Anatomy of the Muscular System
SKELETAL MUSCLE STRUCTURE
Connective Tissue Components
The skeletal muscle cells, or muscle fibers, are covered by a delicate connective tissue membrane called the endomysium (Figure 11-1). Groups of skeletal muscle fibers, called fascicles, are then bound together into bundles by a tougher connective tissue envelope called the perimysium. The muscle as a whole is covered by a coarse sheath called the epimysium. Because all three of these structures are continuous with the fibrous structures that attach muscles to bones or other structures, muscles are firmly harnessed to the structures they pull on during contraction. The epimysium, perimysium, and endomysium of a muscle, for example, may be continuous with fibrous tissue that extends from the muscle as a tendon, a strong tough cord continuous at its other end with the fibrous periosteum covering a bone. Alternatively, the fibrous wrapping of a muscle may extend as a broad, flat sheet of connective tissue called an aponeurosis, which usually merges with the fibrous wrappings of another muscle. So tough and strong are tendons and aponeuroses that they are not often torn, even by injuries forceful enough to break bones or tear muscles. They are, however, occasionally pulled away from bones.
Fibrous connective tissue surrounding the muscle organ and located outside the epimysium and tendon is called fascia. Fascia is a general term for the fibrous connective tissue found under the skin and surrounding many deeper organs, including skeletal muscles and bones. Fascia just under the skin (the hypodermis) is sometimes called superficial fascia, and the fascia around muscles and bones is sometimes called deep fascia.
Sheets of fibrous connective tissue form double-walled tubes called tendon sheaths that enclose certain tendons, notably those of the wrist and ankle. Like bursae, the walls of tendon sheaths have a lining of synovial membrane. The moist, smooth surfaces that face each other within the double wall of a tendon sheath enables the tendon to move easily, almost without friction. Table 11-1 clarifies the arrangement of the fibrous coverings of a muscle organ.
TABLE 11-1
Fibrous Coverings of Muscle Organs
FIBROUS STRUCTURE* | PART COVERED |
Fascia | External to muscles, bones, other organs |
Superficial fascia | Under the skin |
Deep fascia | Surrounds deeper organs, including epimysium of muscle |
Tendon sheath | Tubelike tunnel around tendon of muscle; lined with synovial membrane |
Epimysium† | Surrounds entire muscle organ |
Perimysium† | Surrounds a fascicle (bundle) of muscle fibers |
Endomysium† | Surrounds an individual muscle fiber |
*Listed here from superficial to deep; all of these fibrous structures are continuous with one another (that is, their fibers blend together).
†Continue and fuse together to form a tendon or aponeurosis.
Size, Shape, and Fiber Arrangement
The structures called skeletal muscles are organs. They consist mainly of skeletal muscle tissue plus important connective and nervous tissue components. Skeletal muscles vary considerably in size, shape, and arrangement of fibers. They range from extremely small strands, such as the stapedius muscle of the middle ear, to large masses, such as the muscles of the thigh. Some skeletal muscles are broad in shape and some are narrow. Some are long and tapering and some are short and blunt. Some are triangular, some quadrilateral, and some irregular. Some form flat sheets and others form bulky masses.
The strength and type of movement produced by the shortening of a muscle is related to the orientation of its fibers and overall shape, as well as its attachments to bone and involvement in joints. This is yet another example of the relationship between structure and function. Six muscle shapes are often used to describe and categorize skeletal muscles (Figure 11-2).
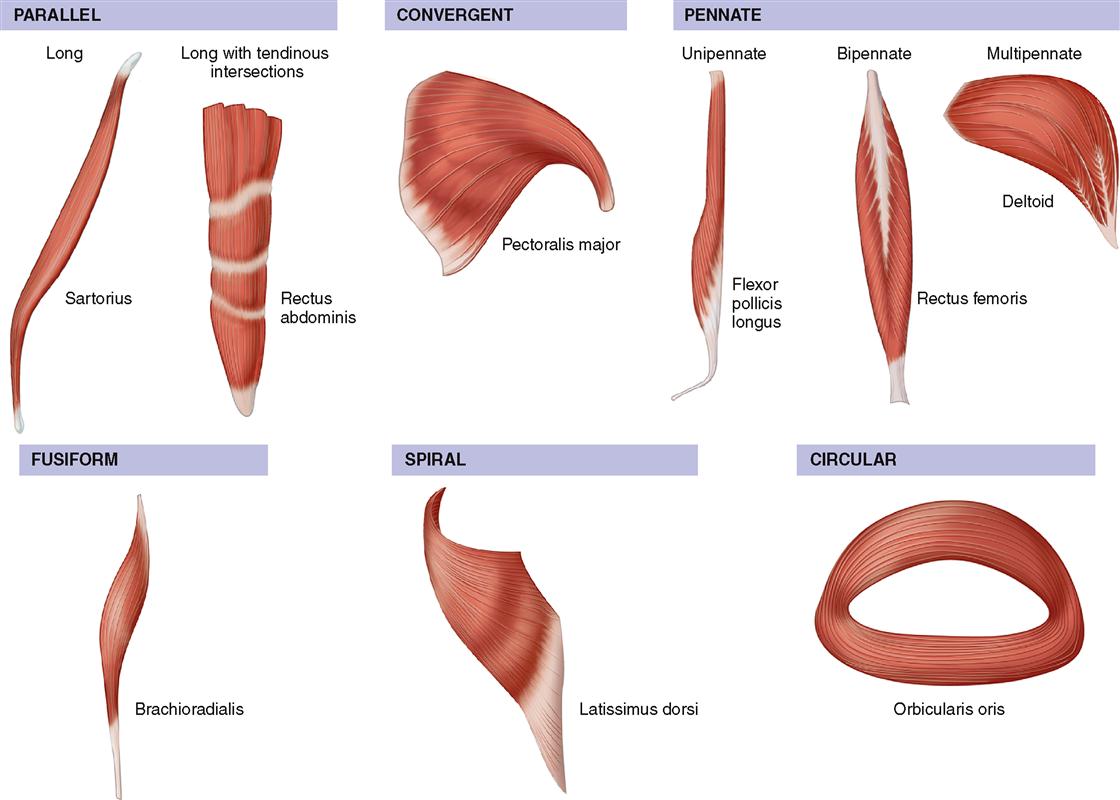
Attachment of Muscles
Most of our muscles span at least one joint and attach to both articulating bones. When contraction occurs, one bone usually remains fixed and the other moves. The points of attachment are called the origin and insertion. The origin is the point of attachment that does not move when the muscle contracts. Therefore, the origin bone is the more stationary of the two bones at a joint when contraction occurs. The insertion is the point of attachment that moves when the muscle contracts (Figure 11-3). The insertion bone therefore moves toward the origin bone when the muscle shortens. In case you are wondering why both bones do not move because both are pulled on by the contracting muscle, the answer is one of them is normally stabilized by isometric contractions of other muscles or by certain features of its own that make it less mobile.
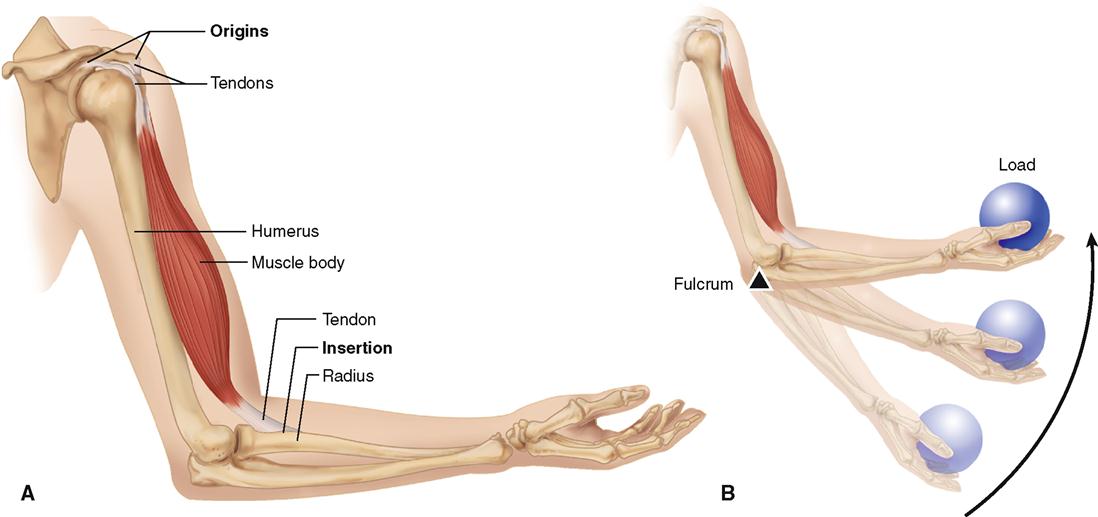
The terms origin and insertion provide us with useful points of reference. Many muscles have multiple points of origin or insertion. Understanding the functional relationship of these attachment points during muscle contraction helps in deducing muscle actions. The attachment points of the biceps brachii shown in Figure 11-3 help provide functional information. Distal insertion on the radius in the forearm causes flexion to occur at the elbow when contraction occurs. It should be realized, however, that origin and insertion are points that may change under certain circumstances. For example, not only can you grasp an object above your head and pull it down, but you can also pull yourself up to the object. Although origin and insertion are convenient terms, they do not always provide the necessary information needed to understand the full functional potential of muscle action.
Muscle Actions
Skeletal muscles almost always act in groups rather than singly. As a result, most movements are produced by the coordinated action of several muscles. Some of the muscles in the group contract while others relax. The result is a movement pattern that allows for the functional classification of muscles or muscle groups. Several terms are used to describe muscle action during any particular movement pattern. The terms prime mover (agonist), antagonist, synergist, and fixator are especially important and are discussed in the following paragraphs. Each term suggests an important concept that is essential to understanding such functional muscle patterns as flexion, extension, abduction, adduction, and other movements discussed in Chapter 10.
Any muscle that performs an action is a “mover.” The term prime mover is used to describe a muscle that directly performs a specific movement. The movement produced by a muscle acting as a prime mover is described as the “action” or “function” of that muscle. For example, the biceps brachii shown in Figures 11-3 and 11-4 is acting as a prime mover during flexion of the forearm. The term agonist can be applied to the prime mover, or any other “mover” muscle that directly contributes to the same action as the prime mover.
Antagonists are muscles that when contracting, directly oppose prime movers (or agonists). They are relaxed while the prime mover is contracting to produce movement (see Figure 11-4). Simultaneous contraction of a prime mover and its antagonist muscle results in rigidity and lack of motion. The term antagonist is perhaps unfortunate because muscles cooperate, rather than oppose, in normal movement patterns. Antagonists are important in providing precision and control during contraction of prime movers.
Synergists are muscles that contract at the same time as the prime mover. They facilitate or complement prime mover actions so that the prime mover produces a more effective movement.
Fixator muscles generally function as joint stabilizers (see Figure 11-4, B). They frequently serve to maintain posture or balance during contraction of prime movers acting on joints in the arms and legs. Because fixator muscles assist the prime mover in performing an action, they are a type of synergist.
Movement patterns are complex, and most muscles function not only as prime movers but also at times as antagonists, synergists, or fixators. A prime mover in a particular movement pattern, such as flexion, may be an antagonist during extension or a synergist or fixator in other types of movement.
Lever Systems
When a muscle shortens, the central body portion, called the belly, contracts. The type and extent of movement are determined by the load or resistance that is moved, the attachment of the tendinous extremities of the muscle to bone (origin and insertion), and the particular type of joint involved. In almost every instance, muscles that move a part do not lie over that part. Instead, the muscle belly lies proximal to the part moved. Thus muscles that move the forearm lie proximal to it, that is, in the upper part of the arm.
Knowledge of lever systems is important in understanding muscle action. By definition, a lever is any rigid bar free to turn about a fixed point called its fulcrum. Bones serve as levers, and joints serve as fulcrums of these levers. A contracting muscle applies a pulling force on a bone lever at the point of the muscle’s attachment to the bone (Box 11-1). This force causes the insertion bone to move about its joint fulcrum.
A lever system is a simple mechanical device that makes the work of moving a weight or other load easier in some way. Lever systems are composed of four component parts:
1. A rigid rod or bar (bone) called a lever
2. A fixed pivot, or fulcrum (F), around which the lever moves (joint)
3. A load (L), or resistance, that is moved
4. A force, or pull (P), which produces movement (muscle contraction).
Figure 11-5 shows the three different types of lever arrangements. All three types are found in the human body.
FIRST-CLASS LEVERS
As you can see in Figure 11-5, A, the fulcrum in a first-class lever lies between the effort, or pull (P), and the resistance, or load (W), as in a set of scales, a pair of scissors, or a child’s seesaw. In the body the head being raised or tipped backward on the atlas is an example of a first-class lever in action. The facial portion of the skull is the load, the joint between the skull and atlas is the fulcrum, and the muscles of the back produce the pull. In the human body first-class levers are not abundant. They generally serve as levers of stability.
SECOND-CLASS LEVERS
In second-class levers the load lies between the fulcrum and the joint at which the pull is exerted. The wheelbarrow is often used as an example. The presence of second-class levers in the human body is a controversial issue. Some authorities interpret the raising of the body on the toes as an example of this type of lever (see Figure 11-5, B). In this example the point of contact between the toes and the ground is the fulcrum, the load is located at the ankle, and pull is exerted by the gastrocnemius muscle through the Achilles tendon. Opening the mouth against resistance (depression of the mandible) is also considered to be an example of a second-class lever.
THIRD-CLASS LEVERS
In a third-class lever the pull is exerted between the fulcrum and the resistance or load to be moved. Flexing of the forearm at the elbow joint is a frequently used example of this type of lever (see Figure 11-5, C). Third-class levers permit rapid and extensive movement and are the most common type found in the body. They allow insertion of a muscle very close to the joint that it moves.
HOW MUSCLES ARE NAMED
The first thing you may notice as you start studying the muscles of the body is that many of the names seem difficult and foreign. The terms are less difficult if you keep in mind that most anatomical terms come from Latin.
Although we have strived to use only English names in this edition, some Latin terms still remain in common usage—especially in anatomy. This is certainly true in the health-related disciplines. For example, in some texts the deltoid muscle is called the deltoideus (Latin) and in others the deltoid (English). To minimize confusion, the terms used here will be the English versions from the Terminologia Anatomica (see Chapter 1). Even so, you will soon discover that the English versions are often the same or similar to the Latin versions!
Regardless of the muscle name used, when one understands the reasons for the term used, it will seem more logical and be easier to learn and understand. Many of the muscles of the body shown in Figure 11-6 or listed in Tables 11-2 through 11-6 are named according to one or more of the following features:
Location. Many muscles are named as a result of location. The brachialis (arm) muscleand gluteus (buttock) muscles are examples. Table 11-2 gives a listing of some major muscles grouped by location.
Function. The function of a muscle often is a part of its name. The adductor muscles of the thigh adduct, or move, the leg toward the midline of the body. Table 11-3 lists selected muscles grouped according to function.
Shape. Shape is a descriptive feature used for naming many muscles. The deltoid (triangular) muscle covering the shoulder is deltoid, or triangular, in shape (see Table 11-4).
Direction of fibers. Muscles may be named according to the orientation of their fibers. The term rectus means straight. The fibers of the rectus abdominis muscle run straight up and down and are parallel to each other (see Table 11-5).
Number of heads or divisions. The number of divisions or heads (points of origin) may be used to name a muscle. The word part -cep- means head. Biceps (two), triceps (three), and quadriceps (four) refer to multiple heads, or points of origin. The biceps brachii is a muscle having two heads located in the arm (see Table 11-5).
Size of muscle. The relative size of a muscle can be used to name a muscle, especially if it is compared to the size of nearby muscles (see Table 11-6). For example, the gluteus maximus is the largest muscle of the gluteal (Greek glautos, meaning “buttock”) region. Nearby, there is a small gluteal muscle, the gluteus minimus, and a midsize gluteal muscle, the gluteus medius.
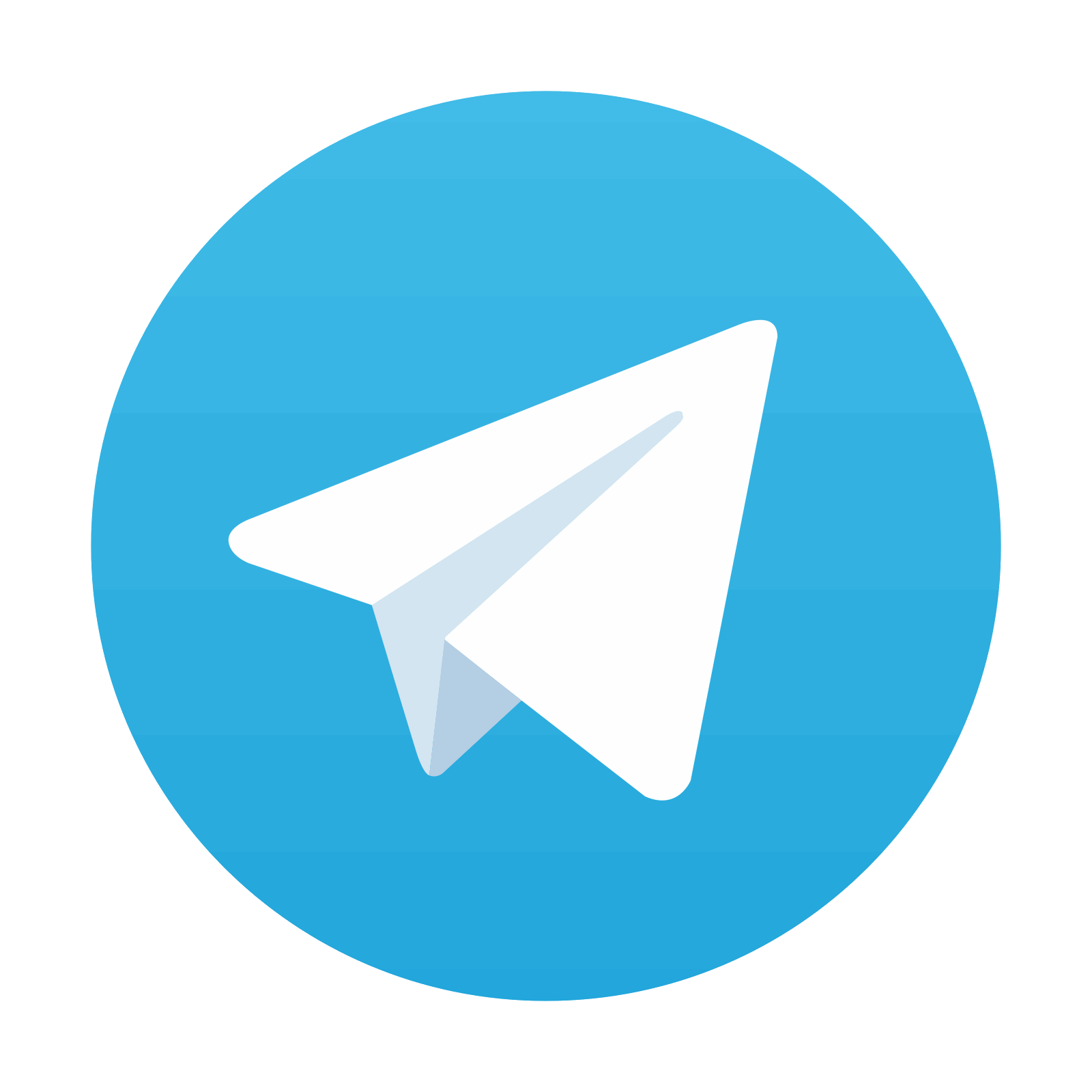
Stay updated, free articles. Join our Telegram channel

Full access? Get Clinical Tree
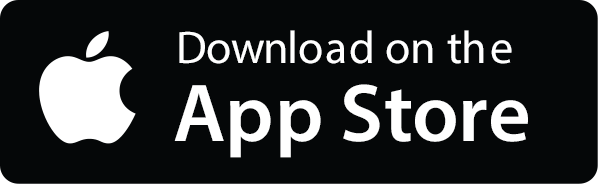
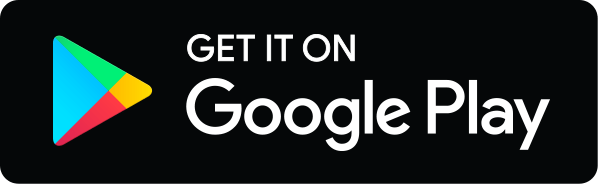
