58
Cellular Basis of the Immune Response
CHAPTER CONTENTS
ORIGIN OF IMMUNE CELLS
The capability of responding to immunologic stimuli rests mainly with lymphoid cells. During embryonic development, blood cell precursors originate mainly in the fetal liver and yolk sac; in postnatal life, the stem cells reside in the bone marrow. Stem cells differentiate into cells of the erythroid, myeloid, or lymphoid series. The latter evolve into two main lymphocyte populations: T cells and B cells (Figure 58–1 and Table 58–1). The formation of T cells and B cells from stem cells is enhanced by interleukin-7 (IL-7) produced by the stromal cells of the thymus and bone marrow, respectively.
FIGURE 58–1 Origin of T and B cells. Stem cells in the bone marrow (or fetal liver) are the precursors of both T and B lymphocytes. Stem cells differentiate into T cells in the thymus, whereas they differentiate into B cells in the bone marrow. Within the thymus, T cells become either CD4-positive (helper) cells or CD8-positive (cytotoxic) cells. B cells can differentiate into plasma cells that produce large amounts of antibodies (immunoglobulins). Dotted lines indicate interactions mediated by interleukins. (Modified and reproduced with permission from Brooks GF et al. Medical Microbiology. 20th ed. Originally published by Appleton & Lange. Copyright 1995 McGraw-Hill.)
TABLE 58–1 Comparison of T Cells and B Cells
The ratio of T cells to B cells is approximately 3:1. Figure 58–1 describes the origin of B cells and the two types of T cells: helper T cells and cytotoxic T cells. Table 58–1 compares various important features of B cells and T cells. These features will be described in detail later in the chapter.
Origin of T Cells
T-cell precursors differentiate into immunocompetent T cells within the thymus. Prior to entering the thymus, stem cells lack antigen receptors and lack CD3, CD4, and CD8 proteins on their surface. During passage through the thymus, they differentiate into T cells that can express both antigen receptors and the various CD proteins. The stem cells, which initially express neither CD4 nor CD8 (double-negatives), first differentiate to express both CD4 and CD8 (double-positives) and then proceed to express either CD4 or CD8. A double-positive cell will differentiate into a CD4-positive cell if it contacts a cell bearing class II major histocompatibility complex (MHC) proteins but will differentiate into a CD8-positive cell if it contacts a cell bearing class I MHC proteins. (Mutant mice that do not make class II MHC proteins will not make CD4-positive cells, indicating that this interaction is required for differentiation into single-positive cells to occur.) The double-negative cells and the double-positive cells are located in the cortex of the thymus, whereas the single-positive cells are located in the medulla, from which they migrate out of the thymus into the blood and extrathymic tissue.
Within the thymus, two very important processes called thymic education occur:
(1) CD4-positive, CD8-positive cells bearing antigen receptors for “self” proteins are killed (clonal deletion) by a process of programmed cell death called apoptosis (Figure 58–2). The removal of these self-reactive cells, a process called negative selection, results in tolerance to our own proteins (i.e., self-tolerance) and prevents autoimmune reactions (see Chapter 66).
FIGURE 58–2 Development of T cells. Note the positive and negative selection that occurs in the thymus. MHC, major histocompatibility complex; TCR, T-cell receptor.
For negative selection to be efficient, the thymic epithelial cells must display a vast repertoire of self proteins. A transcriptional regulator called the autoimmune regulator (AIRE) enhances the synthesis of this array of self proteins. Mutations in the gene encoding the AIRE protein results in the development of an autoimmune disease called autoimmune polyendocrinopathy.
(2) CD4-positive, CD8-positive cells bearing antigen receptors that do not react with self MHC proteins (Figure 58–2) are also killed. This results in a positive selection for T cells that react well with self MHC proteins.
These two processes produce T cells that are selected for their ability to react both with foreign antigens via their antigen receptors and with self MHC proteins. Both of these features are required for an effective immune response by T cells.
Note that MHC proteins perform two essential functions in the immune response: one is the positive selection of T cells in the thymus, as just mentioned, and the other, which is described later, is the presentation of antigens to T cells, the initial step required to activate those cells. MHC proteins are also the most important antigens recognized in the graft rejection process (see Chapter 62).
During their passage through the thymus, each double-positive T cell synthesizes a different, highly specific antigen receptor called the T-cell receptor (TCR). The rearrangement of the variable, diversity, and joining genes (see Chapter 59) that encode the receptor occurs early in T-cell differentiation and accounts for the remarkable ability of T cells to recognize millions of different antigens.
Some T lymphocytes, perhaps as much as 40% of the total, do not develop in the thymus but rather in the gut-associated lymphoid tissue (GALT). These intraepithelial lymphocytes (IELs) are thought to provide protection against intestinal pathogens. Their antigen receptors and surface proteins are different from those of thymus-derived lymphocytes. IELs cannot substitute for thymus-derived lymphocytes because patients with DiGeorge’s syndrome who lack a thymus (see Chapter 68) are profoundly immunodeficient and have multiple infections.
The thymus involutes in adults, yet T cells continue to be made. Two explanations have been offered for this apparent paradox. One is that a remnant of the thymus remains functional throughout life and the other is that an extrathymic site takes over for the involuted thymus. Individuals who have had their thymus removed still make T cells, which supports the latter explanation.
Origin of B Cells
B-cell precursors differentiate into immunocompetent B cells in the bone marrow; they do not pass through the thymus. Analogous to T cells, B cells also undergo clonal deletion (apoptosis) of those cells bearing antigen receptors for self proteins, a process that induces tolerance and reduces the occurrence of autoimmune diseases (see Chapter 66). Note that B cells bearing an antigen receptor for a self protein can escape clonal deletion by a process called receptor editing. In this process, a new, different light chain is produced that changes the specificity of the receptor so that it no longer recognizes a self protein. It is estimated that as many as 50% of self-reactive B cells undergo receptor editing. T cells do not undergo receptor editing.
Origin of Natural Killer Cells
Natural killer (NK) cells are large granular lymphocytes that do not pass through the thymus, do not have an antigen receptor, and do not bear CD4 or CD8 proteins. They recognize and kill target cells, such as virus-infected cells and tumor cells, without the requirement that the antigens be presented in association with class I or class II MHC proteins. Rather, NK cells target those cells to be killed by detecting that they do not display class I MHC proteins on the cell surface. This detection process is effective because many cells lose their ability to synthesize class I MHC proteins after they have been infected by a virus (see page 501).
Origin of Macrophages
In contrast to T cells, B cells, and NK cells, which differentiate from lymphoid stem cells, macrophages arise from myeloid precursors. Macrophages have two important functions, namely, phagocytosis and antigen presentation. They do not pass through the thymus and do not have an antigen receptor. On their surface, they display class II MHC proteins, which play an essential role in antigen presentation to helper T cells. Macrophages also display class I MHC proteins, as do all nucleated cells. The cell surface proteins that play an important role in the immune response are listed in Table 58–2.
T CELLS
T cells perform several important functions, which can be divided into two main categories, namely, regulatory and effector. The regulatory functions are mediated primarily by helper (CD4-positive) T cells, which produce interleukins (Table 58–3). For example, helper T cells make (1) interleukin (IL)-2, which activates CD4 and CD8 cells; (2) IL-4, which help B cells make antibodies, especially IgE; and (3) gamma interferon, which enhances killing by macrophages. The effector functions are carried out primarily by cytotoxic (CD8-positive) T cells, which kill virus-infected cells, tumor cells, and allografts.
CD4 & CD8 Types of T Cells
Within the thymus, perhaps within the outer cortical epithelial cells (nurse cells), T-cell progenitors differentiate under the influence of thymic hormones (thymosins and thymopoietins) into T-cell subpopulations. These cells are characterized by certain surface glycoproteins (e.g., CD3, CD4, and CD8). All T cells have CD3 proteins on their surface in association with antigen receptors (TCR [see later]). The CD3 complex of five transmembrane proteins is involved with transmitting, from the outside of the cell to the inside, the information that the antigen receptor is occupied. One of the CD3 transmembrane proteins, the zeta chain, is linked to a tyrosine kinase called fyn, which is involved with signal transduction. The signal is transmitted via several second messengers, which are described in the section on activation (see later). CD4 is a single transmembrane polypeptide, whereas CD8 consists of two transmembrane polypeptides. They may signal via tyrosine kinase (the lck kinase) also.
T cells are subdivided into two major categories on the basis of whether they have CD4 or CD8 proteins on their surface. Mature T cells have either CD4 or CD8 proteins but not both.
CD4 lymphocytes perform the following helper functions: (1) they help B cells develop into antibody-producing plasma cells; (2) they help CD8 T cells to become activated cytotoxic T cells; and (3) they help macrophages effect delayed hypersensitivity (e.g., limit infection by Mycobacterium tuberculosis). These functions are performed by two subpopulations of CD4 cells: Th-1 cells help activate cytotoxic T cells by producing IL-2 and help initiate the delayed hypersensitivity response by producing primarily IL-2 and gamma interferon, whereas Th-2 cells perform the B-cell helper function by producing primarily IL-4 and IL-5 (Figure 58–3). Note also that the cytokines produced by Th-1 cells (e.g., gamma interferon) help B cells to class switch (see page 497) to produce two subclasses of IgG (namely IgG 1 and IgG 3) that are very effective opsonizers of bacteria.
FIGURE 58–3 The origin of Th-1 and Th-2 cells. On the left side, the origin of Th-1 cells is depicted. Microorganisms are ingested by macrophages, and interleukin (IL) -12 is produced. IL-12 induces naïve Th-0 cells to become Th-1 cells that produce gamma interferon and IL-2. These interleukins activate macrophages and cytotoxic T cells, respectively, and cell-mediated immunity occurs. On the right side, the origin of Th-2 cells is depicted. Microorganisms are ingested by an unknown type of cell (see footnote below), and IL-4 is produced. IL-4 induces naïve Th-0 cells to become Th-2 cells that produce IL-4 and IL-5. These interleukins activate B cells to become plasma cells, and antibodies are produced. Not shown in the figure is an important regulatory step, namely, that IL-10 produced by Th-2 cells inhibits IL-12 production by macrophages and drives the system toward an antibody response and away from a cell-mediated response. *The human cell that produces the IL-4, which induces naïve helper T cells to become Th-2 cells, has not been identified.
One important regulator of the balance between Th-1 cells and Th-2 cells is IL-12, which is produced by macrophages. IL-12 increases the number of Th-1 cells, thereby enhancing host defenses against organisms that are controlled by a delayed hypersensitivity response (Table 58–4). Another important regulator is gamma interferon, which inhibits the production of Th-2 cells. CD4 cells make up about 65% of peripheral T cells and predominate in the thymic medulla, tonsils, and blood.
To mount a protective immune response against a specific microbe requires that the appropriate subpopulation (i.e., either Th-1 or Th-2 cells) play a dominant role in the response. For example, if an individual is infected with M. tuberculosis and Th-2 cells are the major responders, then humoral immunity will be stimulated rather than cell-mediated immunity. Humoral immunity is not protective against M. tuberculosis, and the patient will suffer severe tuberculosis. Similarly, if an individual is infected with Streptococcus pneumoniae and Th-1 cells are the major responders, then humoral immunity will be not be stimulated and the patient will have severe pneumococcal disease. Precisely what component of a microbe activates either Th-1 or Th-2 cells is unknown.
How the appropriate response is stimulated is known for one medically important organism, namely, M. tuberculosis. A lipoprotein of that bacterium interacts with a specific Toll-like receptor on the surface of the macrophage, which induces the production of IL-12 by the macrophage. IL-12 drives the differentiation of naïve helper T cells to form the Th-1 type of helper T cells that are required to mount a cell-mediated (delayed hypersensitivity) response against the organism.
A subset of CD4 cells called Th-17 cells play an important role in mucosal immunity, especially in the mucosa of the gastrointestinal (GI) tract. These cells are characterized by producing IL-17 rather than the typical cytokines produced by Th-1 cells, namely gamma interferon, and Th-2 cells, namely IL-4. IL-17 acts to recruit neutrophils to the site of bacterial infections. One clinical finding related to Th-17 cells is that they are selectively killed by human immunodeficiency virus (HIV). The loss of Th-17 cells results in a high rate of bloodstream infections caused by colonic bacteria, such as Escherichia coli and Klebsiella. IL-17 also contributes to our host defenses against certain fungal infections, such as chronic mucocutaneous candidiasis. The signature cytokines produced by the subsets of CD4-positive helper T cells are described in Table 58–5.
CD8 lymphocytes perform cytotoxic functions (i.e., they kill virus-infected tumor and allograft cells). They kill by either of two mechanisms, namely, the release of perforins, which destroy cell membranes, or the induction of programmed cell death (apoptosis). CD8 cells predominate in human bone marrow and gut lymphoid tissue and constitute about 35% of peripheral T cells.
Activation of T Cells
The activation of helper T cells requires that their TCR recognize a complex on the surface of antigen-presenting cells (APCs) (e.g., macrophages and dendritic cells)1 consisting of both the antigen and a class II MHC protein. The activation of cytotoxic T cells requires that their TCR recognize a complex on the surface of APCs consisting of both the antigen and class I MHC protein. Note that this can occur because APCs have both class I and class II proteins on their surface.
The activation of helper T cells begins with the ingestion of the foreign protein (or microbe) into the APC. Within the cytoplasm of the APC, the foreign protein is cleaved into small peptides that associate with the class II MHC proteins. The complex is transported to the surface of the APC, where the antigen, in association with a class II MHC protein, is presented to the receptor on the CD4-positive helper cell. This plus the action of costimulators (see later) activates the helper T cell.
Note that APCs (e.g., dendritic cells) are typically under an epithelial surface, whereas T cells are primarily in lymph nodes. How do the two cells get together? After the APC ingests the microbe, it produces a receptor for the chemokine CCR7. T cells in the lymph node continuously produce CCR7, and the dendritic cell migrates from the epithelium to the lymph node via the lymphatics by ascending the gradient of CCR7.
The activation of cytotoxic T cells can occur when the APC itself is infected with a virus and viral proteins are synthesized and then presented on the surface in association with class I MHC proteins. Activation of cytotoxic T cells can also occur when the APC ingests pieces of a dying virus-infected cell. Viral antigens from the infected cell are then presented in association with class I MHC proteins, a process called cross-presentation.
Similarly, within a virus-infected cell that is not an APC, the newly synthesized viral peptide associates with class I MHC protein and the complex is transported to the surface, where the viral antigen is presented to the receptor on a CD8-positive cytotoxic cell. Remember the rule of eight: CD4 cells interact with class II (4 × 2 = 8), and CD8 cells interact with class I (8 × 1 = 8).
There are many different alleles within the class I and class II MHC genes; hence, there are many different MHC proteins. These various MHC proteins bind to different peptide fragments. The polymorphism of the MHC genes and the proteins they encode are a means of presenting many different antigens to the TCR. Note that class I and class II MHC proteins can only present peptides; other types of molecules do not bind and therefore cannot be presented. Note also that MHC proteins present peptides derived from self proteins as well as from foreign proteins; therefore, whether an immune response occurs is determined by whether a T cell bearing a receptor specific for that peptide has survived the positive and negative selection processes in the thymus.
Costimulation Is Required to Activate T Cells
Two signals are required to activate T cells. The first signal in the activation process is the interaction of the antigen and the MHC protein with the TCR specific for that antigen (Figure 58–4). Note that when the TCR interacts with the antigen-MHC protein complex, the CD4 protein on the surface of the helper T cell also interacts with the class II MHC protein. In addition to the binding of the CD4 protein with the MHC class II protein, other proteins interact to help stabilize the contact between the T cell and the APC (e.g., lymphocyte function-associated antigen 1 [LFA-1] protein2 on T cells [both CD4-positive and CD8-positive] binds to intracellular adhesion molecule 1 [ICAM-1] protein on APCs).
A second costimulatory signal is also required (i.e., B7 protein on the APC must interact with CD28 protein on the helper T cell) (Figure 58–4). If the costimulatory signal occurs, IL-2 is made by the helper T cell, and it is this step that is crucial to producing a helper T cell capable of performing its regulatory, effector, and memory functions. If, on the other hand, the TCR interacts with its antigen (epitope) and the costimulatory signal does not occur, a state of unresponsiveness called anergy ensues (see Chapter 66). The anergic state is specific for that epitope. Other helper T cells specific for other epitopes are not affected. Production of the costimulatory protein depends on activation of the Toll-like receptor on the APC surface. Foreign antigens, such as bacterial proteins, induce B7 protein, whereas self antigens do not.
FIGURE 58–4 Activation of T cells. Left: An antigen-presenting cell (APC) presents processed antigen in association with a class II major histocompatibility complex (MHC) protein. The antigen is recognized by the T-cell receptor (TCR) specific for that antigen, and the helper T cell is activated to produce interleukin-2 (IL-2). IL-2 binds to its receptor on the helper T cell and further activates it. Note that CD4 protein on the helper T cell binds to the MHC class II protein on the APC, which stabilizes the interaction between the two cells, and that B7 on the APC must interact with CD28 on the helper T cell for full activation of helper T cells to occur. Right: A virus-infected cell presents viral antigen in association with class I MHC protein. The viral antigen is recognized by the TCR specific for that antigen, and in conjunction with IL-2 produced by the helper T cell, the cytotoxic T cell is activated to kill the virus-infected cell. The CD8 protein on the cytotoxic T cell binds to the class I protein on the virus-infected cell, which stabilizes the interaction between the two cells. Note that the class II MHC protein consists of two polypeptides, both of which are encoded by genes in the human leukocyte antigen (HLA) locus. The class I protein, in contrast, consists of one polypeptide encoded by the HLA locus and β2-microglobulin (β2 MG), which is encoded elsewhere.
After the T cell has been activated, a different protein called cytotoxic T lymphocyte antigen-4 (CTLA-4) appears on the T-cell surface and binds to B7 by displacing CD28. The interaction of B7 with CTLA-4 inhibits T-cell activation by blocking IL-2 synthesis (Figure 58–5). This restores the activated T cell to a quiescent state and thereby plays an important role in T-cell homeostasis. Mutant T cells that lack CTLA-4 and therefore cannot be deactivated cause autoimmune reactions. Furthermore, administration of CTLA-4 reduced the rejection of organ transplants in experimental animals.
FIGURE 58–5 Inhibition of activated helper T cells. When the activated helper T cells are no longer needed, a return to a quiescent state occurs when an inhibitory protein called CTL-4 is displayed on the surface of the helper T cell. CTL-4 binds more strongly to B7 than does CD28 and so displaces CD28 from its interaction with B7. This inhibits the synthesis of interleukin-2 (IL-2), and the T cell enters a resting state. Left: Activation of the helper T cells occurs because B7 protein is displayed on the surface of the antigen-presenting cell and interacts with CD28 on the helper T cell. (This is the same process as that depicted on the left side of Figure 58–4.) Right: CTL-4 protein is displayed on the surface of the helper T cell and interacts with B7 on the antigen-presenting cell. As a result, IL-2 is no longer synthesized. MHC, major histocompatibility complex; TCR, T-cell receptor.
The clinical importance of CTLA-4 is dramatically illustrated by the effectiveness of abatacept (Orencia) in rheumatoid arthritis. Abatacept is CTLA-4-IG, a fusion protein composed of CTLA-4 and a fragment of the Fc domain of human IgG. The Fc fragment provides resistance against degradation, resulting in increased plasma levels of CTLA-4 for a longer duration than CTLA-4 alone. The mechanism of action of abatacept is the binding of CTLA-4 to B7, thereby displacing CD-28 from its binding to B7. This results in a reduction of the helper T-cell activity and a reduction in the inflammatory response.
Administration of antibody against CTLA-4 can enhance the immune response against some human cancer cells and cause the cancer to regress. Note that in this instance, the antibody is an inhibitor of an inhibitory molecule (CTLA-4), resulting in an enhancement of the immune response against the cancer cells.
In addition to CTLA-4, there is another inhibitory protein on the surface of T cells called PD-1 (programmed cell death-1). When PD-1 interacts with its ligand (PDL-1) on the surface of APCs, such as dendritic cells and macrophages, the immune response is inhibited. Monoclonal antibodies against PD-1 that enhance the immune response are effective as anticancer drugs in clinical trials.
T Cells Recognize Only Peptides
T cells recognize only polypeptide antigens. Furthermore, they recognize those polypeptides only when they are presented in association with MHC proteins. Helper T cells recognize antigen in association with class II MHC proteins, whereas cytotoxic T cells recognize antigen in association with class I MHC proteins. This is called MHC restriction (i.e., the two types of T cells [CD4 helper and CD8 cytotoxic] are “restricted” because they are able to recognize antigen only when the antigen is presented with the proper class of MHC protein). This restriction is mediated by specific binding sites primarily on the TCR, but also on the CD4 and CD8 proteins that bind to specific regions on the class II and class I MHC proteins, respectively.
Generally speaking, class I MHC proteins present endogenously synthesized antigens (e.g., viral proteins), whereas class II MHC proteins present the antigens of extracellular microorganisms that have been phagocytized (e.g., bacterial proteins). One important consequence of these observations is that killed viral vaccines do not activate the cytotoxic (CD8-positive) T cells, because the virus does not replicate within cells and therefore viral epitopes are not presented in association with class I MHC proteins. Class I and class II proteins are described in more detail in Chapter 62.
This distinction between endogenously synthesized and extracellularly acquired proteins is achieved by processing the proteins in different compartments within the cytoplasm. The endogenously synthesized proteins (e.g., viral proteins) are cleaved by a proteasome, and the peptide fragments associate with a “TAP transporter” that transports the fragment into the rough endoplasmic reticulum, where it associates with the class I MHC protein. The complex of peptide fragment and class I MHC protein then migrates via the Golgi apparatus to the cell surface. In contrast, the extracellularly acquired proteins are cleaved to peptide fragments within an endosome, where the fragment associates with class II MHC proteins. This complex then migrates to the cell surface.
An additional protection that prevents endogenously synthesized proteins from associating with class II MHC proteins is the presence of an “invariant chain” that is attached to the class II MHC proteins when these proteins are outside of the endosome. The invariant chain is degraded by proteases within the endosome, allowing the peptide fragment to attach to the class II MHC proteins only within that compartment.
B cells, on the other hand, can interact directly with antigens via their surface immunoglobulins (IgM and IgD). Antigens do not have to be presented to B cells in association with class II MHC proteins, unlike T cells. Note that B cells can then present the antigen, after internalization and processing, to helper T cells in association with class II MHC proteins located on the surface of the B cells (see the section on B cells, later). Unlike the antigen receptor on T cells, which recognizes only peptides, the antigen receptors on B cells (IgM and IgD) recognize many different types of molecules, such as peptides, polysaccharides, nucleic acids, and small molecules (e.g., drugs such as penicillin).
These differences between T cells and B cells explain the hapten-carrier relationship described in Chapter 57. To stimulate hapten-specific antibody, the hapten must be covalently bound to the carrier protein. The hapten binds to the IgM receptor on the B-cell surface. That IgM is specific for the hapten, not the carrier protein. The hapten-carrier conjugate is internalized and the carrier protein processed into small peptides that are presented in association with class II MHC proteins to a helper T cell bearing a receptor for that peptide. The helper T cell then secretes lymphokines that activate the B cell to produce antibodies to the hapten.
When the antigen–MHC protein complex on the APC interacts with the TCR, a signal is transmitted by the CD3 protein complex through several pathways that eventually lead to a large influx of calcium into the cell. (The details of the signal transduction pathway are beyond the scope of this book, but it is known that stimulation of the TCR activates a series of phosphokinases, which then activate phospholipase C, which cleaves phosphoinositide to produce inositol triphosphate, which opens the calcium channels.) Calcium activates calcineurin, a serine phosphatase. Calcineurin moves to the nucleus and is involved in the activation of the genes for IL-2 and the IL-2 receptor. (Calcineurin function is blocked by cyclosporine, one of the most effective drugs used to prevent rejection of organ transplants [see Chapter 62].)
The end result of this series of events is the activation of the helper T cell to produce various lymphokines (e.g., IL-2), as well as the IL-2 receptor. IL-2, also known as T-cell growth factor, stimulates the helper T cell to multiply into a clone of antigen-specific helper T cells. Most cells of this clone perform effector and regulatory functions, but some become memory cells (see later), which are capable of being rapidly activated upon exposure to antigen at a later time. (Cytotoxic T cells and B cells also form memory cells.) Note that IL-2 stimulates CD8 cytotoxic T cells as well as CD4 helper T cells. Activated CD4-positive T cells also produce another lymphokine called gamma interferon, which increases the expression of class II MHC proteins on APCs. This enhances the ability of APCs to present antigen to T cells and upregulates the immune response. (Gamma interferon also enhances the microbicidal activity of macrophages.)
The process of activating T cells does not function as a simple “on–off” switch. The binding of an epitope to the TCR can result in either full activation, partial activation in which only certain lymphokines are made, or no activation, depending on which of the signal transduction pathways is stimulated by that particular epitope. This important observation may have profound implications for our understanding of how helper T cells shape our response to infectious agents.
There are three genes at the class I locus (A, B, and C) and three genes at the class II locus (DP, DQ, and DR). We inherit one set of class I and one set of class II genes from each parent. Therefore, our cells can express as many as six different class I and six different class II proteins (see Chapter 62). Furthermore, there are multiple alleles at each gene locus. Each of these MHC proteins can present peptides with a different amino acid sequence. This explains, in part, our ability to respond to many different antigens.
Memory T Cells
Memory T (and B) cells, as the name implies, endow our host defenses with the ability to respond rapidly and vigorously for many years after the initial exposure to a microbe or other foreign material. This memory response to a specific antigen is due to several features: (1) many memory cells are produced, so that the secondary response is greater than the primary response, in which very few cells respond; (2) memory cells live for many years or have the capacity to reproduce themselves; (3) memory cells are activated by smaller amounts of antigen and require less costimulation than do naïve, unactivated T cells; and (4) activated memory cells produce greater amounts of interleukins than do naïve T cells when they are first activated.
T-Cell Receptor
The TCR for antigen consists of two polypeptides, alpha and beta,3 which are associated with CD3 proteins.
TCR polypeptides are similar to immunoglobulin heavy chains in that (1) the genes that code for them are formed by rearrangement of multiple regions of DNA (see Chapter 59); (2) there are V (variable), D (diversity), J (joining), and C (constant) segments that rearrange to provide diversity, giving rise to an estimated number of more than 100 million different receptor proteins; (3) the variable regions have hypervariable domains; and (4) the two genes (RAG-1 and RAG-2) that encode the recombinase enzymes that catalyze these gene rearrangements are similar in T cells and B cells.
Note that each T cell has a unique TCR on its surface, which means that hundreds of millions of different T cells exist in each person. Activated T cells, like activated B cells, clonally expand to yield large numbers of cells specific for that antigen.
Although TCRs and immunoglobulins (antibodies) are analogous in that they both interact with antigen in a highly specific manner, the TCR is different in two important ways: (1) it has two chains rather than four, and (2) it recognizes antigen only in conjunction with MHC proteins, whereas immunoglobulins recognize free antigen. Note that the receptor on the surface of B cells (either IgM or IgG) recognizes antigen directly without the need for presentation by MHC proteins. Also TCR proteins are always anchored into the outer membrane of T cells. There is no circulating form as there is with certain antibodies (e.g., monomeric IgM is in the B-cell membrane, but pentameric IgM circulates in the plasma).
Effect of Superantigens on T Cells
Certain proteins, particularly staphylococcal enterotoxins and toxic shock syndrome toxin, act as “superantigens” (Figure 58–6). In contrast to the typical (nonsuper) antigen, which activates one (or a few) helper T cell, superantigens are “super” because they activate a large number of helper T cells. For example, toxic shock syndrome toxin binds directly to class II MHC proteins without internal processing of the toxin. This complex interacts with the variable portion of the beta chain (Vβ) of the TCR of many T cells.4
FIGURE 58–6 Activation of helper T cells by superantigen. Top: The helper T cell is activated by the presentation of processed antigen in association with class II major histocompatibility complex (MHC) protein to the antigen-specific portion of the T-cell receptor. Note that superantigen is not involved and that only one or a small number of helper T cells specific for the antigen are activated. Bottom: The helper T cell is activated by the binding of superantigen to the Vβ portion of the T-cell receptor outside of its antigen-specific site without being processed by the antigen-presenting cell. Because it bypasses the antigen-specific site, superantigen can activate many helper T cells. (Modified and reproduced with permission from Pantaleo G et al. Mechanisms of disease: The immunopathogenesis of human immunodeficiency virus infection. N Engl J Med. 1993;328:327.)
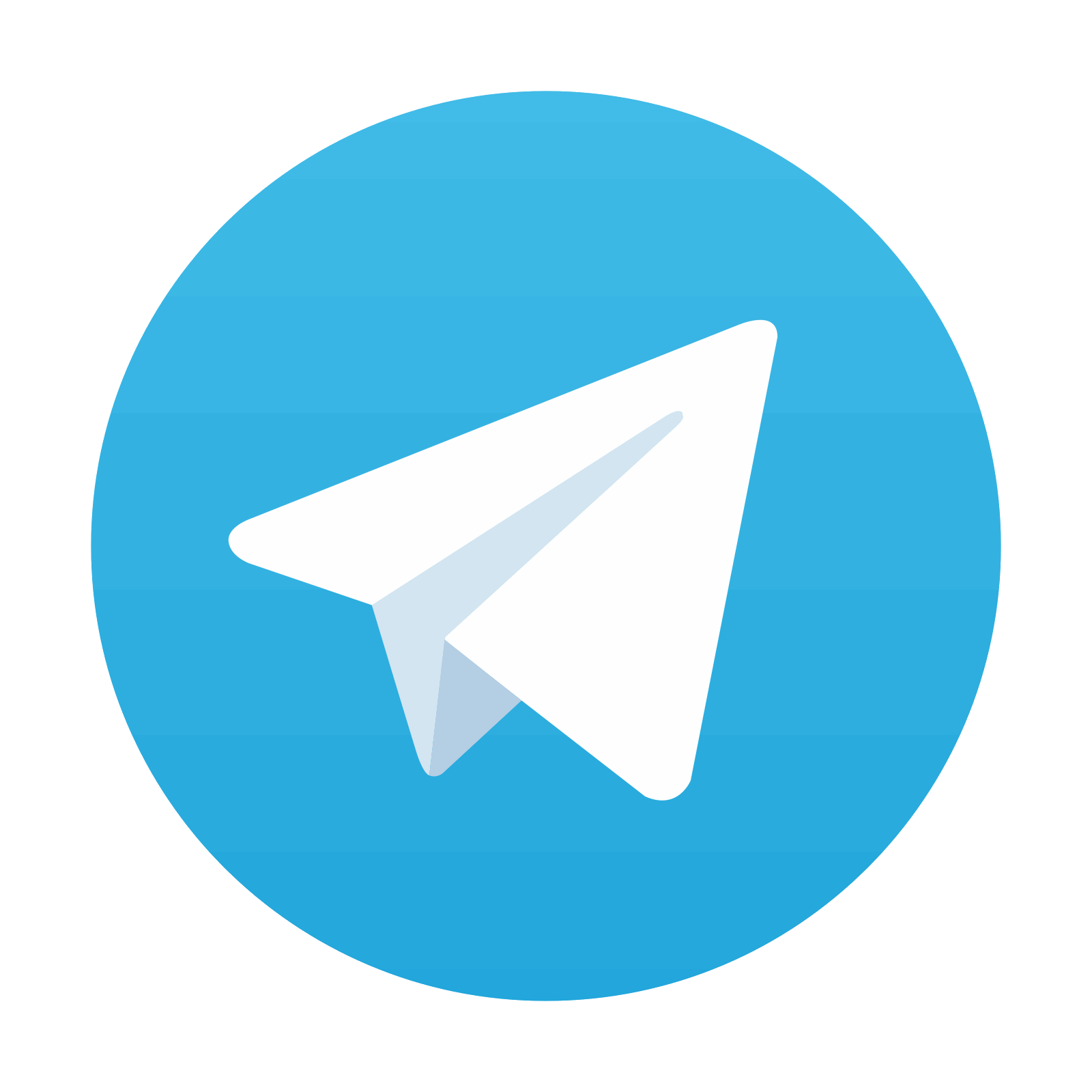
Stay updated, free articles. Join our Telegram channel

Full access? Get Clinical Tree
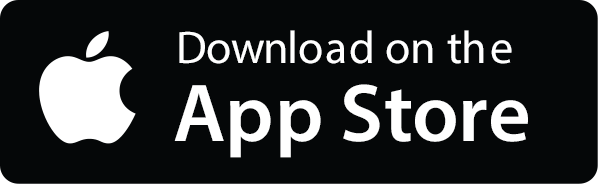
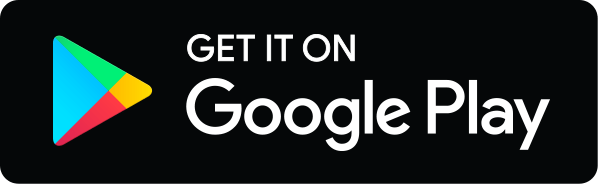