Figure 11.1. Toxic test substances cause changes at the molecular level (DNA or protein), which are expressed at the cellular level, which in turn result in target organ toxicity (e.g. liver, kidney), which can be studied and extrapolated to whole animal or humans based on in vitro studies.
Likewise, human cell lines have been used in search of effective emerging and existing therapies for certain diseases. For example, it is known that there are 51 types of breast cell lines, which originated from 145 human breast tumours.6 These cell lines retain most of the genetic information for autonomous growth. These cell lines have been successfully used to understand molecular mechanisms of sensitivity/resistance to inhibitors with exquisite sensitivity for epidermal growth factor receptor, human epidermal growth factor receptor 2, hepatocyte growth factor receptor and BRAF kinase inhibitors.7 These cell lines indeed paved ways for the use of trastuzumab (Herceptin®) as an adjuvant therapy in the treatment of breast cancer in some individuals.
Organisations Researching and Funding Alternatives to Animal Testing
Center for Alternatives to Animal Testing8
The Johns Hopkins University Center for Alternatives to Animal Testing (CAAT) has worked with scientists since 1981 to find new methods to replace the use of laboratory animals in experiments, reduce the number of animals tested and refine necessary tests to eliminate pain and distress to test animals. The CAAT promotes humane science by supporting the creation, development, validation and use of alternatives to animals in research, product safety testing and education. It is not an activist group; rather, it seeks to effect change by working with scientists in industry, government and academia.
Interagency Coordinating Committee on the Validation of Alternative Methods9
Traditionally, consumer products, medical devices, chemicals and new drugs are tested on animals to predict toxicity, metabolism and efficacy in humans. Scientists in Interagency Coordinating Committee on the Validation of Alternative Methods (ICCVAM) are working to promote the development and validation of alternative test methods. Alternative test methods are those that accomplish one or more of the 3Rs principles.
University of California, Davis Center for Animal Alternatives10
UC Davis Center for Animal Alternatives disseminates information on alternatives to animal experiments with information on the most current methods for improving all aspects of animal care.
Dr. Hadwen Trust11
The Dr Hadwen Trust for Humane Research is a British biomedical research charity, founded in 1970. It funds peer-reviewed alternatives to animal testing in relation to health issues, such as cancer, heart disease, meningitis and Alzheimer’s disease. Trust researchers have cultured human cartilage, which helped to show how arthritis drugs work; developed a three-dimensional model of human teeth and jawbone for dental research; created a computer model of a human foetus; and used mathematical modelling to create drug treatment for non-Hodgkin’s lymphoma and neuroblastoma. Over 200 papers about the Trust’s work have been published in scientific journals.
National Center for the Replacement, Refinement and Reduction of Animals in Research12
The European Center for the Validation of Alternative Methods (ECVAM) launched an online database on toxicology of non-animal alternative test methods in 2006. Categories at present include in vitro methods, quantitative structure activity relationship (QSAR) models and a bibliographic section, aiming to develop alternatives to animal testing. This describes general guidance to researchers and associated veterinary and animal care staff using vertebrates (live animals or animal products) in bioscience research funded by various agencies. It sets out the expectations of the funding bodies for the use of such animals in research and is therefore useful to ethics committees, referees and members involved in reviewing research proposals. Implementation of the principles in this guidance is a condition of receiving funds from the funding bodies.
How to Achieve 3Rs with Minimal to No Animal Testing?
Over the years, several ways have been identified and practiced, where possible, in achieving 3Rs. Some of the examples are as follows:
These strategies are described below.
In Silico Computer Simulation
There are a variety of in silico computer simulation softwares available in the public domain and on a fee-based service. Computers can often predict the toxicity of chemicals, including their potential to cause cancer or birth defects, based on their molecular structure. Computer simulations can also predict the metabolism and distribution of chemicals in human tissues.
Lhasa limited is a not-for-profit organisation that promotes the sharing of data and knowledge in chemistry and life sciences (http://www.lhasalimited.org/index.php). It has developed software tools such as DEREK (deductive estimation of risk based on existing knowledge) for Windows™, Meteor and Vitic to facilitate such sharing. DEREK for Windows™ and Meteor are knowledge-based expert systems that predict the toxicity and metabolism of a chemical, respectively. Vitic is a chemically intelligent toxicity database. These software tools include illustrations on (i) the use of data entry and editing tools for the sharing of data and knowledge within organisations; (ii) the use of proprietary data to develop non-confidential knowledge that can be shared between organisations; (iii) the use of shared expert knowledge to refine predictions; (iv) the sharing of proprietary data between organisations through the formation of data-sharing groups and (v) the use of proprietary data to validate predictions. Sharing of chemical toxicity and metabolism data and knowledge in this way offers a number of benefits, including the possibilities of rapid scientific progress and reductions in the use of animals in testing. Maximising the accessibility of data also becomes increasingly crucial as in silico systems move towards the prediction of more complex phenomena for which limited data are available. Recently, it has been shown that the use of in silico methods is the way forward for assessing the genotoxicity and potential carcinogenicity/repeat dose general toxicology of large numbers of chemicals.14 Importantly, it is expected that these methods will contribute to the reduction of animal use.
There are a number of other in silico methods which have been developed and validated, for example, Leadscope, MCase, Topkat, Toxtree and Osiris software, as well as QSAR models developed by the FDA with proprietary databases.15 It is proposed that, depending on the type of molecule being tested and the type of safety information being sought, one or more softwares may be used to understand the structural alters for the endpoint desired. As the data outputs are reviewed, a consensus and reproducibility of alerts in at least two or more databases may be considered as significant alerts.
In Vitro Cell Culture Techniques16–19
In vitro cell culture is currently the most successful, and promising, alternative to animal use. Isolated cells, tissues and organs can be prepared and maintained in culture by methods that preserve properties and characteristics of the same cells, tissues and organs in vivo. Using such in vitro systems will permit data to be generated under controlled experimental conditions that are, in the absence of many complicating factors, characteristic of experiments in whole animals. For example, cultured cells have also been developed to create monoclonal antibodies thereby replacing use of animals required to undergo a procedure likely to cause pain and distress. An enormous amount of valuable in vitro cell culture research is conducted today. The National Disease Research Interchange20 provides more than 130 types of human tissue to scientists investigating more than 50 diseases, including cancer, diabetes and glaucoma. Cell and tissue cultures are used to screen new therapies and to test for product safety. Genetic microarrays21 are being used to predict liver toxicity by measuring gene expression in human liver cells.
Limitations of Alternative In Vitro Tests
A variety of limitations of in vitro tests have been described. Briefly, the in vitro test systems are not available for all tissues and organs. Further, normal systemic mechanism of absorption, distribution, metabolism and excretion systems is absent under culture conditions. In vitro systems lack the complex, interactive effects of the immune, blood, endocrine system, nervous system and other integrated elements of the whole animal. Therefore, in vitro tests cannot be used to study the complex nature of systemic toxicity. Finally, validation of new methods is time consuming and expensive. Acceptance of in vitro tests as alternatives to traditional toxicity testing in whole animals is expected to be slow. However, significant amount of progress has been made towards regulatory acceptance of such methods, for example, the Registration, Evaluation, Authorisation and Restriction of Chemicals (REACH) programme22 by the European Union has implemented acceptance of in vitro data for regulatory purposes.
Applications of In Vitro Tests
In vitro genetic toxicology testing23–26: In vitro genetic toxicology tests have routinely been used for over 30 years to predict the carcinogenicity of substances that are of safety concern. Such assays include, for example, bacterial assays, in vitro cell culture assays for chromosomal aberration assessment and cytotoxicity assessment.
- Bacterial mutation assay: This assay measures genetic damage at the single base level in DNA by using five or more tester strains of bacteria. Each of the Salmonella typhimurium and Escherichia coli strains used in the assay have a unique mutation that turns off histidine biosynthesis in Salmonella or tryptophan biosynthesis in E. coli. Because of these original mutations, the bacteria require exogenous histidine or tryptophan to survive and will starve to death if grown without these essential nutrients (auxotrophy). The key to the assay is the bacteria that can undergo a reverse mutation turning the essential gene back on permitting the cell to grow in the absence of either histidine or tryptophan (prototrophy). A specific type of mutation, either a base-pair substitution or frame-shift mutation, created each bacterial strain. The standard Ames assay designs include preliminary toxicology tests or combined toxicity and mutation tests followed by a definitive mutation assay. In both toxicity and mutation tests, tester strains are combined with S9 mix (prepared from the livers of Aroclor-treated rats) buffer, test or control article, a trace of histidine or tryptophan and molten agar. The bacteria use the trace histidine or tryptophan to undergo several cell divisions, but will stop growing once they have run out, leaving a characteristic ‘background lawn’ that decreases in density with increasing toxicity. After 48 h, only those cells that have undergone a reverse mutation turning the essential gene back on have survived, producing mutant colonies. The background lawn density is scored followed by counting the number of revertant colonies. Mutation results are reported as revertants per plate. These assays are performed in the presence and absence of exogenous metabolic activation systems (S9 mix).
- Chromosome aberration assay in cultured cells: In vitro cytogenetic analysis provides a valuable technique for evaluation of damage to chromosomes on the basis of direct observation and classification of chromosomal aberrations. Cells arrested in metaphase are examined microscopically for both numerical and structural chromosome aberrations. An alternate cytogenetic approach is the observation of micronuclei, which represent chromosomal-breakage fragments or lagging chromosomes in the cell cytoplasm. The cytogenetic assays may be performed either in vivo or in vitro. In vitro cytogenetic assays are performed in the presence and absence of S9 mix.
- Comet or single cell gel (SCG) electrophoresis assay27: Comet assay is a microgel electrophoresis technique that detects DNA damage and repair in individual cells. The damage is represented by an increase of DNA fragments that have migrated out of the cell nucleus in the form of a characteristic streak similar to the tail of a comet. The DNA fragments are generated by DNA double-strand breaks, single strand breaks and/or strand breaks induced by alkali-labile sites in the alkaline version of the assay. The length and fragment content of the tail is directly proportional to the amount of DNA damage (Figure 11.2). Comet assay can be conducted in both in vitro and in vivo test systems and is increasingly being used in genotoxic testing of industrial chemicals, agrochemicals and pharmaceuticals. Comet assay is rapid (results are available in days), simple to perform, requires small amounts of test substance (25–50 mg) and can be performed in almost any eukaryotic cell (different animal target organs). Comet assay serves as an important tool in the early drug development as a mechanistic and genotoxic predictor.
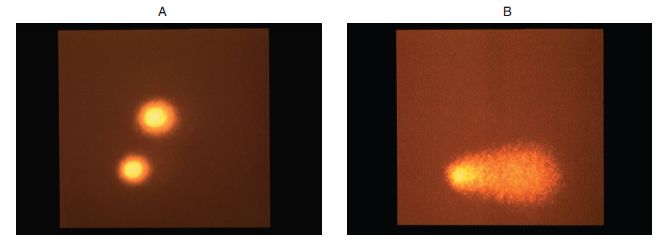
Figure 11.2. Photomicrographs of In vitro comet assay in Chinese hamster ovary cells. (A) Control cell with normal intact nuclei following electrophoresis (no DNA damage). (B) A test substance treated cell showing DNA migration due to DNA-strand breakage (damage), in the form of a comet following electrophoresis.
In vitro pharmacologic activity testing: Pharmacology is the study of drug effects in biological systems, including therapeutic or toxic effects. The field of in vitro pharmacology takes advantage of the technological advances in studying drug effects in the lab settings using cell culture models and isolated tissue assays and predicts such effects to in vivo models. In vitro pharmacology data provides invaluable information on the compounds’ chances to succeed at the clinical phase. Multiple contract research organisations offer a variety of panels of validated in vitro pharmacological assays that cover a broad range of targets. Apart from ascertaining the potency of a new chemical entity (NCE) on target, assays are also made available for selectivity screening and profiling. These assays are available either as stand-alone or as a screening platform. See Chapter 5 for study protocol examples.
- Isolated tissues: In the study of the molecular mechanism of drug action, receptors are the most important link in the chain of events. The characteristics of the receptors are studied by the observations and quantitation of some physiological response elicited by a tissue in response to a drug. This constitutes the pharmacological approach and is an indirect study of the drug-receptor interaction. Pharmacological activity of a compound can be easily, quickly and inexpensively obtained from isolated tissue experiments. Because of their simplicity, researchers in the early 1900s were able to define the interaction between drugs and receptors, predict drug effects and formulate mathematical models of drug receptor theory. Isolated tissue techniques revolutionised and dominated the field until the end of the 20th century. Readers are directed to read excellent reviews by Kenakin where the author discusses very important concepts in isolated tissue methods and procedures for measuring drug activity in isolated tissues.28,29
- A variety of isolated tissues for each receptor class have been described in literature. Three of the most widely used classes of isolated tissues are from cardiac muscle, vascular smooth muscle, gastrointestinal and other smooth muscles.
- Receptor-binding studies: Radioligand-binding studies were developed to identify and characterise receptor sites in various tissues. These studies provided insights into molecular interactions between ligands (endogenous and exogenous) and receptors; clarified the modes of drug action and the general mechanism that can lead to altered receptor function. Two principal parameters that are determined with these studies are the ‘density (Bmax)’ of a given class of receptor sites and their ‘affinities’ for various ligands. The values determined from these studies are compared with those derived from in vitro and in vivo functional studies. There are subtle differences between pharmacological methods and radioligand-binding procedures in regard to the characterisation of receptors. Binding studies lack a functional response. However, agonist’s interactions with binding sites and their sensitivity to temperature, various ions and often to guanine nucleotides can provide some indication of efficacy. Disruption of the tissue to prepare membranes or solubilisation may alter the pharmacological characteristics of receptor sites. With membrane preparations, there are few diffusional barriers and thus the actual concentration of the drug in the receptor compartment can be fairly estimated. In addition, radioligand-binding studies identify ligand-binding sites, which may or may not represent bonafide receptors. Thus, results must be treated cautiously.
- In drug discovery programme, radioligand-binding studies are developed and performed for several therapeutic targets such as G protein-coupled receptors (GPCRs), ion channels (including hERG) and transporters using membranes from recombinant cells as well as native tissues.
- GPCR functional assays: In addition, functional assays are used as a follow-up screen on GPCR targets. Ligand binding to GPCR promotes G-protein coupling initiating signal transduction pathways, which trigger a series of cellular responses. These data can be used to substantiate the binding data.
- Enzyme assays: Enzymes are increasingly recognised as potential drug targets in drug discovery. Multiple contract research organisations offer assays for several enzyme classes using different readouts like fluorescence, luminescence, time-resolved fluorescence and radiometry. The following are a few examples of enzymes that have been validated and are available for applications in pharmacology research.
Commonly used enzymes for pharmacological studies
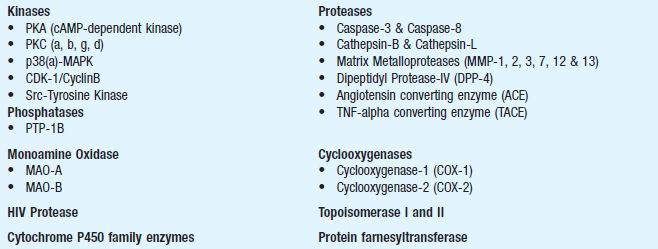
In vitro drug disposition studies: In Chapter 7, a variety of protocols have been described on absorption, distribution, metabolism and elimination (ADME). These are the processes by which the body handles chemicals, referred to as drug disposition studies. The process of pharmacodynamics, however, describes how chemicals or test substances affect the living organisms at the molecular, cellular, organ, host and population level. As understanding of the molecular basis for ADME properties evolves, it is becoming possible to move beyond studying pharmacokinetics in intact people or animals. By combining data collected in vitro characterising specific processes with computer models describing the interplay among the pharmacokinetic processes in the whole body, one can predict what would be expected to occur if intact organisms were exposed. Integrating data or predictions about chemical concentrations in different organs in the body with data or predictions about effects those chemicals would be expected to cause provides a long-term path towards predicting beneficial (therapeutic) or adverse (toxicological) effects. The success of this long-term path depends upon continued research on the basic biological processes underlying pharmacokinetics, further development of in vitro systems both for ADME processes and for effects, and improved computer models. The major uses of in vitro ADME studies include the following:
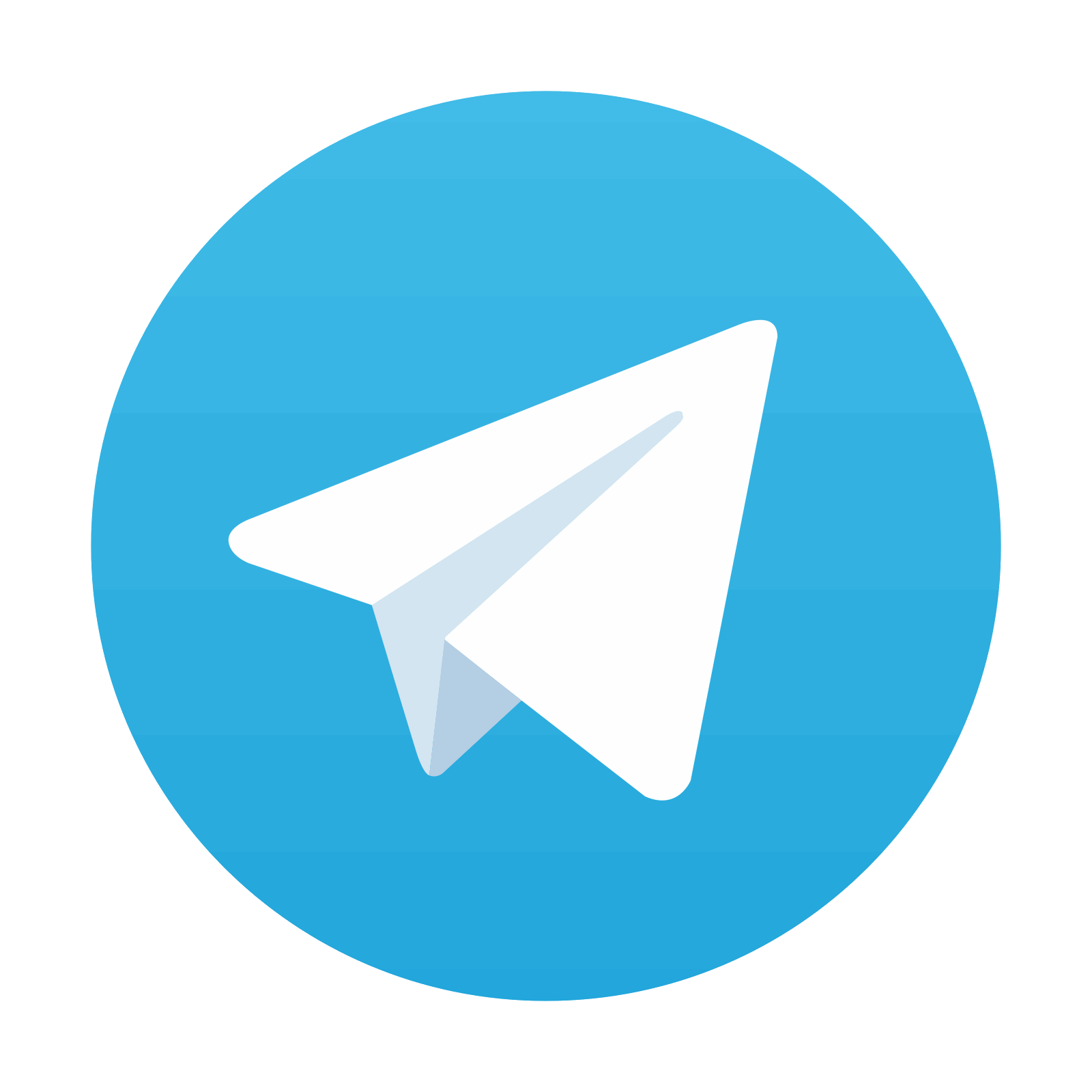
Stay updated, free articles. Join our Telegram channel

Full access? Get Clinical Tree
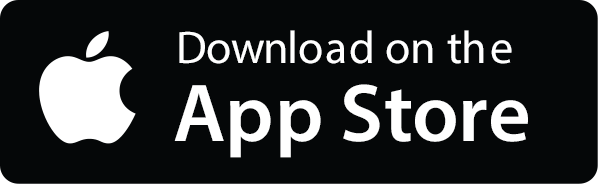
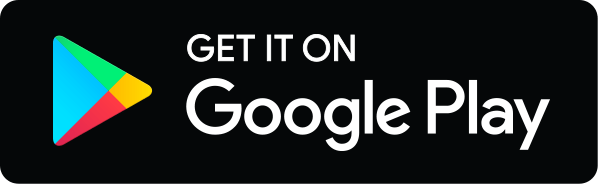