CALCIUM HOMEOSTASIS
Serum calcium level is maintained at a constant level for the optimal excitability of neural and muscular tissue and the coordinated functioning of various organ systems in the human body. Calcium levels are maintained with a narrow range for optimal nerve impulse transmission, muscular contraction, blood coagulation, hormone secretion, and intercellular adhesion. Imbalance of calcium can lead to serious clinical consequences.
In this chapter, the regulation of blood calcium and consequences of alterations in calcium balance are discussed. A preview of organs and organ systems involved is as described in Figure 24.1.
FIGURE 24.1 Calcium homeostasis. Tissue and organs involved in calcium homeostasis (gut, skeleton, and kidneys) and how they relate to blood calcium. Also shown is the means of getting calcium de novo into the system (GI absorption) and elimination of calcium from the system (renal excretion) under normal physiologic conditions.
An adult human body contains 1,000 g of calcium.1 About 99% of this is in the form of hydroxyapatite salt (provides structural rigidity along with collagen matrix), and the remainder (1%) is in the extracellular fluids and regulates various biochemical events. In the blood, calcium exists in ionized (50%), protein-bound (40% to plasma proteins), and complexed (10% to citrate and phosphate) forms. The pH of blood influences binding with proteins (binding increases with high pH and decreases with low pH). The ionized calcium is the only relevant fraction from the cellular function standpoint. The principal organs involved in calcium homeostasis are the skin, liver, small intestine, skeleton, parathyroid glands, and kidneys. Diet is the only source of calcium. The skeleton acts as a mineral repository, releasing calcium into blood on demand, especially during times of poor intake. During prolonged low oral intake or malabsorption, the blood levels are maintained at near-normal levels at the expense of the skeletal stores. The only significant loss of calcium from the body is into urine.
HORMONAL REGULATION OF CALCIUM METABOLISM
Vitamin D and parathyroid hormone (PTH) are the two most important hormones that regulate calcium and phosphate homeostasis. No clear role for calcitonin has been established in healthy humans.
Vitamin D
Vitamin D is a steroid hormone that is synthesized in the skin following exposure to UVB rays from the sun. Following the photobiosynthesis of vitamin D from cholesterol, it undergoes further hydroxylation in the liver and kidneys (Fig. 24.2).2
FIGURE 24.2 Vitamin D synthesis. Tissues involved in the synthesis of vitamin D and the steps that each tissue is responsible for. Also shown are enzymes responsible for the two enzymatically mediated steps (hepatic 25-hydroxylation and renal 1α-hydroxylation). The product of this pathway, 1,25(OH)2D, is responsible for the tissue-specific effects of vitamin D.
The hepatic enzyme, 25-hydroxylase, metabolizes vitamin D3 to 25-hydroxy vitamin D3. Serum 25-hydroxy vitamin D estimation is the best indicator of body stores; therefore, low 25-hydroxy vitamin D levels in the blood imply deficiency. The renal 1α-hydroxylase, under PTH regulation, completes the formation of the active metabolite, 1,25-dihydroxy vitamin D (1,25(OH)2D).
Endogenous synthesis of vitamin D is influenced by the amount of sun exposure, available sunlight (less in northern latitudes), skin covering (clothing and sun block), and age of the individual. Older individuals have less effective photobiosynthesis of vitamin D.
Vitamin D3 (cholecalciferol) is scarcely found in nature. The major dietary sources are internal organs such as liver and sea food. Vitamin D2 (ergocalciferol) is found in edible mushrooms. Commercially available milk is fortified with vitamin D3. Breast-fed infants are at risk for vitamin D deficiency, since breast milk is a poor source of vitamin D. Multivitamins contain 400 units of vitamin D3, about the same amount found in a quart of vitamin D—fortified milk.
Vitamin D is a steroid hormone derived from cholesterol. The same cholesterol biosynthetic pathway that provides the precursors for vitamin D also provides the precursors for other steroid hormones from the adrenal gland and gonads. The steroid receptor supergene family of nuclear receptors carries out its physiologic regulation by directing transcription of specific vitamin D–responsive genes. 1,25(OH)2D is the natural ligand for the vitamin D receptor. Synthetic analogs of vitamin D such as paricalcitol bind to vitamin D receptors with very high affinity and are used to treat secondary hyperparathyroidism due to chronic renal failure.
The 1,25(OH)2D–vitamin D receptor complex binds to the vitamin D response element upstream (5′) of the transcription start site of vitamin D genes and induces gene transcription (Fig. 24.3).
FIGURE 24.3 Vitamin D mechanism of action. DNA binding and interaction with other components of the transcriptional machinery of the vitamin D–vitamin D receptor complex are shown. Note the striking evolutionary similarity between this mechanism and that of other steroid and thyroid hormones. As prime examples, vitamin D inhibits transcription of the PTH gene in parathyroid tissue and stimulates transcription of the calcium transporter in the intestinal brush border epithelium.
Activated vitamin D, 1,25(OH)2D, induces active absorption of calcium in the small bowel cells, which is the dominant mechanism of calcium absorption in humans. Only about 5% to 10% of calcium is absorbed passively.3, 4 The lack of active intestinal absorption of calcium induced by activated vitamin D, 1,25(OH)2D, results in hypocalcemia. Even though activated 1,25(OH)2D also stimulates absorption of phosphate, a larger fraction of phosphate absorption occurs passively and is much less dependent upon normal 1,25(OH)2D levels. This mechanism is illustrated in chronic renal failure patients.
In the bone, 1,25(OH)2D stimulates differentiation of osteoclast precursors to osteoclasts. 1,25(OH)2D also stimulates osteoblasts to influence osteoclasts to mobilize bone calcium. 1,25(OH)2D does not directly affect mature osteoclast function. 1,25(OH)2D plays an important role in the mineralization of bone, and abnormal bone results when vitamin D is deficient (e.g., celiac sprue) or its metabolism is defective (e.g., renal failure).
As noted earlier, 1,25(OH)2D increases blood calcium by augmenting intestinal absorption of calcium. Blood calcium feeds back to parathyroid glands and regulates the synthesis and secretion of PTH. Also, 1,25(OH)2D has direct control over the PTH secretion, and high levels reduce secretion of PTH. Elevated serum phosphate levels reduce 1,25(OH)2D formation (Fig. 24.4).
FIGURE 24.4 Calcium, PTH, and vitamin D feedforward and feedback loops. The endocrine response to shifts in blood calcium (rising or falling) is shown. A concerted hormone response, mediated at the organ level by the organs shown in Figure 24.1, helps restore blood calcium toward normal.
Parathyroid Hormone
PTH is secreted from four parathyroid glands that are located adjacent to the thyroid gland. The parathyroid glands were first described in 1852 by Sir Richard Owen,5 when he performed dissection on an Indian Rhinoceros that had died in London Zoo. Normal parathyroid glands are ovoid or bean shaped and measure approximately 3 mm in size. The superior parathyroid glands are smaller than the inferior pair. The parathyroid glands have an anatomically distinct vascular supply from that of the thyroid gland and are enveloped in a pad of anatomically distinct fibrofatty capsule.6
One human postmortem examination study revealed that four glands are found in 91% of the subjects, three glands in 5%, and five glands in 4%.7 The locations of parathyroid glands vary widely due to the embryonic origination from the third and fourth pharyngeal pouches with eventual migration to the lower neck. The superior parathyroid glands develop in the fourth pharyngeal pouch and migrate caudally along with the ultimobranchial bodies, which give rise to parafollicular (C) cells of the thyroid gland. The superior pair is commonly located along the upper two-thirds of the posterior margin of the thyroid lobe. The superior parathyroid glands are relatively constant in their location along the posterior margin of the thyroid gland. The third bronchial pouches give rise to the inferior pair and the thymus gland, and together, they migrate to the lower neck; in some individuals, the inferior parathyroid glands can migrate into the thorax.
The parathyroid glands have specialized calcium-sensing receptors (CSRs) that respond to rising or falling calcium levels by increasing or decreasing PTH secretion, respectively. The primary targets for the PTH are the bone and kidneys. PTH mobilizes calcium from bone by increasing bone resorption. PTH has three effects on the kidneys:
1. Increase the reabsorption of renal tubular calcium
2. Increase phosphate excretion
3. Enhance 1α-hydroxylation of 25-hydroxy vitamin D
Activated 1,25(OH)2D promotes meal-related intestinal absorption of calcium. In summary, low blood calcium is sensed by the parathyroid CSR, which in turn secretes PTH and sets into motion a cascade of events aimed at restoring normal blood calcium levels.
The multiple actions of PTH described above are mediated via PTH receptor, located on the cell membrane of target tissues. This receptor activates adenylate cyclase and the “second messenger” pathway involving cyclic AMP (cAMP), which modulates protein phosphorylation.
ORGAN SYSTEM REGULATION OF CALCIUM METABOLISM
The three organ systems that regulate calcium metabolism are the gastrointestinal (GI) tract, the kidneys, and the bone.
GI Regulation
Normal intestinal function is required for calcium absorption. Interruptions in intestinal function, as may be seen with short bowel syndromes (following resection), gastric weight loss surgeries, intestinal mucosal disease (celiac disease), genetic defects, and bowel fistula, may affect calcium absorption. Adequate dietary calcium intake, the availability of normal amounts of vitamin D, and metabolism are all necessary for optimal calcium absorption. 1,25(OH)2D controls calcium absorption from the small bowel. Dietary phosphate can bind dietary calcium in the intestinal lumen, forming the insoluble and nonabsorbable precipitate, calcium phosphate. The insolubility of calcium phosphate is the basis for the use of calcium carbonate as a “phosphate binder” in patients with renal failure. Likewise, a diet rich in phosphate will tend to inhibit calcium absorption by the same mechanism.
Role of Kidneys
The kidneys play an essential role in calcium metabolism. This is exemplified in subjects who develop renal failure, leading to markedly disordered calcium and phosphate metabolism.8 Impaired hydroxylation of 25-hydroxy vitamin D to form the active 1,25(OH)2D results in poor calcium absorption from the gut. Diseased kidneys fail to excrete phosphate, leading to elevated serum phosphate levels. Hyperphosphatemia is a powerful stimulus for PTH and fibroblast growth factor 23 (FGF23) secretion. FGF23 is a powerful phosphaturic hormone secreted by the osteocytes that binds to the FGF receptor 1 and coreceptor Klotho in the kidney. FGF23 inhibits 1α-hydroxylation of 25 vitamin D (inactive) to and 1,25(OH)2D (active) but also stimulates 24α-hydroxylation (inactivation) of 1,25(OH)2D. The net result is very low 1,25(OH)2 D levels, leading to hypocalcemia.
Serum PTH levels can be elevated in chronic renal failure patients. Once a critical calcium/phosphate product is reached, precipitation of these minerals occurs within the tissues, leading to devastating consequences.
In physiological state, PTH increases renal tubular reabsorption of calcium, thereby reducing renal loss. Hypercalcemia, whether as a result of autonomous overproduction of PTH, termed primary hyperparathyroidism (PHPT), or from other causes, increases the filtered load of calcium. Even though PTH stimulates tubular reabsorption of calcium, this process is overwhelmed, and the net calcium excretion is increased compared with normal state. This is the fundamental mechanism by which PHPT subjects form calcium kidney stones. See Figure 24.5 for organ system integration of calcium homeostasis.
FIGURE 24.5 Organ system integration of calcium homeostasis.
Bone Physiology
Bone contains about 1 kg of calcium and serves as a repository for calcium, phosphate, and magnesium. Bone turnover or “remodeling” is a regulated and coupled process of simultaneous bone formation and breakdown that occurs to varying degrees throughout the life and at multiple sites in the skeleton. The process of “coupling” ensures that neither resorption nor formation occurs in excess compared to the other, thereby preventing imbalance between formation and loss of bone. Too much resorption results in weaker bones and too much bone formation can obliterate the marrow space. Bone formation is mediated by osteoblasts, and bone breakdown, or resorption, is mediated by osteoclasts (a cell in the monocyte/macrophage lineage). Interestingly, although osteoclasts are required to mobilize calcium from bone, they do not appear to express receptors for either 1,25(OH)2D or PTH. Rather, these hormones act directly on osteoblasts, which in turn produce a complex array of hormones, which regulate the osteoclast activity, either activating or deactivating them. When the net rates of osteoblast-mediated bone formation and osteoclast-mediated bone resorption are mismatched, or uncoupled, such that resorption exceeds formation, bone mass will ultimately decrease. Decreased bone mass translates clinically into an increased risk of fracture, as seen in osteoporosis. As bones turn over, several proteins are released into the blood and eventually excreted in the urine, collectively called as bone turnover markers.9 Bone turnover markers are useful in the monitoring of clinical response to osteoporosis therapies and for predicting fracture risks.
Resorption markers include the following:
- Hydroxyproline
- N-telopeptides
- C telopeptides
- Cross-links
- Tartrate-resistant acid phosphatase (TRAP)
Formation markers include the following:
- Bone-specific alkaline phosphatase (BSAP)
- Osteocalcin
- Procollagen N-terminal extension peptides
There are two main types of bones found in the skeleton: cortical bone and trabecular bone. Cortical bone is the predominant bone type found in the shaft of long bones, such as the femur. Cortical bone is very strong, yet light in weight and is therefore well suited for the mechanical needs of the extremities. Trabecular bone is the primary bone type found in the axial skeleton, such as the vertebrae. It consists of numerous cross-hair or honeycomb-type connections called trabeculae. These interconnected trabeculae give this type of bone considerable strength for weight bearing. Although the femur is primarily cortical bone and the vertebrae is primarily trabecular bone, sites such as the femoral trochanter and proximal humerus are a mixture of both cortical and trabecular bones. The ends of long bones contain more trabecular bone. The shafts of long bones are predominantly cortical. Certain disease states induce preferential bone loss in specific bone types. Cortical bone is preferentially lost in PHPT, and trabecular bone is mostly lost in postmenopausal osteoporosis. In most conditions, bone loss is diffuse.
In summary, normal calcium homeostasis is achieved by a complex and regulated interplay between vitamin D, PTH, and the target organs. Defects in the function and formation of hormones or organ system disorders can induce disease states, which will be reviewed in the rest of this chapter.
HYPERCALCEMIA
Hypercalcemia results when blood calcium levels in a subject are above the expected normal range of a healthy population. Patients with low serum albumin would be expected to have low total calcium and normal ionized calcium; the opposite is true for patients with high serum albumin. Ionized calcium best correlates with the biological activity of calcium, as well as with symptoms of hypercalcemia or hypocalcemia, and thus, the direct measurement of ionized calcium may often be more valuable clinically. The binding of calcium to proteins should be taken into consideration during assessment of blood calcium levels.
The duration and the level of calcium elevation determine the severity of symptoms and the degree of end-organ damage. Some common symptoms of acute severe hypercalcemia include lethargy, stupor, and coma. Patients with chronic mild hypercalcemia are symptom free. Moderate calcium elevation is associated with intellectual weariness, personality changes, nausea, anorexia, polyuria, kidney stones, hypertension, and electrocardiogram (ECG) changes. In the outpatients setting, PHPT is the most common cause for hypercalcemia.
Cancer-mediated hypercalcemia often presents with profound central nervous system (CNS) symptoms due to rapid and significant calcium elevation at the time of diagnosis.
Causes of Hypercalcemia
1. Disorders of parathyroid glands and CSRs:
- PHPT (autonomous overproduction of PTH): adenoma (>80%), hyperplasia (5% to 10%), carcinoma (<1%), and familial syndromes (MEN1, MEN2a, and PHPT–jaw tumor syndrome).
- Secondary and tertiary hyperparathyroidism: in chronic renal failure patients, the sustained hypocalcemic–hyperphosphatemic stimuli result in parathyroid hyperplasia, leading to sustained and autonomous overproduction of PTH with ensuing hypercalcemia.
- CSR abnormalities (extracellular calcium levels are sensed aberrantly resulting in hypercalcemia): familial hypocalciuric hypercalcemia (FHH) (inactivating mutation of CSR), calcium receptor antibodies, and lithium carbonate.
- Other endocrine disorders: adrenal insufficiency, hyperthyroidism, pheochromocytomas, and vipoma.
2. Cancer-mediated hypercalcemia (paraneoplastic hypercalcemia):
- PTHrP production by tumors: Upper GI adenocarcinomas, squamous cell carcinomas of head and neck and lung, renal cell carcinoma, ovarian carcinoma, HTLV-1 lymphoma, adrenal cancer, carcinoid tumor, islet cell cancer, and pheochromocytoma. Prostate and colonic cancers rarely produce PTHrP.
- 1,25 Hydroxylation of 25-hydroxy vitamin D: Various cell types of lymphomas.
- Cytokine production by tumor cells leads to activation of bone resorption (myeloma and diffuse bone metastasis): Cytokines such as macrophage inflammatory protein-1 alpha, tumor necrosis factor alpha, prostaglandins, and IL-6, which activate osteoclasts through receptor activator of nuclear factor kappa B (RANK).
3. Granulomatous diseases: Sarcoidosis, tuberculosis, leprosy, fungal infections, Crohn disease (granulomas express the hydroxylation enzyme that is required to convert 25-hydroxy vitamin D to active 1,25(OH)2D).
4. Medications: Hydrochlorothiazide (HCTZ), lithium, vitamin A and/or D toxicity, oral calcium excess (milk alkali syndrome [MAS]), tamoxifen, and aminophylline toxicity.
5. Miscellaneous: William’s syndrome and chronic immobilization.
The signs and symptoms of hypercalcemia are highly variable and depend upon the rapidity of onset and severity of hypercalcemia. Clinically, the signs and symptoms also vary from patient to patient and comorbid conditions may also influence the development of symptoms. The signs and symptoms are best described by organ systems:
CNS: Impairment of CNS function, including lethargy, decreased alertness, depression, confusion, forgetfulness, obtundation, and coma.
GI: Patients may experience anorexia, constipation, peptic ulcers, and nausea and vomiting.
Renal: Hypercalciuria leading to kidney stone formation and calcification of renal tubules resulting in nephrogenic diabetes insipidus.
Skeletal: Increased bone resorption and demineralization leading to fractures.
Cardiovascular: Hypercalcemia may cause or exacerbate hypertension. The following ECG changes are seen in hypercalcemia: Increased PR interval, wide QRS complex, and short QT syndrome.
Primary Hyperparathyroidism
PHPT is the most common cause of hypercalcemia, with an incidence of 1 in 500 to 1,000 in the general population. Women are affected threefold more commonly than men. The hallmark of this disorder is the autonomous overproduction of PTH, most commonly by a single adenoma in one of the parathyroid glands (>80%), less commonly by multiple gland hyperplasia (~5% to 10%), and very rarely by parathyroid cancer. Parathyroid carcinoma occurs more commonly in a familial syndrome called the hyperparathyroidism–jaw tumor syndrome. PHPT is also encountered in multiple endocrine neoplasia types 1 and 2 (MEN1 and MEN2a). The apparent increase in the incidence of PHPT was traced to the introduction and wide availability of multichannel analyzers in the 1970s. The incidence of PHPT increased from 7.8 cases per 100,000 to 51 cases per 100,000 in Olmsted County, Minnesota, United States, following the introduction of routine calcium testing.10 Fuller Albright’s description of the disease in the 1930s was notable for advanced bone and end-stage kidney disease at the time of diagnosis.11 This presentation is very uncommon. The fortuitous detection of a large proportion of the prevalent subclinical PHPT subjects, along with early intervention and treatment, has changed the clinical presentation of PHPT. In countries where multichannel analyzers are not available, the disease presentation is severe and with catastrophic renal and skeletal consequences, similar to how it was in the United States in the early 1900s. The dominant clinical phenotype of PHPT in the United States is asymptomatic hypercalcemia, invariably identified fortuitously in patients undergoing yearly physical examination. The apparent increase in the incidence of PHPT was correlated to the wide availability and use of multichannel testing since the 1970s.12 In the Kaiser Permanente study, involving 3.5 million people, the incidence of PHPT varied from 34 to 124 per 100,000 person years. The incidence increases with advancing age and was highest and Blacks followed by White’s with rates for Asians and Hispanics lowest among the ethnicities. Women are affected threefold more frequently than men.13 Along with the increase in the diagnosis of patients with PHPT, the surgical case volume increased an astounding 177% in a state-wide health planning study from California between 1999 and 2008.14
The diagnosis of PHPT is made by biochemical testing, and elevated serum calcium (or ionized calcium) level with inappropriately normal or elevated PTH level confirms the diagnosis. The biochemical differential diagnosis of hypercalcemia is listed in Table 24.1.
TABLE 24.1 Biochemical Differential Diagnosis of Hypercalcemia
PTH, parathyroid hormone; PTHrP, parathyroid hormone–related protein; PHPT, primary hyperparathyroidism; CRF, chronic renal failure; HPT, hyperparathyroidism; FHH, familial hypocalciuric hypercalcemia; milk alk Vit D, milk alkali vitamin D.
PTH Assays
PTH is a polypeptide hormone with C-terminal and amino (N)-terminal ends composed of 84 amino acid residues. PTH 1 to 84 refer to the entire hormone sequence. n-Truncated PTH (7 to 84 PTH) are deficient in the first few residues of the n-terminal end and hence biologically inactive. The integrity of 1 to 11 amino acid residues in the N-terminal end of PTH is required for the biologic activity of PTH. PTH assays use two antibodies that bind to specific sites (one on each of the C- and N-terminal ends), which enable precise detection of intact PTH. A few commonly available assays include the following:
- Intact PTH: measures 7 to 84 PTH and 1 to 84 PTH.
- Bioactive PTH: measures 1 to 84 PTH.
- CAP assay: cAMP inducible PTH. This assay detects biologically active PTH by its ability to induce formation of cAMP.
- PRHrP assays and PTH assays are exclusive to each other, by design. There is no cross-reactivity. Therefore, PTH levels are low in subjects with cancers producing PTHrP.
- PTH can also be estimated in needle biopsy specimens obtained from parathyroid tumors. Hook effect has not been observed despite massive elevation of PTH levels in these specimens obtained by direct puncture of enlarged parathyroid glands.15
CASE STUDY 24.1
A 40-year-old woman presents to her physician complaining of marked left flank pain that began the previous night. She reports that the pain is worse than that of giving birth. She also reports blood in her urine earlier on the day she came to see her doctor. She has felt more fatigued and as if her concentration has not been as good as normal for the last year or so, and she feels more forgetful. She has no significant past medical history. She is taking no medications. Family history contributes no pertinent information. On physical examination, she appears to be in extreme pain. There is marked tenderness on very gentle percussion over the left costovertebral angle. Labs are drawn and are notable for calcium 11.2 mg/dL (normal, 8.5 to 10.2 mg/dL), albumin 3.8 g/dL (normal, 3.5 to 4.8 g/dL), and intact PTH 162 pg/mL (normal, 11 to 54 pg/mL). Renal function is normal (BUN 25 mg/dL and creatinine 0.9 mg/dL). Urine analysis is notable for blood and greater than 50 red blood cells per high-power field. This prompts a 24-hour urine collection, which reveals calcium elevated at 483 mg per 24 hour (normal, 100 to 250 mg per 24 hour).
questions
1. Which laboratory results are abnormal?
2. What is the presumptive diagnosis for this patient? The differential diagnosis?
3. What treatment is indicated for this disease?
Biochemical Findings in PHPT
1. Hypercalcemia
2. Hypophosphatemia: PTH induces phosphaturia.
3. Elevated PTH relative to serum calcium (inappropriately normal PTH in the face of hypercalcemia).
4. Low-normal 25-OH-D and high normal or elevated (30%) 1,25(OH)2D due to PTH-enhancing renal hydroxylation.
5. Urinary calcium excretion is elevated.
6. Metabolic hyperchloremic acidosis (chloride is exchanged for phosphate).
7. Elevated serum alkaline phosphatase in severe disease.
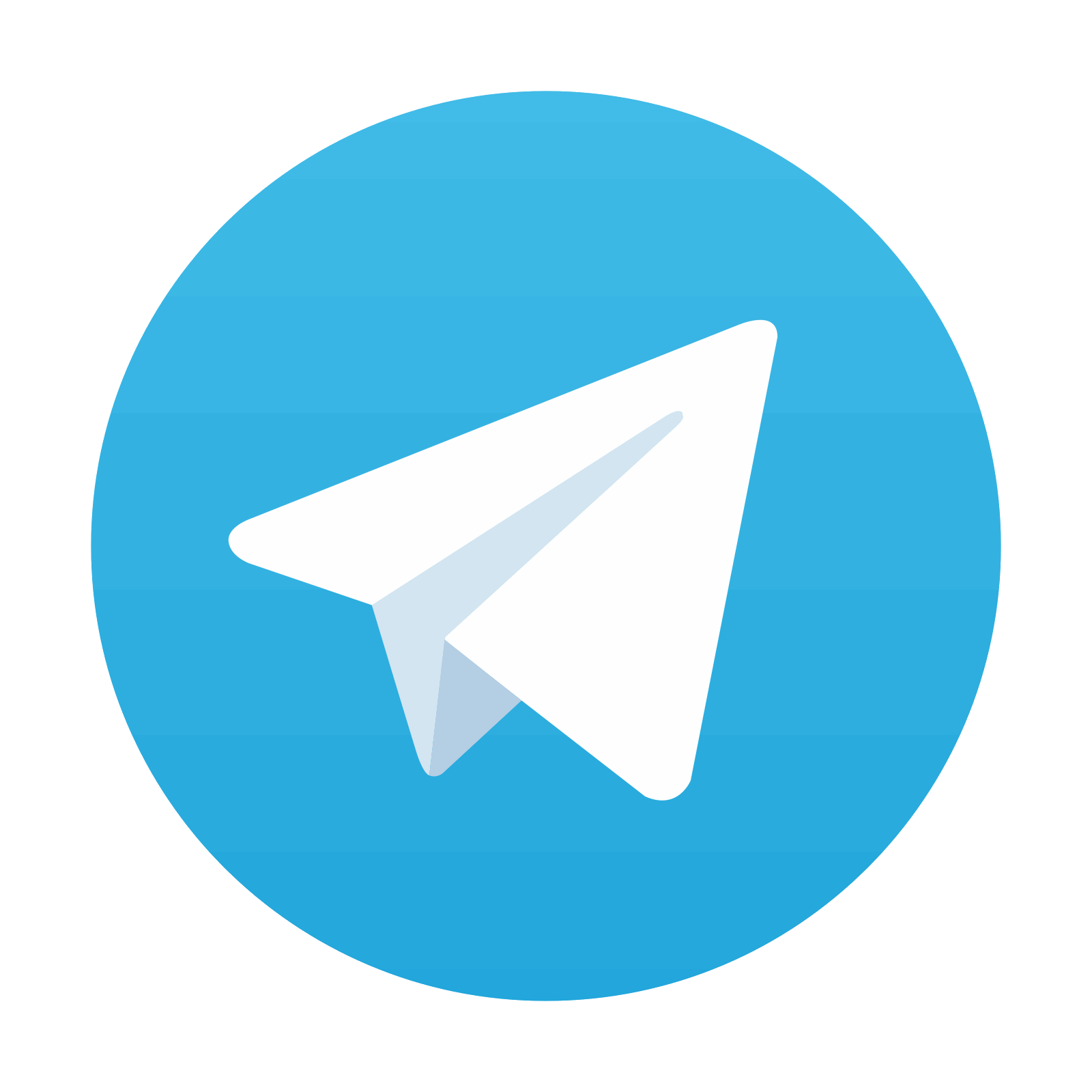
Stay updated, free articles. Join our Telegram channel

Full access? Get Clinical Tree
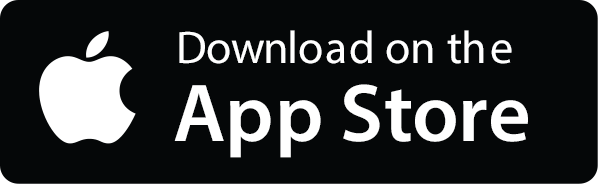
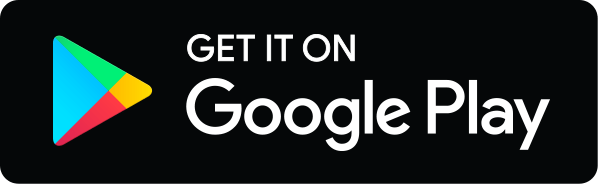