Bone Marrow Structure, Morphology, and Hematopoiesis
Thuy-Lieu Thi Vo
Diane C. Farhi
BONE MARROW STRUCTURE
Bone marrow is a red, soft, semisolid, gelatinous, mesenchymal-derived tissue occupying the medullary cavities of the axial skeleton. It is composed of hematopoietic cells and bone marrow stroma. The medullary cavity is protected by subcortical and cortical bone, which in turn is covered by periosteum (Fig. 1.1). It has an interlaced, delicate network of thin, bony trabeculae. The inner surface of the cortical bone and the outer surface of the bony trabeculae are lined by a single layer of thin, flat, elongated endosteal cells.
Bone marrow specimens from very young children show active remodeling with islands of cartilage, and prominent osteoblasts and osteoclasts. With age, the cartilage converts to bone and osteoblastic and osteoclastic activities become less recognizable. In young to middle-aged adults, osteoblasts become flattened cells and osteoclasts are rarely seen. In older adults, very little bone remodeling is present in normal bone (2,3).
The bone marrow is supported by the nutrient artery and the periosteal capillary network. The nutrient artery penetrates the bony shaft and branches into the medullary cavity. It consists of ascending and descending branches, arterioles, and smaller branching vessels (Fig. 1.2). These smaller branches of the nutrient artery further divide and ultimately form a sinusoidal network composed of ectatic, widely patent, and thin-walled channels at the capillary-venous sinus junctions.
The periosteal capillary network connects with the sinuses at the bone marrow junction through the Haversian canals. The smaller venous sinuses drain into a large, centrally located sinus, which connects with other large sinuses to form the comitant vein. The comitant vein and the nutrient artery travel through the bone marrow together in the same vascular canal.
The hematopoietic cells are closely associated with the thin-walled venous sinuses (4, 5, 6, 7). The venous sinuses are the most prominent vascular space in the bone marrow. They are lined inside by the endothelial cells and supported outside by the adventitial, fibroblastic-like stromal cells (4,6). The sinusoids are the main routes of exit for the hematopoietic cells from bone marrow. After maturation, hematopoietic cells migrate into the sinusoidal space to enter the blood circulation. The sinusoids usually appear histologically empty, but sometimes contain actively dividing hematopoietic cells and megakaryocytes.
Stimulation of erythropoietic or granulopoietic activity leads to increased blood flow to bone marrow. True capillaries are difficult to identify in the bone marrow. The small arterioles appear thickened and more numerous in specimens from patients with chronic hypertension. Bone marrow lacks lymphatic channels (6,8, 9, 10, 11).
The sensory and autonomic nerve fibers innervate the bone marrow and they accompany the blood vessels. Little is known of the relationship between the nervous system and hematopoiesis (Fig. 1.2) (6,12,13).
BONE MARROW MORPHOLOGY
In a healthy individual, the hematopoietic cells and the adipocytes occupy the majority of the bone marrow space, whereas the stromal cells and extracellular matrix claim a very small space. The proportion of hematopoietic cells in comparison to the bone marrow fatty tissue is conventionally referred to as bone marrow cellularity. It is defined as the percentage of the bone marrow volume occupied by hematopoietic cells. The value varies significantly with the age of the individual as well as the location of the bone marrow. The bone marrow cellularity of the iliac crest in a middle-aged, healthy person is about 50%. Younger individuals show more than 50% bone marrow cellularity, and older individuals show less than 50%. The proportion of fatty tissue in the marrow increases with age. This increase is due to increase in the volume and the number of adipocytes (Fig. 1.3). Bone marrow cellularity is higher in the vertebrae and lower in the ribs than in the iliac crest and sternum (14, 15, 16).
In normal bone marrow, there is an organized pattern to the hematopoietic cells. The marrow is hypocellular to acellular in the subcortical region. The immature myeloid precursors are found adjacent to the bony trabeculae, away from the sinusoids. The maturing myeloid cells are in the center of the bone marrow cavity and, when they are mature and needed, pass directly through the cytoplasm of endothelial cells into the sinusoids and enter the circulation.
Megakaryocytes are located adjacent to the sinusoidal wall and they extend their cytoplasmic processes through the cytoplasm of endothelial cells and into the sinusoidal lumen and shed platelets directly into the circulation.
The erythroid precursors present as small, nonparatrabecular clusters that are located near the sinusoids and are composed of cells in varying stages of maturation. Some of these clusters have a centrally located histiocyte. Mature erythrocytes enter the sinusoids by passing directly through the cytoplasm of the endothelial cells. Some of these clusters may remain intact on the smears. Alteration to the cellularity and cellular arrangement of the bone marrow are seen with serious infections or clonal hematopoietic disorders (17,18).
HEMATOPOIESIS
General Features of Hematopoiesis
All hematopoietic cells are derived from pluripotent hematopoietic stem cells. The common pluripotent stem cells that are capable of self-renewal and differentiation give rise to multipotential hematopoietic stem cells and multipotential mesenchymal stem cells. In normal bone marrow, pluripotent hematopoietic stem cells are rare and comprise approximately 0.05% of the total hematopoietic cell mass; they are found among the progenitor cells and more mature, recognizable hematopoietic cells. Multipotential hematopoietic stem cells give rise to progenitor multilineage myeloid stem cells and progenitor lymphoid stem cells. Lymphoid stem cells differentiate into T, B, and natural killer cells and plasma cells. Progenitor myeloid stem cell differentiation includes neutrophils, eosinophils, basophils, monocytes and macrophages, mast cells, erythroid cells, and megakaryocytes. This differentiation is achieved by progressive loss of proliferative activity and by gradual acquisition of lineage-specific characteristics.
Development of Hematopoietic Tissue
Embryonic hematopoiesis begins in the yolk sac as blood islands when the mesoderm layer is formed at the end of the third week of gestation, and declines to an insignificant level by the end of the first trimester. During this stage the hematopoietic stem cells derived from the mesoderm consist
mainly of multipotential stem cells and primitive erythroblasts. The latter are nucleated forms and synthesize embryonic hemoglobins, Hb Portland, and Hb Gower I and II.
mainly of multipotential stem cells and primitive erythroblasts. The latter are nucleated forms and synthesize embryonic hemoglobins, Hb Portland, and Hb Gower I and II.
![]() Figure 1.3 Bone marrow core: normal cellularity. (A) 19-year-old, (B) 32-year-old, (C) 60-year-old, (D) 78-year-old. |
By the sixth week of gestation, hematopoiesis develops in the embryonic liver, which becomes the most prominent source of hematopoiesis by the end of first trimester. In early hepatic hematopoiesis, the most prominent cells are hematopoietic stem cells and erythroid cells. Megakaryocytes are seen by the 12th week and mature neutrophils are present after the 16th week of fetal development. Hepatic hematopoietic activity reaches its maximum level around the third gestational month and gradually declines from the seventh month until birth. Hepatic erythrocytosis produces nonnucleated erythrocytes that are larger than adult red blood cells.
In the bone marrow, hemaopoiesis begins around the end of the 16th week of gestation and, by the 26th week of gestation, the marrow is the primary site of hematopoiesis. Other sources of blood cell formation during fetal life include the spleen during the second trimester, thymus, and lymph nodes. Although the latter two organs are primarily involved in lymphocyte production. The bone marrow is the most prominent hematopoietic organ at birth and remains so throughout life. In young children hematopoiesis occurs in most of the bone cavities, including the distal portion of long bones. In adults, the process is confined to the flat bones (pelvis, skull, ribs, sacrum, vertebrae, scapulae, clavicle) and proximal parts of the long bones (femur and humerus) (29, 30, 31, 32, 33, 34, 35, 36, 37).
The signals for the shift of hematopoiesis from one organ to another during fetal life are not fully understood. It is not known whether this shift is due to migration of the hematopoietic cells from one organ to another or is due to activation and differentiation of primitive multipotent mesenchymal cells already present in these organs.
Regulation
Calculations based on the blood volume and the half-life of each type of blood cell in the circulation indicate that each day an adult produces approximately 200 billion erythrocytes, 100 billion leucocytes, and 100 billion platelets. These
rates can increase many times when the demand for blood cells increases (38,39).
rates can increase many times when the demand for blood cells increases (38,39).
Maturation of the hematopoietic cells is a complex, but orderly process that takes place in the bone marrow microenvironment and involves cell-to-cell interaction, specific growth hormones, and various proteins called cytokines. This results in proliferation, differentiation, activation, and homing of the hematopoietic cells (36,38,40, 41, 42, 43, 44, 45).
Cytokines are a large family of proteoglycans that are produced mainly by stromal cells. They bind to receptor molecules to exert either stimulatory or inhibitory influence on the primitive stem cells, the lineage-committed cells, and the mature cells. Cytokines may act locally at the site of their production or circulate in the blood, and they usually affect more than one lineage (46, 47, 48, 49, 50, 51, 52, 53, 54, 55, 56, 57, 58, 59, 60, 61, 62, 63, 64).
In addition to the cytokines, adhesion molecules are also essential for the regulation of hematopoiesis. The important adhesion molecules include adhesion molecules of the immunoglobulin supergene family, integrins, and selectin. These adhesion molecules mediate and facilitate cell-to-cell interaction and the interaction of hematopoietic cells with the extracellular matrix. They influence the induction, differentiation, and function of hematopoietic cells. Adhesion molecules also regulate the retention and release of hematopoietic cells in the bone marrow (65, 66, 67).
Erythropoietin
Erythropoietin is encoded by a gene located on chromosome 7; it is produced predominantly in the liver during fetal gestation and by the juxtatubular interstitial cells of the renal cortex after birth. The blood levels of erythropoietin are inversely related to the levels of tissue oxygenation. Normally the level is 20 mU per milliliter; however, it can increase up to 20,000 mU per milliliter in response to anemia or arterial hypoxemia. It promotes the proliferation of erythroid progenitor cells by reducing the level of cell-cycle inhibitors and augmenting transcription of cyclins, and supports their survival by increasing the antiapoptosis protein BCLX. Responses to exogenous erythropoietin can be seen readily in the peripheral blood with a rise in the reticulocyte counts due to a large increase in red cell production and the premature exit of erythrocytes from the bone marrow.
Granulocyte-Colony-Stimulating Factor (G-CSF)
G-CSF is a 19.6-kD glycoprotein encoded by a gene located on chromosome 17. It is produced by endothelial cells, fibroblasts, and macrophages. It supports the survival of and stimulates the proliferation of neutrophil progenitors and promotes their differentiation into mature neutrophils. In addition, it causes premature release of neutrophils from the bone marrow and enhances neutrophil phagocytic capacity, generation of superoxide anions, and bacterial killing. The administration of G-CSF causes toxic granulation of neutrophils and a release of immature myeloid cells (left-shift) in the peripheral blood. It also mobilizes the hematopoietic stem cells out of the bone marrow by activating the neutrophils to release metalloproteases.
Thrombopoietin
Thrombopoietin is produced mainly in the liver. The kidney and skeletal muscles also produce a small amount of the hormone. It is the primary regulator of megakaryopoiesis. It supports the survival and proliferation of megakaryocyte progenitors by mechanisms such as increased expression of the cell-cycle regulator cyclin D and the antiapoptosis molecule BCLX, and suppression of the cell-cycle inhibitor p27Kip. Thrombopoietin is also required for the survival and expansion of hematopoietic stem cells. The effects of thrombopoietin on stem cells are mediated by enhanced expression or nuclear localization of several transcription factors (signal transducers and activators of transcription, homeobox B4, and homeobox A9) and by autocrine production of vascular endothelial growth factor (80, 81, 82).
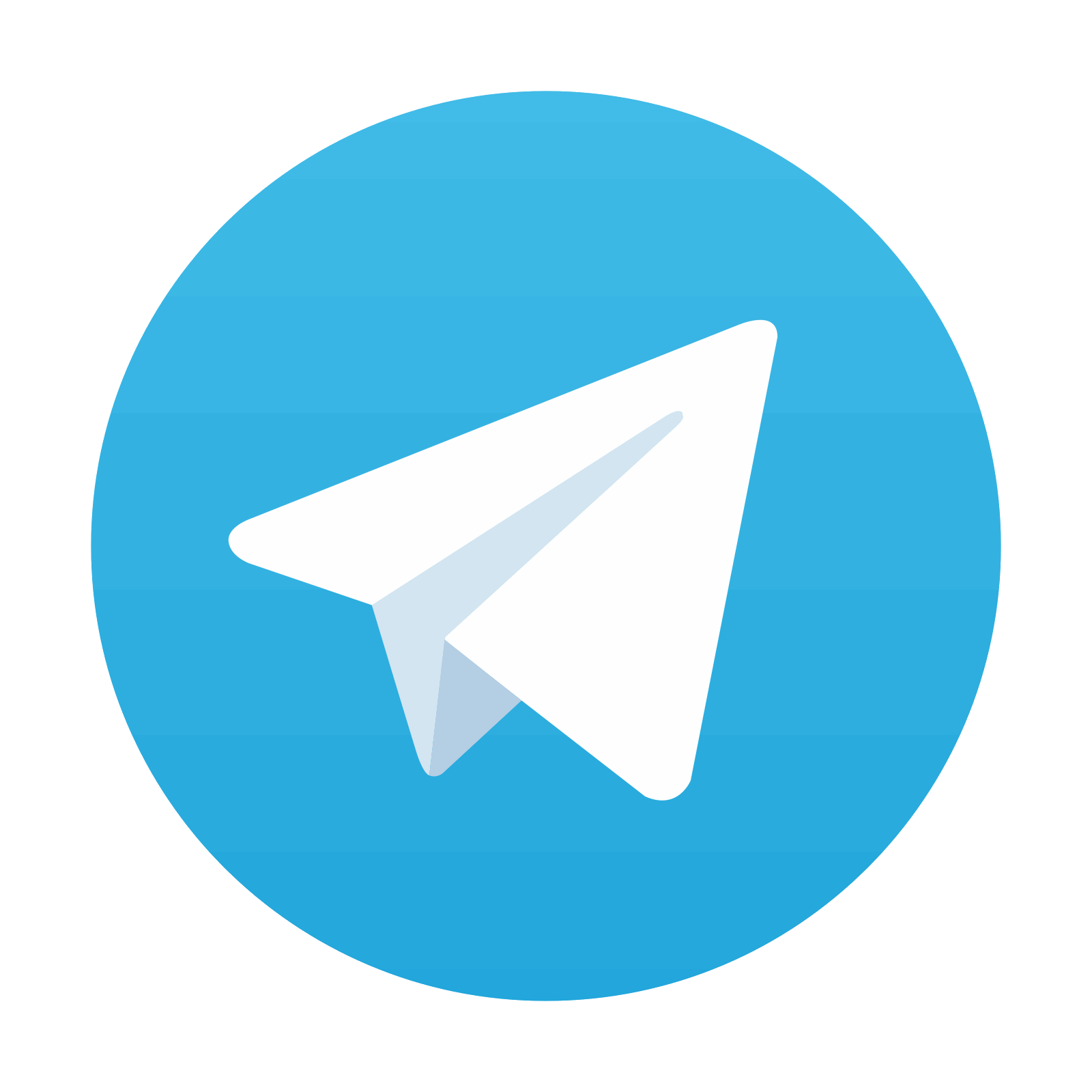
Stay updated, free articles. Join our Telegram channel

Full access? Get Clinical Tree
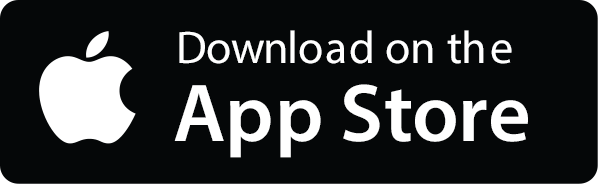
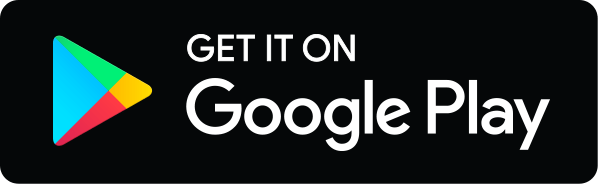
