The blood is the body’s main transport system. Although the transport and delivery of oxygen to the cells of the tissues is carried out by specialized cells, other vital components such as nutrients, metabolites, electrolytes, and hormones are all carried in the noncellular fraction of the blood, the plasma. Some components, such as glucose, are dissolved in the plasma; others, for example, lipids and steroid hormones, are bound to carrier proteins for transport. The osmotic pressure exerted by the plasma proteins regulates the distribution of water between the blood and the tissues. Plasma proteins in conjunction with platelets maintain the integrity of the circulatory system through the process of clotting.
Blood normally circulates through endothelium-lined blood vessels. When a blood vessel is severed, a blood clot (called a thrombus, which is formed by the process of thrombosis) forms as part of hemostasis, the physiological response that stops bleeding. Blood clots also form to repair damage to the endothelial lining, in which components of the subendothelial layer become accessible to plasma proteins.
In hemostasis and thrombosis, a primary hemostatic plug, consisting of aggregated platelets and a fibrin clot, forms at the site of injury. Platelets are attached to the subendothelial layer of the vessel principally through the protein von Willebrand factor and are activated to bind fibrinogen. Fibrinogen binds the platelets to each other to form a platelet aggregate. The platelet aggregate is rapidly covered with layers of fibrin, which are formed from fibrinogen by the proteolytic enzyme thrombin.
Injury to the endothelium and exposure of tissue factor on the subendothelial layer to plasma proteins also activate the blood coagulation cascade, which ultimately activates thrombin and factor XIII. Factor XIII cross-links strands of polymerized fibrin monomers to form a stable clot (the secondary hemostatic plug). The blood coagulation cascade consists of a series of enzymes (such as factor X), which are inactive until they are proteolytically cleaved by the preceding enzyme in the cascade. Other proteins (factor V and factor VIII) serve as binding proteins, which assemble factor complexes at the site of injury. Ca2+ and γ-carboxyglutamate residues in the proteins (formed by a vitamin K–dependent process in the liver) attach the factor complexes to phospholipids exposed on platelet membranes. Consequently, thrombus formation is rapidly accelerated and localized to the site of injury.
Regulatory mechanisms within the blood coagulation cascade and antifibrinolytic mechanisms prevent random coagulation within blood vessels that might obstruct blood flow. Impairments in these mechanisms lead to thrombosis.
THE WAITING ROOM 
Peter K., a 6-month-old male infant, was brought to his pediatrician’s office with a painful, expanding mass in his right upper thigh that was first noted just hours after he fell down three uncarpeted steps in his home. The child appeared to be in severe distress.
A radiograph showed no fractures, but a soft tissue swelling, consistent with a hematoma (bleeding into the tissues), was noted. Peter K.’s mother related that soon after he began to crawl, his knees occasionally became swollen and seemed painful.
The pediatrician suspected a disorder of coagulation. A screening coagulation profile suggested a possible deficiency of factor VIII, a protein involved in the formation of blood clots. Peter K.’s plasma factor VIII level was found to be only 3% of the average level found in normal subjects. A diagnosis of hemophilia A was made.
I. Plasma Proteins Maintain Proper Distribution of Water between Blood and Tissues
When cells are removed from the blood, the remaining plasma is composed of water, nutrients, metabolites, hormones, electrolytes, and proteins. Plasma has essentially the same electrolyte composition as other extracellular fluids and constitutes approximately 25% of the body’s total extracellular fluid. The plasma proteins serve a number of functions, including maintaining the proper distribution of water between the blood and the tissues, transporting nutrients, metabolites, and hormones throughout the body, defending against infection, and maintaining the integrity of the circulation through clotting. Many diseases alter the amounts of plasma proteins produced and hence their concentration in the blood. These changes can be determined by electrophoresis of plasma proteins over the course of a disease.
A. Body Fluid Maintenance between Tissues and Blood
As the arterial blood enters the capillaries, fluid moves from the intravascular space into the interstitial space (that surrounding the capillaries) due to Starling’s forces. The hydrostatic pressure in the arteriolar end of the capillaries (~37 mm Hg) exceeds the sum of the tissue pressure (~1 mm Hg) and the osmotic pressure of the plasma proteins (~25 mm Hg). Thus, water tends to leave the capillaries and enter extravascular spaces. At the venous end of the capillaries, the hydrostatic pressure falls to approximately 17 mm Hg while the osmotic pressure and the tissue pressure remain constant, resulting in movement of fluid back from the extravascular (interstitial) spaces and into the blood. Thus, most of the force bringing water back from the tissues into the plasma is the osmotic pressure mediated by the presence of proteins in the plasma.
B. The Major Serum Protein, Albumin
As indicated in Table 43.1, the liver produces a number of transport or binding proteins and secretes them into the blood. The major protein synthesized is albumin, which constitutes approximately 60% of the total plasma protein, but because of its relatively small size (69 kDa), it is thought to contribute 70% to 80% of the total osmotic pressure of the plasma. Albumin is a carrier of free fatty acids, calcium, zinc, steroid hormones, copper, and bilirubin. Many drugs also bind to albumin, which may have important pharmacologic implications. For example, when a drug binds to albumin, such binding will likely lower the effective concentration of that drug and may lengthen its lifetime in the circulation. Drug dosimetry may need to be recalculated if a patient’s plasma protein concentration is abnormal.
TABLE 43.1 Specific Plasma-Binding Proteins Synthesized in the Liver
Albumin | Binds free fatty acids, calcium, zinc, steroid hormones, copper, bilirubin |
Ceruloplasmin | Binds copper; appears to be more important as a copper storage pool than as a transport protein; integrates iron and copper homeostasis |
Corticosteroid-binding globulin | Binds cortisol |
Haptoglobin | Binds extracorpuscular heme |
Lipoproteins | Transport cholesterol and fatty acids |
Retinol-binding protein | Binds vitamin A |
Sex hormone–binding globulin | Binds estradiol and testosterone |
Transferrin | Transports iron |
Transthyretin | Binds thyroxine (T4); also forms a complex with retinol-binding protein |
II. The Plasma Contains Proteins That Aid in Immune Defense
Two different sets of proteins aid in the immune response, the immunoglobulins, and the complement proteins. The immunoglobulins are secreted by a subset of differentiated B lymphocytes termed plasma cells and bind antigens at binding sites formed by the hypervariable regions of the proteins (see Chapter 7). Once the antibody–antigen complex is formed, it must be cleared from the circulation. The complement system participates in this function. The complement system consisting of approximately 20 proteins becomes activated in either of two ways. The first is interaction with antigen–antibody complexes, and the second, specific for bacterial infections, is through interaction of bacterial cell polysaccharides with complement protein C3b. Activation of the complement system by either trigger results in a proteolytic activation cascade of the proteins of the complement system, resulting in the release of biologically active peptides or polypeptide fragments. These peptides mediate the inflammatory response, attract phagocytic cells to the area, initiate degranulation of granulocytes, and promote clearance of antigen–antibody complexes.
Protease inhibitors in plasma serve to carefully control the inflammatory response. Activated neutrophils release lysosomal proteases from their granules that can attack elastin, the various types of collagen, and other extracellular matrix proteins. The plasma proteins α1-antiproteinase (α1-antitrypsin) and α2-macroglobulin limit proteolytic damage by forming noncovalent complexes with the proteases, thereby inactivating them. However, the product of neutrophil myeloperoxidase, HOCl, inactivates the protease inhibitors, thereby ensuring that the proteases are active at the site of infection.
III. Plasma Proteins Maintain the Integrity of the Circulatory System
Blood is lost from the circulation when the endothelial lining of the blood vessels is damaged, and the vessel wall is partly or wholly severed. When this occurs, the subendothelial cell layer is exposed, consisting of the basement membrane of the endothelial cells and smooth muscle cells. In response to the damage, a barrier (the hemostatic plug, a fibrin clot), initiated by platelet binding to the damaged area, is formed at the site of injury. Regulatory mechanisms limit clot formation to the site of injury and control its size and stability. As the vessel heals, the clot is degraded (fibrinolysis). Plasma proteins are required for these processes to occur.
A. Formation of the Hemostatic Plug
1. The Platelet
Platelets are nonnucleated cells in the blood whose major role is to form mechanical plugs at the site of vessel injury and to secrete regulators of the clotting process and vascular repair. In the absence of platelets, leakage of blood from rents in small vessels is common. Platelets are derived from megakaryocytes in the bone marrow. Megakaryocytes differentiate from the hematopoietic stem cell. As the megakaryocyte matures, it undergoes synchronous nuclear replication without cellular division, to form a cell with a multilobed nucleus and enlarged cytoplasm. At approximately the eight-nucleus stage, the cytoplasm becomes granular, and the platelets are budded off the cytoplasm. A single megakaryocyte gives rise to approximately 4,000 platelets.
In the nonactivated platelet (a platelet that is not involved in forming a clot), the plasma membrane invaginates extensively into the interior of the cell, forming an open membrane (canalicular) system. Because the plasma membrane contains receptors and phospholipids that accelerate the clotting process, the canalicular structure substantially increases the membrane surface area that is potentially available for clotting reactions. The platelet interior contains microfilaments and an extensive actin/myosin system. Platelet activation in response to endothelial injury causes Ca2+-dependent changes in the contractile elements, which, in turn, substantially change the architecture of the plasma membrane. Long pseudopodia are generated, increasing the surface area of the membrane as clot formation is initiated.
Platelets contain three types of granules. The first is electron-dense granules, which contain calcium, adenosine diphosphate (ADP), adenosine triphosphate (ATP), and serotonin. The second type of granule is the α-granule, which contains a heparin antagonist (heparin interferes with blood clotting; see “Biochemical Comments”), platelet-derived growth factor, β-thromboglobulin, fibrinogen, von Willebrand factor (vWF), and other clotting factors. The third type of granule is the lysosomal granule, which contains hydrolytic enzymes. During activation, the contents of these granules, which modulate platelet aggregation and clotting, are secreted.
2. Platelet Activation
Three fundamental mechanisms are involved in platelet function during blood coagulation: adhesion, aggregation, and secretion. Adhesion sets off a series of reactions termed platelet activation, which leads to platelet aggregation and secretion of platelet granule contents.
The adhesion step refers primarily to the platelet-subendothelial interaction that occurs when platelets initially adhere to the sites of blood vessel injury (Fig. 43.1). Blood vessel injury exposes collagen, subendothelial matrix-bound vWF, and other matrix components. vWF is a protein synthesized in endothelial cells and megakaryocytes, and it is located in the subendothelial matrix, in specific platelet granules, and in the circulation bound to factor VIII. The platelet cell membrane contains glycoproteins (GPs) that bind to collagen and to vWF, causing the platelet to adhere to the subendothelium. Binding to collagen by GPIa (integrin α2β1) causes the platelet to change its shape from a flat disk to a spherical cell. The latter extrudes long pseudopods, which promote platelet/platelet interactions. Binding of subendothelial vWF by GPIb causes changes in the platelet membrane that expose GPIIb/IIIa (integrin αIIbβ3)-binding sites to fibrinogen and vWF.
FIGURE 43.1 Adhesion of platelets to the subendothelial cell layer. (1) GPIa initially binds to the exposed collagen, which results in changes in the three-dimensional configuration of the complex, allowing GPIb to bind to von Willebrand factor (Vwf) (2). (3) This second binding event exposes the GPIIb/GPIIIa complex, which also can bind to vWF and fibrinogen.
The initial adherence of platelets sets off a series of reactions (platelet activation) that results in more platelets being recruited and aggregated at the site of injury. After initial adherence, some of the platelets release the contents of their dense granules and their α-granules, with ADP release being of particular importance because ADP is a potent platelet activator. ADP released from the platelets and from damaged red blood cells binds to a receptor on the platelet membrane, which leads to the further unmasking of GPIIb/IIIa-binding sites. Aggregation of platelets cannot take place without ADP stimulation because ADP induces swelling of the activated platelets, promoting platelet/platelet contact, and adherence.
Fibrinogen is a protein that circulates in the blood and is also found in platelet granules. It consists of two triple helices held together with disulfide bonds (Fig. 43.2). Binding of fibrinogen to activated platelets is necessary for aggregation, providing, in part, the mechanism by which platelets adhere to one another. Cleavage of fibrinogen by thrombin (a protease that is activated by the coagulation cascade) produces fibrin monomers that polymerize and, together with platelets, form a “soft clot.” Thrombin itself is a potent activator of platelets, through binding to a specific receptor on the platelet surface.
FIGURE 43.2 Cleavage of fibrinogen results in clot formation. A. Fibrinogen, the precursor protein of fibrin, is formed from two triple helices joined together at their N-terminal ends. The α,β-peptides are held together by disulfide bonds, and the γ-peptides are joined to each other by disulfide bonds. The terminal α,β-peptide regions, shown in red, contain negatively charged glutamate and aspartate residues that repel each other and prevent aggregation. B. Thrombin, a serine protease, cleaves the terminal portions of fibrinogen that contain negative charges. The fibrin monomers can then aggregate and form a “soft” clot. The soft clot is subsequently cross-linked by another enzyme.
B. The Blood Coagulation Cascade
Thrombus (clot) formation is enhanced by thrombin activation, which is mediated by the complex interaction that constitutes the blood coagulation cascade. This cascade (Fig. 43.3) consists primarily of proteins that serve as enzymes or cofactors, which function to accelerate thrombin formation and localize it at the site of injury. These proteins are listed in Table 43.2. All of these proteins are present in the plasma as proproteins (zymogens). These precursor proteins are activated by cleavage of the polypeptide chain at one or more sites. The key to successful and appropriate thrombus formation is the regulation of the proteases that activate these zymogens.
TABLE 43.2 Proteins of Blood Coagulation
FACTOR | DESCRIPTIVE NAME | FUNCTION/ACTIVE FORM |
Coagulation Factors | ||
I | Fibrinogen | Fibrin |
II | Prothrombin | Serine protease |
III | Tissue factor | Receptor and cofactor |
IV | Ca2+ | Cofactor |
V | Proaccelerin, labile factor | Cofactor |
VII | Proconvertin | Serine protease |
VIII | Antihemophilia factor A | Cofactor |
IX | Antihemophilia factor B, Christmas factor | Serine protease |
X | Stuart–Prower factor | Serine protease |
XI | Plasma thromboplastin antecedent | Serine protease |
XIII | Fibrin-stabilizing factor | Ca2+-dependent transglutaminase |
Regulatory Proteins | ||
Thrombomodulin | Endothelial cell receptor binds thrombin | |
Proteins C and S | Activated by thrombomodulin-bound thrombin; is a serine protease cofactor; binds activated protein C |
FIGURE 43.3 The blood coagulation cascade. Activation of clot formation occurs through interlocking pathways, termed the intrinsic and extrinsic pathways. The intrinsic pathway is activated when factor XI is converted to factor XIa by thrombin. The extrinsic pathway (external damage, such as a cut) is activated by tissue factor. The reactions designated by “PL, Ca” are occurring through cofactors bound to phospholipids (PL) on the platelet and blood vessel endothelial cell surface in a Ca2+-coordination complex. Factors XIa, IXa, VIIa, Xa, and thrombin are serine proteases. Note the positive feedback regulation of thrombin on the activation of proteases earlier in the cascade sequence (indicated by the dashed lines).
The proenzymes (factors VII, XI, IX, X, and prothrombin) are serine proteases that, when activated by cleavage, cleave the next proenzyme in the cascade. Because of the sequential activation, great acceleration and amplification of the response are achieved. That cleavage and activation have occurred are indicated by the addition of an “a” to the name of the proenzyme (e.g., factor IX is cleaved to form the active factor IXa).
The cofactor proteins (tissue factor, factors V and VIII) serve as binding sites for other factors. Tissue factor is not related structurally to the other blood coagulation cofactors and is an integral membrane protein that does not require cleavage for active function. Factors V and VIII serve as procofactors, which, when activated by cleavage, function as binding sites for other factors.
Two additional proteins that are considered part of the blood coagulation cascade, protein S and protein C, are regulatory proteins. Only protein C is regulated by proteolytic cleavage, and when it is activated, it is itself a serine protease.
Additionally, in response to collagen and thrombin, platelets release vasoconstrictors. Serotonin is released from the dense granules of the platelets, and the synthesis of thromboxane A2 is stimulated. This reduces blood flow to the damaged area. Platelet-derived growth factor, which stimulates proliferation of vascular cells, is also released into the environment surrounding the damage.
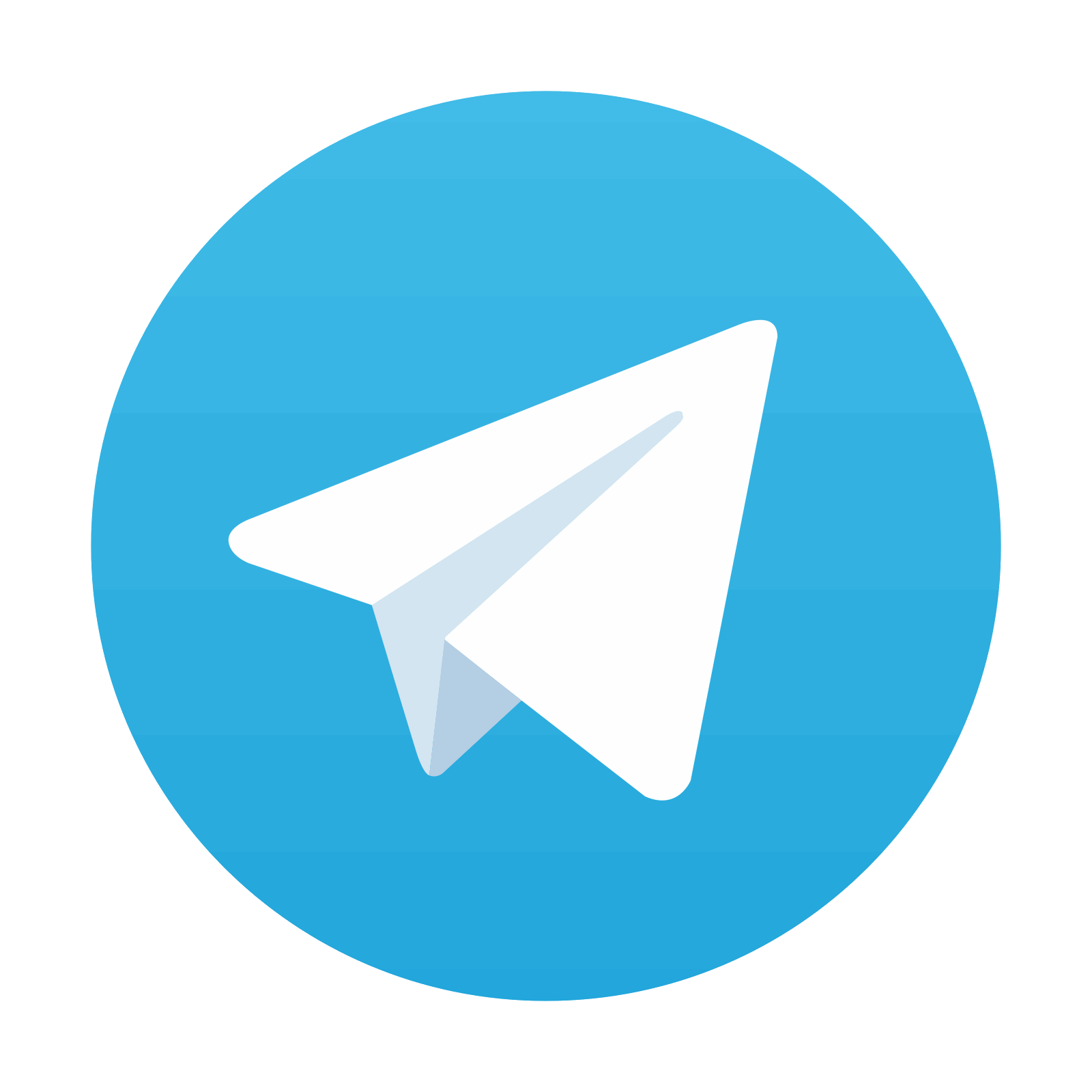
Stay updated, free articles. Join our Telegram channel

Full access? Get Clinical Tree
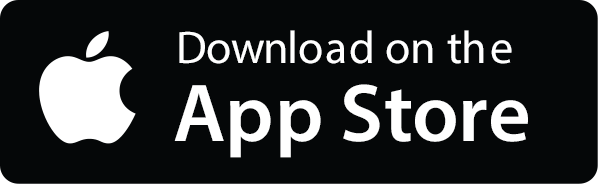
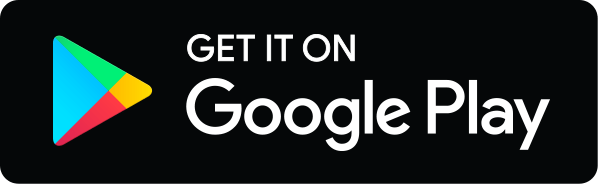