Chapter 4 Cardiovascular Physiology
I. Cardiac Mechanics
A. Cardiac cycle: composed of systole and diastole
1. Systole
• In atrial systole, the atria pump blood into the relaxed ventricles, whereas in ventricular systole, the ventricles pump blood into the blood vessels.
• Function to establish one-way flow of blood in the heart (Fig. 4-1)
1. Atrioventricular valves: mitral and tricuspid valves
• The atrioventricular (AV) valves prevent blood from flowing back into the atria during ventricular systole.
• Reflect valve closure and/or pathologic states
1. S1: closure of AV valves
• Produced by closure of the AV valves in early systole as a result of the rapidly increasing ventricular pressure
• Has mitral (M1) and tricuspid (T1) components that do not vary with the respiratory cycle (i.e., S1 split during inspiration or expiration is not normal)
2. S2: closure of semilunar valves
• Diastolic pressures in the aorta and pulmonary artery exceed the pressures in the relaxing ventricles, causing the semilunar valves to close.
• Has aortic (A2) and pulmonic (P2) components (A2:P2), which vary with the respiratory cycle (Fig. 4-2)
• During inspiration, when intrathoracic pressures decrease, the reduced pulmonary artery pressure decreases the back pressure responsible for pulmonic valve closure, resulting in delayed closure of the pulmonic valve and a “split” S2.
a. Furthermore, despite the increased preload, for unclear reasons the aortic valve closes earlier during inspiration.
3. S3: ventricular gallop
• Caused by a sudden limitation of ventricular expansion (some authors believe it is caused by sudden tensing of the chordae tendineae)
4. S4: atrial gallop
• An S4 is sometimes heard in late diastole and is caused by atrial contraction against a stiffened (noncompliant) ventricle.
D. Ventricular pressure changes during the cardiac cycle (Fig. 4-3)
• During diastole, the ventricles gradually increase in volume, causing ventricular pressures to gradually increase (A).
• The slight “hump” before systole (B) represents atrial contraction in the final “topping off” phase of ventricular filling.
• Pressure continually builds until the ventricular pressure exceeds that of the aorta (left ventricle) or the pulmonary artery (right ventricle) (D).
• After the ventricles have finished contracting, they begin to relax, and intraventricular pressures decrease.
• After closure of the semilunar valves, the ventricles continue to relax, and intraventricular pressures continue to decrease (H).
• Once intraventricular pressures are less than atrial pressures, the AV valves open (I), and the ventricular filling of diastole begins again.
• Note: The normal phonocardiogram in Figure 4-3 parallels the ventricular pressure curve.
a. S1 occurs at the beginning of systole with AV valve closure (C), and S2 occurs at the beginning of diastole with semilunar valve closure (G).
b. If an S3 were present, it would occur in early diastole, because it is caused by rapid ventricular filling.
II. Cardiac Performance
• Cardiac performance is often assessed by measuring the cardiac output, which is the volume of blood pumped out of the heart each minute.
A. Cardiac output
4. CO can also be calculated by measuring whole body oxygen consumption.
B. Stroke volume
2. Ejection fraction
• Ejection fraction (EF) is the percentage of blood in the ventricle at the end of diastole that is pumped into the circulation with each heartbeat.
• SV is determined by three principal factors: preload, contractility, and afterload.
• Preload
b. This load is mainly determined by the volume of blood within the ventricle at the end of diastole (EDV), which in itself is primarily dependent on venous return.
d. Precisely why an increased EDV increases SV remains controversial, but there are two prominent theories.
• The Frank-Starling relationship, also called the length-tension relationship of the heart theory, postulates that the increased ventricular wall tension associated with increased EDV stretches ventricular myocytes and results in a greater overlap of actin and myosin filaments.
• Contractility
a. Contractility is a measure of the forcefulness of contraction at any given preload (i.e., independent of myocardial wall tension at EDV) (Fig. 4-5).
4. Mechanical characterization of contraction
• Wall tension
c. The Laplace equation relates these two forces:
where σ = wall tension, P = intraluminal pressure, r = intraluminal radius, and h = wall thickness.
d. Think of the ventricle as a very thick-walled vessel, and use the Laplace equation to determine that, if the ventricle must generate a greater intraventricular pressure to overcome an afterload, myocardial wall tension will increase.
• Stroke work
a. Stroke work is a measure of the mechanical work performed by the ventricle with each contraction.
b. Stroke work will increase in two scenarios: by increasing stroke volume against a constant afterload or by maintaining a given SV while afterload increases.
c. Stroke work has two main components.
• Pressure-volume work is work used to push the SV into the high-pressure arterial system and is equal to the systemic arterial pressure multiplied by the SV (P × SV).
C. Venous return
1. Effect of venous return on cardiac output by influencing preload
• The rate of venous return is determined by the pressure gradient between the systemic veins and the right atrium.
• Increased venous return increases preload and CO (Fig. 4-7).
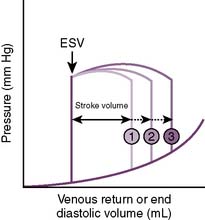
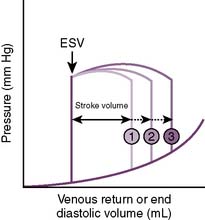
4-7 Relationship of preload to stroke volume. ESV, End systolic volume.
(From Lilly LS: Pathophysiology of Heart Disease, 3rd ed. Philadelphia: Lippincott Williams & Wilkins; 2003, Fig. 9-5A.)
• At increased right atrial pressures (e.g., pulmonary hypertension), venous return is reduced because the pressure gradient driving venous return is reduced.
• When right atrial pressure equals systemic venous pressure, there is no pressure gradient and therefore no flow.
• Contraction of the veins or infusion of volume increases the systemic venous pressure and therefore the driving force for venous return to the right side of the heart, increasing venous return (Fig. 4-8, point B) at a given right atrial pressure.
• Alternatively, loss of blood or dilation of the veins reduces systemic venous pressure and decreases venous return (see Fig. 4-8, point C).
• The situation becomes more complex when the CO curve is superimposed on the venous return curve (Fig. 4-9).
2. Other determinants of venous return
• Skeletal muscle pump
a. During physical exercise, muscle contraction increases the pressure in the veins in the skeletal muscles, which increases the pressure gradient for venous return and thus increases the rate of venous return.
c. The skeletal muscle pump is particularly important in the lower extremities, where the force of gravity has a tendency to cause venous pooling.
D. Ventricular pressure-volume loops
2. There are four phases (Fig. 4-10).
• Phase II: isovolumic contraction
b. The ventricle is contracting, but not shrinking, because sufficient pressure must develop to exceed pressures in the aorta (pulmonary artery for the right ventricle).
• Phase III: ejection period
a. This phase begins as pressures in the left ventricle exceed those in the aorta, causing the aortic valve to open.
E. Atrial pressure changes during the cardiac cycle (Fig. 4-11)
2. A large pressure increase (c wave) is caused by isovolumic ventricular contraction and inward bulging of the AV valves.
3. A rapid reduction in pressure (x descent) is caused by initiation of the ventricular ejection phase; sometimes referred to as the vacuum effect.
F. Pathophysiology of the major valvular diseases
1. Aortic stenosis
• The cross-sectional area of the aortic valve becomes pathologically decreased, causing substantial resistance to ejection of blood through the valve.
• This increase in resistance increases afterload, which decreases the SV and consequently decreases CO.
• Notice how an increased intraventricular pressure must be attained to overcome the significant afterload produced by the stenotic valve.
• The heart expends more energy developing increased pressures; therefore, less energy is available for the ejection phase, so the SV is decreased.
• Development of the pressure necessary to overcome the afterload also takes time, which means that it takes longer for a pulse to appear after closure of the AV valves (S1).
• The combination of reduced SV (which reduces the pulse pressure) and delayed pulse from the increased afterload is responsible for the description of the pulse seen in aortic stenosis: pulsus parvus et tardus (weak and late) (see Fig. 4-12B).
2. Aortic regurgitation (insufficiency)
• In aortic regurgitation, a significant fraction of the blood ejected into the aorta with each heartbeat returns to the left ventricle (Fig. 4-13A). Naturally, this decreases the CO.
• The increased preload that occurs from blood regurgitating back into the ventricle increases the SV (although not necessarily the effective SV), which helps maintain a relatively normal systolic pressure.
3. Mitral stenosis
• In early diastole, the mitral valve opens and provides negligible resistance to blood flow from the left atrium to the left ventricle.
• In mitral stenosis (Fig. 4-14), the mitral valve becomes stenotic owing to abnormal structural changes.
• Resistance to blood flow across the mitral valve increases, and adequate ventricular filling can occur only at pathologically elevated atrial filling pressures.
• As left atrial pressures become elevated, the hydrostatic pressures in the pulmonary veins and capillaries also become elevated, causing net transudation of fluid into the pulmonary interstitium.
• Once the left atrial pressure exceeds 30 to 40 mm Hg, however, the compensatory capacity of the lymphatics is overwhelmed, and fluid begins to accumulate in the lungs.
• This fluid accumulation causes the symptoms of mitral stenosis, such as dyspnea and reduced exercise capacity.
4. Mitral regurgitation (insufficiency)
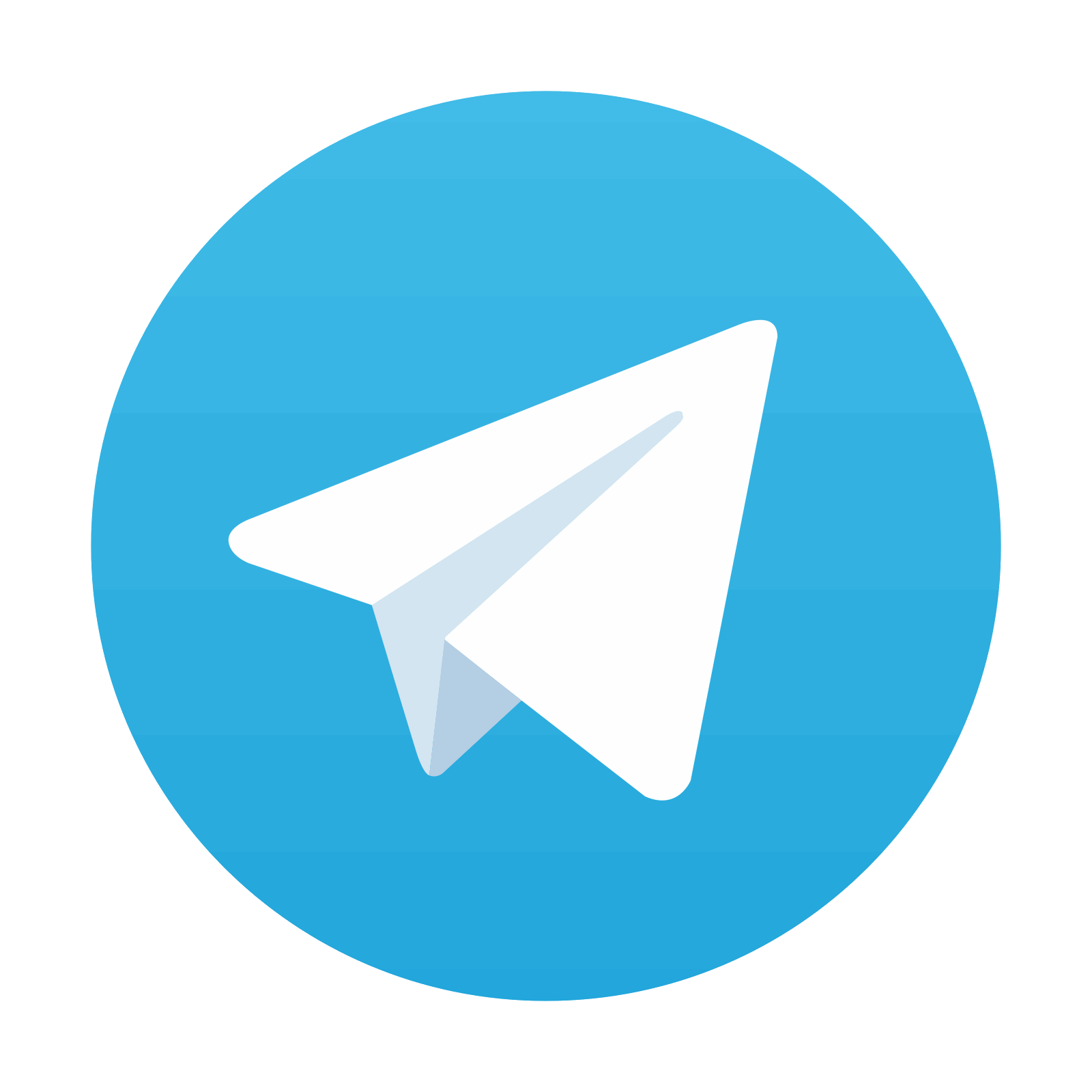
• In early systole, ventricular contraction is isovolumic when both the semilunar and AV valves are closed.
• This allows the entire left ventricular output to move “forward” into the aorta once the aortic valve opens.
• In mitral regurgitation, however, the mitral valve does not form a good “seal” and allows backward flow of blood into the left atrium during early systole.
• Figure 4-15 shows the hemodynamic changes in mitral regurgitation. Note that both the left atrium and left ventricle are enlarged.
• Symptoms of mitral regurgitation therefore may be associated with reduced forward flow CO, elevated left atrial pressures, and/or left ventricular volume overload because of the additional preload imposed on the left ventricle by the addition of the “regurgitated” blood to the normal venous return.
< div class='tao-gold-member'>
< div class='tao-gold-member'>
Only gold members can continue reading. Log In or Register a > to continue
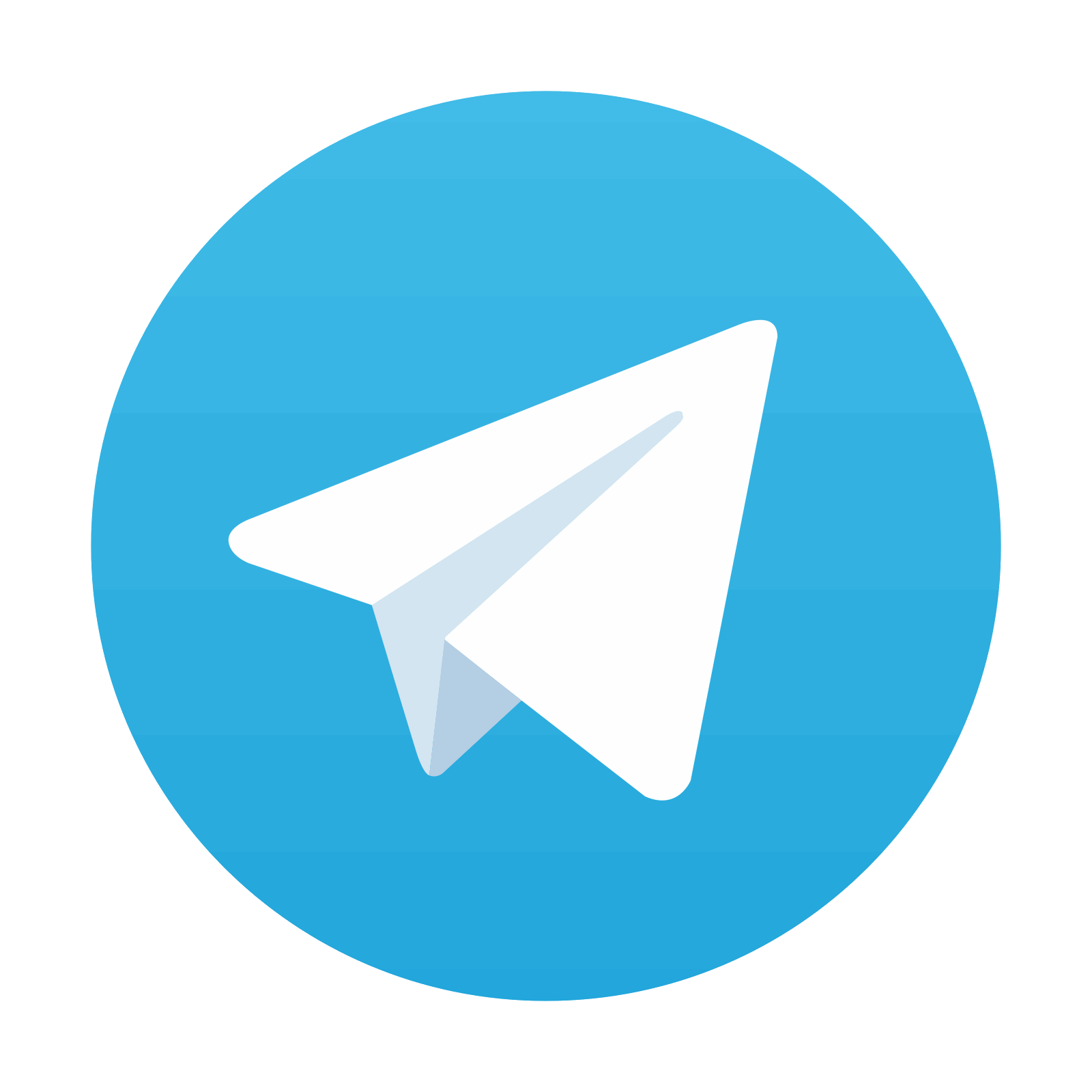
Stay updated, free articles. Join our Telegram channel

Full access? Get Clinical Tree
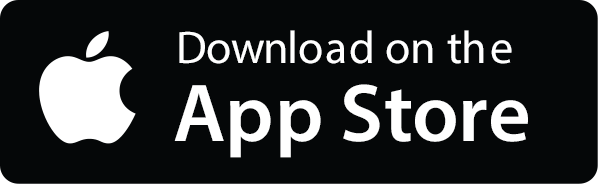
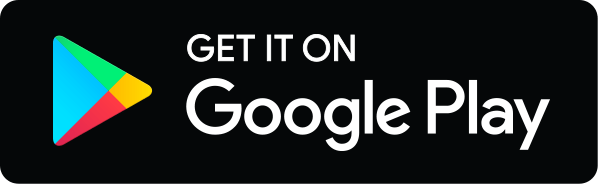