular flowers were partially to completely opened. Eliasová et al. (2012) found that polyamine conjugate 1N,5N,10N,14N-tetracoumaroyl spermine (tetracoumaroyl spermine) was present mainly in tubular flowers, reaching its maximal content during the 3rd phase of flowering when the corollae of tubular flowers start to open. The later observed decrease could result from a release of pollen that also contained a considerable amount of tetracoumaroyl spermine. They postulated that tetracoumaroyl spermine may play an important role in pollen development. This polyamine from chamomile flower heads could be used for the treatment of several human disorders such as depression and anxiety.
The purified mucilage from chamomile flowers yielded xylose (21 %), arabinose (10 %), galactose (15 %), glucose (7 %), rhamnose (2 %) and glucuronic acid (45 %) (Janecke and Weisser 1964, 1965). The mucilage had a degree of polymerization of approximately 27 (molecular weight 3,500–4,200) and the linkages of sugars in the mucilage were suggested to be predominantly β-glycosidic. Carle and Isaac (1985) reported that chamomile mucilage consists of fructose, arabinose, xylose, glucose, galactose, rhamnose, galacturonic acid and glucosamine and the main chain the polysaccharide tp consists of α-1 → 4 connected d-galacturone acids. The polysaccharide was later confirmed to be methyl-glucurone oxylane (Füller et al. 1991, 1993). They also identified a neutral fructane of medium molecular mass 3,600 containing 74.3 % fructose, 3.4 % glucose (similar to inulin) and a strongly branched rhamnogalacturonane of medium molecular mass 9,300 consisting of 28 % uronic acid and 3.2 % protein (similar to pectin). These were found to have arabino-3,6-galactane glycoproteins as side chains. In an aqueous alcoholic chamomile extract, only fructanes were found.
Root Phytochemicals
The essential oil of Matricaria chamomilla roots contained the sesquiterpenes chamomillol, caryophyllene, caryophyllenepoxide and the polyenes Chamomillaester I and II (Reichling et al. 1984). The essential oil accumulated in schizogenous oil passages and oil cells restricted to the roots. Callus surface cultures of Matricaria chamomilla initiated from stems and flower heads produced an essential oil similar to that of the root. It exclusively accumulated in oil cells typical of the roots.
Plant Aerial Parts/Leaf/Cell Culture Phytochemicals
Sesquiterpenes, flavonoids, coumarins and polyacetylenes are considered the most important constituents of the chamomile drug (Schilcher and Kamille 1987). Secondary metabolites found in M. chamomilla included isobutyl angelate, 2-methylbutyl angelate, farnesene, β-farnesene, farnesol, (−)-α-bisabolol, bisabolol oxide A, bisabolol oxide B, matricin, chamazulene, guaiazulene, umbelliferone, herniarin, caffeic acid, chlorogenic acid, apigenin, luteolin, apigenin-7-O-glucoside, luteolin 7-O-glucoside, quercetin and Z-enyne dicycloether.
Flavonoids found in chamomile leaves included 7-glucosides of quercetin, isorhamnetin and luteolin together with small amounts of chrysoeriol and apigenin 7-glucoside and their aglyca (Greger 1975).
Polyphenolic compounds (g/kg dry matter) in the aerial chamomile plant parts were determined as follows: chlorogenic acid 1.16 g, 3,5-DCQA (dicaffeoylquinic acid) 2.92 g, 4,5, DCQA 1.61 g, total caffeoyl derivatives 5.69 g, total dihydroxycinnamic acid derivatives 15.89 g, total flavonoids 9.48 g, total dihydroxycinnamic acid derivatives + flavonoids 25.37 g and total polyphenolic compounds 36.79 g (Fraisse et al. 2011). The highest amount of phenolic and flavonoid was detected in the methanol extract of chamomile aerial parts with a mean value of 50.7 and 36.7 % and the lowest in the aqueous extract with a mean value of 3.94 and 1.36 %, respectively (Haghi et al. 2013). The apigenin 7-glucoside (0.21–1.23 g/100 g dry samples) in the crude extracts was much higher than the free apigenin (0.04–0.74 23 g/100 g dry samples). The compounds detected were chlorogenic acid, caffeic acid, p-coumaric acid, salicylic acid and flavonoids, rutin, apigenin-7-glucoside, quercetin, luteolin, apigenin, kaempferol and isorhamnetin.
Matricaria chamomilla cell suspensions cultured in a two phase system consisting of an aqueous nutrient medium and a nontoxic lipophilic phase (triglyceride) accumulated (Bisson et al. 1983) a great number of lipophilic substances in the triglyceride phase during the first week of culture period. One of these substances was identified as α-bisabolol. The composition of the essential oil of chamomile hairy root cultures on different media, namely, Murashige-Skoog (MS) medium and Gamborg (B5) media, was found to be similar, but differing in proportion (Máday et al. 1999). The main component of the essential oil was trans-β-farnesene, as in the intact roots. Chamomile essential oil was found to contain primarily chamazulene, (−)-α-bisabolol, bisabolol oxides, bisabolon oxide A, trans-β-farnesene, α-farnesene, spathulenol, β-eudesmol and cis/trans-en-in-dicycloethers. Formaldehyde was found in intact plants, micropropagated plants and hairy root cultures of chamomile (Máday et al. 2000). HCHO should not be considered as a side product, but a basic and indispensable component, required for various biological processes in chamomile.
Flavonoids, Coumarins, Mucilages, Mono- and Oligosaccharides Also Had Pharmacological Effects
Among the cultivated and wild chamomile species examined, the wild species from the areas of Szeghalom were found to contain the highest quantity of β-eudesmol (9.25 % in the total essential oil) in intact and organized roots of chamomile cultures (Szöke et al. 2004a). A new component α-selinene (Szöke et al. 2004c) and germacrene D, berkheyaradulene, 4-(2′, 4′, 4′-trimethyl-bicyclo[4.1.0]hept-2′-en-3′-yl)-3-buten-2-one, geranyl-isovalerate and cedrol, were found in the hairy roots of sterile chamomile cultures (Szöke et al. 2004d). These cultures generated the most important terpenoid and polyin compounds characteristics of the intact plant. Among wild chamomile populations in Hungary, a population was found in the area of Szabadkigyós containing significant amounts, on average 48 %-of (−)-α-bisabolol in its inflorescence oil. The intact roots of in vitro cultures contained no (−)-α-bisabolol but contained the sesquiterpene alcohol β-eudesmol. The main components of hairy root cultures derived from wild chamomile rich in (−)-α-bisabolol in the inflorescence oil were trans-β-farnesene, α-farnesene, geranyl isovalerate and cedrol. β-Selinene was identified as a new component of the genetically transformed cultures (Szöke et al. 2004b).
Effect of Abiotic Factors on Chamomile Oil and Chemical Composition
Optimum chamomile oil yield was found to be strongly influenced by genotype and optimum ecological conditions (Bettray and Vömel 1992). Herb quantity, individual flower-head weight and days from buds to full-opened flowers of genotypes were reduced with increasing temperature, but content of apigenin, (−)-α-bisabolol and essential oil but not chamazulene rose with increasing temperature, optimal yields were obtained from 20 to 26 °C. The content of apigenin-7-glucoside was influenced by genotype and not temperature. The increased content of the coumarin, umbelliferone, was observed in leaves 12 hours after abiotic stress elicitation by CuCl2 (Repcák et al. 2001). In 48 hours, this amount rose approximately ten times. In the same period of time, a decrease of (Z)- and (E)-2-β–d-glucopyranosyloxy-4-methoxy cinnamic acid and an increase of herniarin were found. The content of herniarin in the CuCl2-treated chamomile plants rose approximately three times, simultaneously with a decline of its precursor (Z)- and (E)-2-β-d-glucopyranosyloxy-4-methoxycinnamic acid (Eliasová et al. 2004). The highest amounts of umbelliferone in stressed plants exceeded 9 and 20 times those observed in control plants of the tetraploid and diploid cultivar, respectively. Due to stress, the concentration of ene-yne-dicycloether in leaves decreased by more than 40 %. The pattern of quantity changes of the examined compounds in tetraploid and diploid plants was similar. The aerial parts of chamomile were found to synthesize and accumulate(Z)- and (E)-2-β-D: -glucopyranosyloxy-4-methoxy cinnamic acids (GMCA), the precursors of phytoanticipin herniarin (7-methoxycoumarin), a compound with anticoagulant properties (Repcák et al. 2009).
Studies found that the yield of dry flower and essential oil per pot, essential oil percent and its composition in Matricaria chamomilla varied with irrigation regimes (Pirzad et al. 2006). Highest amount of essential oil percent, yield of dry flower and essential oil per pot were obtained from irrigation at 85 % of the field capacity. However, it was not significantly different from irrigation at 70 % of field capacity. Lowest amount of essential oil percent were obtained when the plants irrigated with 100 and 55 % of field capacity. Minimum yield of dry flower and essential oil per pot were observed when the plants irrigated with 55 % of field capacity, but it was not significantly different from irrigation at 100 % of field capacity. Major constituents of the essential oil for all irrigation treatments were azulene-7-ethyl-1,4-dimethyl, limonene, bisabolol oxides A and B, bisabolone oxide, trans-β-farnesene and isobornyl isobutyrate <8-isobutyryloxy >.
Nitrogen deficiency was found to induce changes of free amino acids and coumarin contents in chamomile leaves (Kovacik et al. 2006). Among secondary metabolites, the sum of 2-β–d-glucopyranosyloxy-4-methoxycinnamic acids increased sharply, herniarin increased slowly and the content of umbelliferone was low in N-deficient plants. A decrease in levels of all detected amino acids, besides histidine, was found. Within aromatic amino acids, tyrosine was the most abundant. The content of free phenylalanine was significantly lower in both control and N-deficient plants when compared to the content of tyrosine. The increase of herniarin glucosidic precursors was attributed to enhancing phenylalanine ammonia-lyase activity under nitrogen deficiency and nitrogen-free carbon skeletons were shunted in to the phenylpropanoid metabolism, including biosynthesis of (Z)-and (E)-2-β–d-glucopyranosyloxy-4-methoxycinnamic acids.
In the 3 years’ experiment (2005–2007), Gosztola et al. (2010) found the moderately warm and relatively wet year of 2006 produced the highest contents of essential oil and also that of its alpha-bisabolol component in 28 wild populations of chamomile and 4 registered cultivars. Although bisabolol oxide A also showed a high variability through the years, its direct connection with weather conditions could not be proven. A moderate variability was established for the proportions of chamazulene and the lowest one for bisabolol-oxide B. Considerable genotype–weather interaction was suggested, especially for the essential oil content and for the ratio of bisabolol oxide A. The results of studies suggested that the hexaconazole-induced tolerance to water deficit stress in chamomile was related to the changes in growth variables, antioxidants and the apigenin-7-glucoside content (Hojati et al. 2011). The exogenous application of 15 mg/l provided better protection when compared to the other concentration.
Chamomile had been reported to have antioxidant, antimicrobial antiplatelet activities in vitro and antiinflammatory, antimutagenic, cholesterol-lowering antispasmodic and anxiolytic activities in vivo (McKay and Blumberg 2006). However, human studies had been limited, and clinical trials examining the purported sedative properties of chamomile tea were lacking.
Antioxidant Activity
Chamomile flower essential oil exhibited good antioxidant when evaluated using the β-carotene bleaching assay (Owlia et al. 2007). Total antioxidant capacity (%) (DPPH scavenging activity) of chamomile aerial plant parts was 2.78 % and contribution from the main caffeoyl derivatives was as follows: chlorogenic acid 4.31 %, 3,5-DCQA (dicaffeoylquinic acid) 13.66 %, 4,5-DCQA 7.53 % and total caffeoyl derivatives 25.50 % (Fraisse et al. 2011).
It was found that chamazulene, a bioactive compound of chamomile, inhibited lipid peroxidation in a concentration and time-dependent manner presenting an IC50 of 18 μM after 45 minutes incubation (Rekka et al. 1996). It also inhibited the autoxidation of dimethyl sulfoxide (33 mM) by 76 % at 25 mM and had a weak capacity to interact with DPPH radical.
Anticancer Activity
Exposure of chamomile aqueous and methanolic extracts caused minimal growth inhibitory responses in normal cells, whereas a significant decrease in cell viability was observed in various human cancer cell lines (Srivastava and Gupta 2007, 2009). Chamomile exposure resulted in differential apoptosis in cancer cells but not in normal cells at similar doses. The aqueous and methanolic chamomile flower extracts exhibited antiproliferative and apoptosis inducing effects on human prostate cancer PC-3 cells (Srivastava and Gupta 2009). Exposure of PC-3 cells with aqueous chamomile extract for 24 hours resulted in dose-dependent increase in cell growth inhibition varying from 3 to 25 % at concentration ranging from 25 to 800 μg/ml. Similarly, 4–74 % cell growth inhibition was observed after exposure of PC-3 cells to methanolic chamomile extract ranging from 25 to 800 μg/ml. Exposure of PC-3 cells to both aqueous and methanolic chamomile extract at 200 μg/ml concentration for 24 hours caused induction of apoptosis. Similar cell growth inhibitory and apoptotic effects were noted in other human cancer cell lines including breast, colon, fibrosarcoma and cervical adenocarcinoma. Apigenin glucosides inhibited cancer cell growth but to a lesser extent than the parent aglycone, apigenin. Ex-vivo experiments suggested that deconjugation of glycosides occurred in vivo to produce aglycone, especially in the small intestine. The cytotoxicity of 10 essential oils including chamomile oil on human prostate carcinoma cell (PC-3) was significantly stronger than on human lung carcinoma (A549) and human breast cancer (MCF-7) cell lines (Zu et al. 2010). Studies showed the combination of 5-fluorouracil, an anticancer agent and bisabololoxide A, one of main constituents in German chamomile, inhibited the growth of human leukaemia K562 cells although the additive inhibition of growth by bisabololoxide A became smaller as the concentration of 5-fluorouracil increased (Ogata-Ikeda et al. 2011). The authors suggested that the simultaneous application of German chamomile containing bisabololoxide A may reduce the dose of 5-fluorouracil.
Studies demonstrated that chamomile and Marigold (Calendula officinalis) tea exerted selective dose-dependent cytotoxic action against target cancer cells; cytotoxicity of marigold tea was higher than chamomile (Matić et al. 2012). However, the cytotoxic effect of chamomile tea was very weak to healthy peripheral blood mononuclear cells (PBMC), while the effect of marigold tea on PBMC was more pronounced. Chemical analyses showed that dominant phenolic compounds in examined infusions and decoctions were flavonoid glycosides and hydroxycinnamic acid derivatives.
Antigenotoxic Activity
Three phenols (apigenin, bisabolol and protocatechuic acid) from two medicinal plants, Matricaria chamomilla and Uncaria tomentosa, showed an antigenotoxic effect against the hydrogen peroxide effect in the wing spot test of Drosophila melanogaster and also exhibited tumoricidal activity (Anter et al. 2011). Apigenin (2.24–35.96 mM) showed a lower 50 % inhibitory concentration than bisabolol and protocatechuic acid. They did not exhibit any genotoxic effect. These phenolics also induced apoptosis in HL-60 leukaemia cells. The authors suggested that the antioxidant activity of Chamomilla and Uncaria could be partially responsible of their beneficial activity.
Antiinflammatory Activity
In Vitro Studies
Chamomile treatment inhibited LPS-induced NO production and significantly blocked interleukin IL-1β, IL-6 and TNFα-induced NO levels in RAW 264.7 macrophages (Bhaskaran et al. 2010). Chamomile caused reduction in LPS-induced iNOS mRNA and protein expression. The study found that chamomile inhibited NO production and iNOS gene expression by inhibiting RelA/p65 activation and supported the utilization of chamomile as an effective antiinflammatory agent. Chamomile infusions from both the capitula and sifted flowers exhibited antiinflammatory activity when tested on phorbol 12-myristate 13-acetate-stimulated human adenocarcinoma gastric cells and human neutrophil elastase (Bulgari et al. 2012) This antiinflammatory activity was postulated due to the inhibition of neutrophil elastase and gastric metalloproteinase-9 activity and secretion, the inhibition occurring in a concentration-dependent manner. The promoter activity was also inhibited and the decrease of metalloproteinase-9 expression was found to be associated with the inhibition of NF-kB driven transcription. The results suggested that the flavonoid-7-glycosides, major constituents of chamomile flowers, may be responsible for the antiinflammatory action of the chamomile infusion observed.
Studies showed that matricine, chamazulene, (−)-α-bisabolol and guaiazulene, components of chamomile exhibited varying degree of antiinflammatory activity in rat paw carrageenan oedema (Jakovlev et al. 1983). Two to four hours after administration, matricine on a molar basis was equally effective as (−)-α-bisabolol. In contrast, chamazulene and guaiazulene showed significantly less activity than (−)-α-bisabolol and matricine. After 4 hours, the pharmacological effects of guaiazulene decreased significantly, whereas chamazulene showed nearly constant activities over the entire testing period. Safayhi et al. (1994) found that chamazulene inhibited the formation of leukotriene B4 in intact neutrophilic granulocytes, while matricine showed no effect up to 200 μM. Chamazulene (IC50: 2 μM), but not matricine, suppressed the chemical peroxidation of arachidonic acid. Further, matricine (up to 200 μM) had no effects on the cyclooxygenase and 12-lipoxygenase activities in human platelets. Therefore, they concluded that chamazulene, but not matricine, may contribute to the antiinflammatory activity of chamomile extracts by inhibiting the leukotriene synthesis and additional antioxidative effects.
Matricine and chamazulene and (−)-α-bisabolol, components of chamomile essential oil, had no distinct effects on protamine sulphate-provoked degranulation of mast cells from Lewis-1a rats (Miller et al. 1996). The trans-en-yne-dicycloether partly inhibited the degranulation of rat mast cells in concentrations above 10−4 M. Studies in lipopolysaccharide (LPS)-activated RAW 264.7 mouse macrophages suggested that modulation of inducible cyclooxygenase (COX-2) and inducible nitric oxide synthase (iNOS) by apigenin and related flavonoids (genistein, kaempferol) may be important in inflammation and carcinogenesis (Liang et al. 1999). Suppression of transcriptional activation of COX-2 and iNOS by apigenin might mainly be mediated through inhibition of IkB kinase activity induced by LPS or interferon gamma. Chamazulene carboxylic acid, a natural profen and matricin, a degradation product of proazulenic sesquiterpene lactones, both found in chamomile and yarrow, were found to have antiinflammatory activity (Ramadan et al. 2006). Matricin and the yarrow proazulenes were proposed to be antiinflammatory through conversion to chamazulene carboxylic acid.
Aqueous herbal extracts of chamomile (Matricaria chamomilla), meadowsweet (Filipendula ulmaria) and willow bark (Salix alba) and isolated polyphenolic compounds (apigenin, quercetin and salicylic acid, 0–100 μM) were found to have antiinflammatory activity in THP1 macrophages (Drummond et al. 2012). At concentrations of 10 μM, both apigenin and quercetin reduced interleukin IL-6 significantly. Apigenin at 10 μM and quercetin at 25 μM reduced tumour necrosis factor-alpha (TNF-α) significantly. Among the herbal extracts, willow bark had the greatest antiinflammatory activity at reducing IL-6 and TNF-α production. This was followed by meadowsweet and then chamomile.
Animal Studies
Alpha-bisabolol, a component of chamomile oil, suppressed carrageenan-induced paw oedema in rats (Jakolev and Schlichtegroll 1969). In mice, hydroalcoholic extracts of chamomile induced a reduction of oedema similar to the nonsteroidal antiinflammatory agent benzydamine used as reference (Tubaro et al. 1984). Three polysaccharides isolated from chamomile flower heads exhibited remarkable antiinflammatory activity against mouse ear oedema induced by croton oil (Füller et al. 1991, 1993, 2000). Animal studies showed that (−)-α-bisabolol, a bioactive sesquiterpene alcohol obtained from chamomile plant, was found to have peripheral antiinflammatory and antinociceptive activities (Rocha et al. 2011b). In the inflammatory models of paw oedema induced by carrageenan and dextran, the mice treated with (−)-α-bisabolol showed smaller oedemas compared to animals treated only with the vehicle. (−)-α-Bisabolol was capable of reducing paw oedemas induced by 5-HT but not oedemas induced by histamine. (−)-α-Bisabolol exhibited antinociceptive activity in the models of visceral nociception induced by acetic acid and in the second phase of the nociception test induced by the intraplantar administration of formalin. (−)-α-Bisabolol did not have any effect in a thermal nociception model using a hot plate but was able to reduce mechanical inflammatory hypernociception induced by carrageenan. These findings suggested the antinociceptive action of (−)-α-bisabolol was not linked to a central mechanism but instead was related to the inflammatory process. (−)-α-Bisabolol also decreased leukocyte migration, protein extravasations and the amount of TNF-α to the peritoneal cavity in response to carrageenan. Further, (−)-α-bisabolol diminished neutrophil degranulation in response to phorbol myristate acetate.
Clinical Studies
In a phase III double-blind, placebo-controlled study involving 164 patients (men and women), mouthwash containing chamomile flower extract did not decrease 5-fluorouracil-induced stomatitis (Fidler et al. 1996). Mazokopakis et al. (2005) reported a case of methotrexate-induced oral mucositis in a patient with rheumatoid arthritis, who was successfully treated with wild chamomile mouthwashes. Studies showed that hamsters with oral mucositis induced by 5-fluoracil treated with chamomile had a 12-fold greater chance of scoring zero (absence of mucositis) than the control group (Pavesi et al. 2011). Also animals treated with chamomile or the corticoid agent (betamethasone elixir—Celestone®) weighed significantly less than those in the control group. The group treated with the corticoid agent exhibited a more severe clinical condition, whereas the group treated with chamomile exhibited mild mucositis throughout the experiment. Analysis of the histopathological results demonstrated that the group treated with chamomile exhibited a lesser degree of mucositis throughout the evaluation period in comparison to the control and corticoid groups.
In a clinical dose response curve study of 25 patients with phlebitis, peripheral intravenous infusion of chamomile extract elicited an antiinflammatory effect (Reis et al. 2011). The time regression of phlebitis was shorter for groups with 2.5 % concentration (mean = 29.2 hours) and 5 % concentration (mean = 38.8 hours). Local toxicity was almost not observed.
Wound Healing Activity
Studies showed that rats with dead space wounds from excision and incision, treated with chamomile, exhibited a greater reduction in the wound area when compared with the controls (61 % versus 48 %), faster epithelialization and a significantly higher wound-breaking strength after 15 days (Nayak et al. 2007). Additionally, wet and dry granulation tissue weight and hydroxyproline content were significantly higher. In separate studies, male albino rats with induced secondary degree cutaneous burns, treated by rubbing with chamomile extract dissolved in olive oil twice a day, exhibited accelerated wound healing compared to control (Jarrahi 2008). In a subsequent study Jarrahi et al. (2010) demonstrated that chamomile extract dissolved in olive oil administered topically exhibited wound healing potential in linear incisional wound model in male Wistar rats. Studies by Martins et al. (2009) showed that male rats treated with chamomile presented significantly faster wound healing of experimental ulcers in comparison to those treated with corticosteroids, triamcinolone acetonide and clobetasol propionate. Other animal studies showed chamomile to have wound healing effect (Duarte et al. 2011). Rats with inflicted tongue ulcer treated with chamomile showed the best results regarding epithelialization and percentage of collagen fibers after 10 days. As expected, time had a statistically significant effect on fibroblast count, epithelialization, inflammation and wound size; animals sacrificed at 3 days showed the worst results.
In a double-blind trial of 14 patients with weeping wound area after dermabrasion of tattoos, the therapeutic efficacy of chamomile extract was shown by the significant decrease of the weeping wound area as well as the drying tendency (Glowania et al. 1987). In a controlled clinical study of colostomy patients with peristomal skin lesions, the lesions healed significantly faster in the chamomile than in the hydrocortisone group (Charousaei et al. 2011). Stoma patient symptoms (pain and itching) were also resolved more expediently in the chamomile than in the hydrocortisone group. The results suggested that German chamomile could be recommended to relieve itching and inflammation and that twice-daily application facilitated healing of peristomal skin lesions.
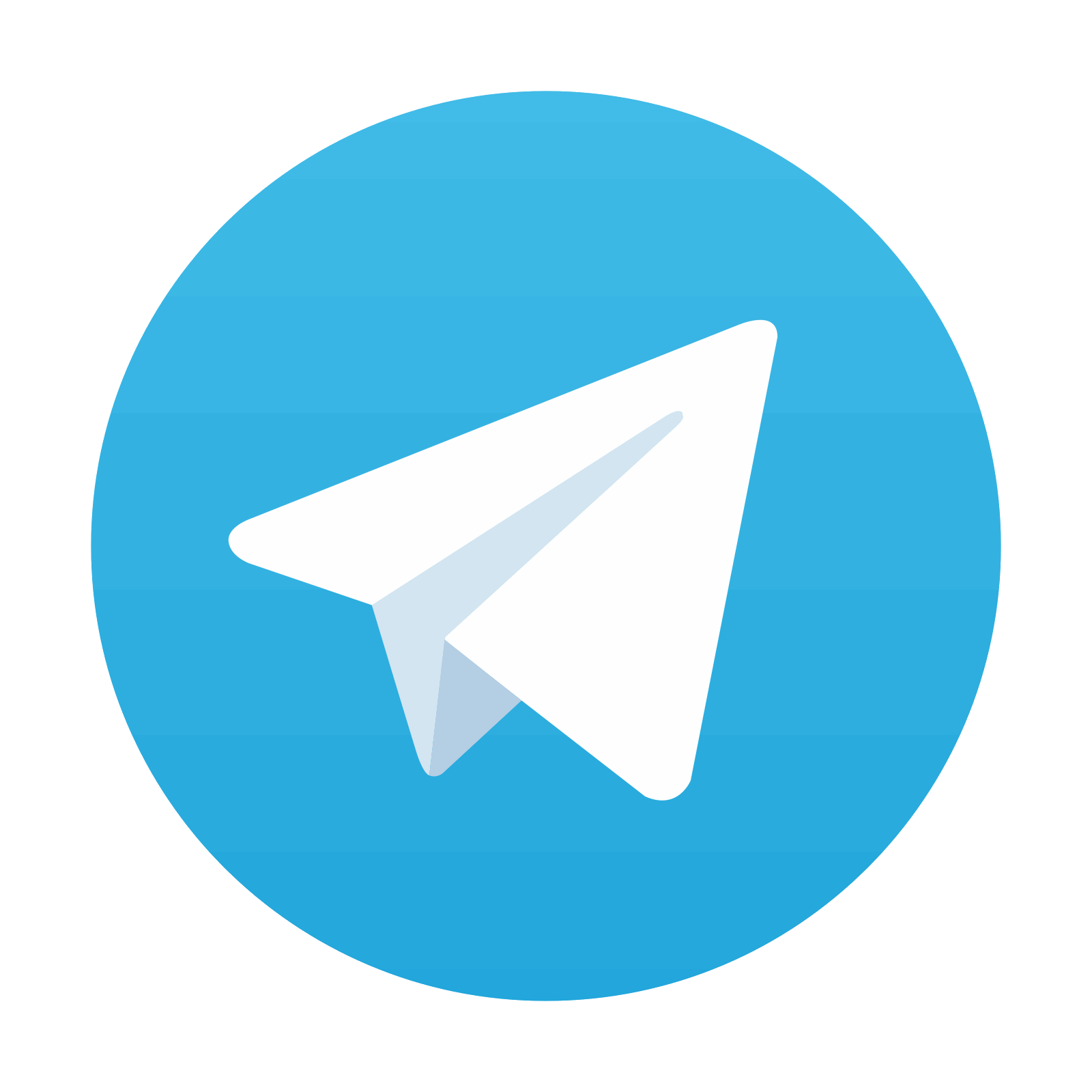
Stay updated, free articles. Join our Telegram channel

Full access? Get Clinical Tree
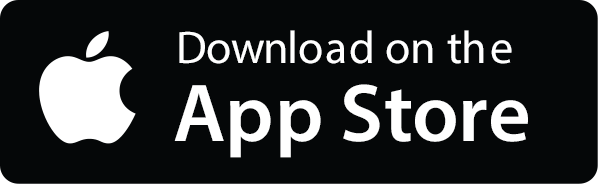
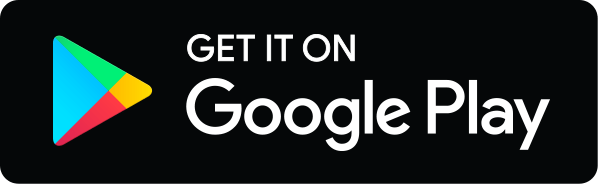
