Alterations in Musculoskeletal Function
Trauma, Infection, and Disease
Carol L. Danning
Key Questions
• What are the key factors in the mechanisms of wound healing?
• What is the process and duration of normal bone healing after a fracture?
• What are the different complications that can occur following a fracture?
• What are the manifestations, dangers, and management of compartment syndrome?
• What are the clinical findings and management of bone infections?
• How are osteoporosis and osteomalacia or rickets similar, and how do they differ?
• What terminology is used to describe primary bone tumors?
• What are the cause and pathogenesis of muscular dystrophy and myasthenia gravis?
• Describe the clinical features and treatment options in fibromyalgia syndrome.
http://evolve.elsevier.com/Copstead/
A smoothly functioning musculoskeletal system facilitates a complete range of human actions, including walking, talking, running, breathing, and a myriad of voluntary physical activities. Any abnormality in the musculoskeletal system decreases the efficiency of movement and increases mechanical stress. Ballistic requirements of many sports and occupations, such as skiing and driving an automobile, have increased the potential for trauma. Diseases also disrupt the integrity of the musculoskeletal system. Infectious processes, genetic abnormalities, immune-mediated inflammatory disorders, and nutritional deficiencies may all affect movement.
Clinicians who work with patients experiencing dysfunctions of the musculoskeletal system must have a solid background in evaluation and management of such disorders. Without this preparation, interventions will not be sufficient to promote maximal functional return. This chapter discusses disorders particular to the musculoskeletal system as they affect the soft tissues, skeletal frame, and muscles.
Soft-Tissue Injuries
In addition to injuries of the bony skeleton, soft tissue may also be traumatized. At times it is difficult to differentiate among the types of soft tissue. In an attempt to differentiate the exact site of a lesion, Cyriax1 described two types of soft tissue: contractile and inert. Contractile tissue is composed of structures involved in the contraction of muscle and includes not only the muscle belly but also the tendon and bony insertion, called an enthesis. Although not involved in a pure contraction, as is the muscle belly, the tendon and its insertion into bone are mechanically linked to tension generated by the muscle. Inert, or noncontractile, tissue possesses no ability to contract or relax. Inert soft tissues include joint capsules, ligaments, bursae, fasciae, dura mater, and nerve roots. Passive stretching provokes pain from inert tissue. Evaluation of inert tissue lesions requires identification of all structures involved: the capsule of a joint, a section of a ligament or a nerve, or the mechanical displacement of the meniscus.
Inert Soft-Tissue Injuries
Ligament Injuries
A ligament is a dense connective tissue with parallel-fibered collagenous tissues designed to connect bone to bone. Ligaments contribute to mechanical stability of the joint, guide motion, and prevent excessive motion. Injuries to ligaments occur when loading exceeds the physiologic range of motion. Microfailure precedes total failure of the ligament. With total failure of a ligament, damage to surrounding soft tissue occurs. Ligament injuries are classified by the extent of tear and may be described as mild, moderate, or severe.
Grade 1 (mild) = stretching injury without instability
Grade 2 (moderate) = severe injury with instability but some ligament fibers still intact
Clinical manifestations
A common site of ligament injury, particularly among athletes, is the anterior cruciate ligament of the knee (Figure 51-1). Symptoms may include a sudden “tearing” sensation or “popping” in the knee followed by pain with weight bearing and often acute swelling of the knee. Another common site for ligament injury is the anterior ankle (talofibular ligament).
Treatment
Treatment is geared primarily toward relief of symptoms, and recovery is usually complete. A moderate ligament injury is a definite tear in some component of the ligament with loss of strength. The fibers are not widely separated. Treatment is primarily protection of the ligament. With a severe ligament injury, the ligament is completely torn and no longer functions. Potentially the fragments are widely separated. Treatment may require surgical restoration of ligament continuity, when possible.
Joint Capsule Injuries
Another inert structure that is intimately involved in stabilization of a synovial joint is the joint capsule (Figure 51-2). The joint capsule is composed of an inner layer and an outer layer. The inner layer is highly vascularized but has minimal innervation. It synthesizes the hyaluronic acid component of synovial fluid, produces matrix collagen, and is essential for joint nutrition.3 The outer layer of the capsule is attached to the periosteum of the bones through Sharpey fibers. The capsule is reinforced by ligaments and musculotendinous structures. The outer layer of the capsule is poorly vascularized but richly innervated by joint receptors. Joint receptors are able to detect the rate and direction of motion, proprioception, compression and tension, vibration, and pain.3
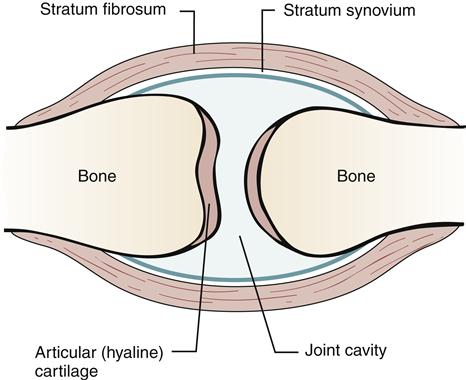
After injury to the joint capsule, the ensuing increase in vascularity and development of fibrous tissue lead to a thickening of the capsule. Any effusion into the joint cavity may lead to stretching of the capsule and its associated ligaments. The joint capsule, like ligaments, provides joint stability. The capsule, however, has an interesting mechanical adaptation: capsular redundancy. An example of the importance of the redundancy has been identified by Hettinga4: “The inferior medial portion of the shoulder joint capsule is a loose, redundant sac that becomes tense only when the shoulder is fully abducted or flexed. The posterior capsule of the knee is loose in flexion but so tight in extension that it becomes an important stabilizer.”
Capsular redundancy provides for a stable joint at the end ranges of movement. Any injury or edema in the joint that causes scarring in the lax section of the capsule prevents full range of motion. Prolonged immobilization of a joint causes loss of mobility and extensibility of the capsule, with subsequent loss of motion. Immobilization of a joint causes an alteration in the flow of synovial fluid and contracture of the joint capsule and periarticular muscle. The altered flow of synovial fluid prevents fluid diffusion into and out of cartilage and causes compression and distention of cartilage. Nutrition of the joint components stagnates, leading to degenerative changes in the joint that become permanent. Contracture of the joint capsule and periarticular muscle results when fatty tissue proliferates in the joint space. This increase in the amount of connective tissue leads to adhesions that limit joint motion. The increase in connective tissue is due to failure to keep the latticework of tissue stretched open, which usually occurs by normal flexion and extension of muscle. The muscles bridging the immobilized joint also shorten.
Adhesive Capsulitis
An example of such a restriction is loss of function in the shoulder after even a minor injury, leading to a “frozen shoulder,” also called adhesive capsulitis. With an injury to any component of the shoulder complex, inflammation occurs in the joint along with swelling and distention of the joint capsule. With prolonged immobilization, thickening of the capsule may ensue possibly attributable to proliferation of fibroblasts and capsular contraction. Capsular tightness leads to a loss of movement and an increase in pain, especially at night. Excessive joint motion may cause a tearing of the capsule, similar to a ligamentous tear, and render the joint unstable. Conservative treatment of the frozen shoulder is usually recommended with intraarticular corticosteroid injections, gentle stretching and physical therapy, and antiinflammatory medication. Prevention of adhesive capsulitis involves avoiding prolonged or excessive immobilization of the shoulder after minor injuries and performing early, gentle stretching.3
Internal Joint Derangement
Internal joint derangement may be caused by injury to inert soft-tissue structures. Meniscal tears at the knee, labrum tears at the glenohumeral joint, and disk tears in the temporomandibular joint all cause restrictions of the joint and may lead to soft-tissue dysfunction in the form of weakness, loss of motion, or pain. Tears of the medial and lateral menisci in the knee are common causes of knee pain, with the medial meniscus being torn more often. A meniscal tear is usually the result of a twisting motion.
The anterior and posterior cruciate ligaments prevent anterior and posterior displacement of the tibia relative to the femur, respectively (see Figure 51-1). These ligaments can be torn in varying degrees of severity. Injury to either ligament leads to some degree of instability in the knee joint.
Injuries to Fasciae and Bursae
Fasciae and bursae may also be causes of pain and restriction of movement of the musculoskeletal system.
Fasciae
When connective tissues of the body are arranged in sheaths that envelop muscles, they are designated fasciae. Individual muscles are surrounded by a thin fascia called the perimysium. Trauma to fascia, as with any soft tissue, may cause edema and scarring. Restrictions in fascia movement cause a restriction in joint function.
Bursae
In many locations between muscles or between muscle or tendon and bone, connective tissue forms a pocket lined with synovium that contains fluid. These pockets are identified as bursae. Bursae are located in areas of high friction and are designed to dissipate some of the stress. With faulty mechanics of the joint, repetitive movement, or direct trauma, the bursal sac may become inflamed (bursitis) and extremely painful. Bursitis, because of its strategic position at stress points of muscle function, causes major disruption of movement. An inflamed bursa may restrict any movement of the joint and lead to restriction in capsular function or muscle dysfunction as a result of edema. Some of the more common sites of bursitis include the trochanteric bursa (lateral hip), subacromial bursa (shoulder), pes anserine bursa (medial knee), and olecranon bursa (elbow).
Injuries to Nerves, Nerve Roots, or Dura Mater
Trauma to any soft tissue may lead to adhesive constriction of the nerve, nerve root, or dura mater. Irritation or entrapment of a nerve causes pain that radiates along the structures innervated by that nerve. Pain, altered sensation (numbness and tingling), motor weakness, and diminished reflexes may result from trauma to these essential soft-tissue components of the musculoskeletal system.5
An example is trauma to vertebrae in the lumbosacral area with nerve root impingement. Components of the intervertebral disk can herniate and cause pressure on nerve roots (Figure 51-3). The intervertebral disk is a shock absorber located between vertebrae. The center is a gelatinous-like material, the nucleus pulposus, which has a high water content. The nucleus pulposus is surrounded by the fibrous annulus fibrosus. Trauma to the back can cause unequal pressure on the disk leading to herniation. Common sites of disk problems are at L3 to L4, which affects the L4 nerve root; L4 to L5, which affects the L5 nerve root; and L5 to S1, which affects the S1 nerve root.
Contractile Soft-Tissue Injuries
Injury to Tendons
Injury to tendons occurs along a continuum from a minor strain, in which a few fibers of the tendon are torn, to a complete tear or rupture. The sheath in which a tendon slides may also be traumatized. Inflammation of the tendon within the sheath is called tendinitis. This inflammation may be due to infection, direct injury, or injury from repetitive motion. Tendons are injured when the stress placed on them is greater than the fibers can tolerate. Muscle tendons that are subjected to high tensile stress or compression are more prone to injury. Frequently injured tendons include the following: extensor pollicis brevis and abductor pollicis longus of the thumb (de Quervain disease), rotator cuff of the shoulder, biceps brachii tendon, tendons of the patellar complex, quadriceps tendon, hamstring tendon, Achilles tendon, and posterior tibialis tendon.
Muscle and Tendon Strains
Muscle trauma compromises the contractile unit. As in the case of injury to a tendon, tears in a muscle may range from a minor tear to complete rupture. Most injuries to muscle are due to abnormal muscle contraction. Muscle and tendon strains are often categorized by the severity of injury in a scale similar to that used for ligament injuries: mild, moderate, and severe, with the latter term applying in the setting of total rupture of the contractile structure.
Blunt Trauma
A soft-tissue contusion or crush injury also compromises the contractile structure. Any blunt trauma that causes bleeding into the muscle belly may lead to an inability to contract the muscle. Hemorrhage in a muscle belly has the potential to coagulate and calcify. This abnormal calcification in a muscle results in a painful condition called myositis ossificans. Calcification prevents a normal and strong contraction of the muscle involved.
Compartment Syndrome
Compartment syndrome is due to trauma to soft tissue caused by the unyielding structure of inert tissue. Causes of compartment syndrome may be divided into three categories: decreased compartment size, increased compartment content, or externally applied pressure. With an injury, edema causes an increase in pressure within the compartment. Because volume is expanding in a confined area, pressure reduces capillary flow. Muscle and nerves become ischemic, with a resultant excruciating pain and tissue damage. For a more detailed discussion of compartment syndrome, see the Complications of Fractures section later in this chapter.
Soft-Tissue Healing After Trauma
Trauma to soft tissue results in disruption of the circulatory and lymphatic systems. Hemorrhage, fluid loss, and cell death result. Blood vessels at the site of trauma constrict, which limits blood loss from the affected area. Norepinephrine mediates this initial constriction response, which may last a few minutes. Serotonin (from mast cells of connective tissue) and platelets prolong vasoconstriction. These processes may also contribute to vasodilation in inflamed tissue.
Platelets adhere to collagen fibers and release serotonin and adenosine diphosphate, which causes platelets to adhere to the traumatized endothelial wall and form a platelet plug that temporarily decreases bleeding. Trauma to the endothelial surface triggers release of an enzyme that initiates clotting by converting prothrombin to thrombin, which converts fibrinogen to fibrin. The endothelial surfaces of small vessels are also compressed, thereby ensuring that vessels remain closed after vasoconstriction has ceased. In the early stages of inflammation, the endothelial margins of the venules may be covered with neutrophilic leukocytes, a process called neutrophilic margination. At this point the release of histamine from mast cells, basophils, and platelets causes vasodilation and increased permeability of venules. With the increase in permeability, serous fluid containing cells and plasma proteins accumulates as edema in tissue spaces (Figure 51-4). This edema fluid contains fibrinogen, which forms fibrin through an interaction with thrombin. Fibrin seals damaged lymphatics and confines the inflammatory reaction to an area immediately surrounding the injury.
Wound Repair
The inflammatory response prepares injured tissue to progress to the healing process of repair and reorganization. Figures 51-5 and 51-6 provide a summary of the phases of wound repair. The acute response lasts about 2 weeks, and the subacute phase lasts another 2 weeks. When the wound is clear of foreign substances, an infiltrate of macrophages and fibroblasts is noted. A matrix of collagen, hyaluronic acid, and fibronectin develops. Lymphatics form in the matrix, prevent additional edema, and assist in preventing infection. This granulation tissue develops in the wound space. Macrophages have an important role in wound repair.
Next in the process of wound repair is reepithelialization of the wound surface. Epidermal cells migrate over established epidermal cells until the defect is closed. The formation of basement membrane follows. This membrane is first laid down at the wound periphery and then progresses to the center of the wound. A strong bond forms between epidermal cells and the newly formed basement membrane to complete reepithelialization (granulation tissue formation).
Wound tensile strength is a result of the deposition of collagen. Collagen production begins approximately 5 days after myofibroblast migration into the wound space. Hyaluronic acid, found in the extracellular matrix, assists glycosaminoglycans to stimulate fibroplasia. Myofibroblasts secrete an extracellular matrix, which induces cell migration and proliferation, and synthesize proteoglycans, which stimulate collagen formation and increase tissue resilience and tensile strength. By the end of the first month, tensile strength begins to increase, but several months are required to achieve the maximal level. Collagen reaches its maximal strength approximately 3 months after injury. Maximal tensile strength is only 70% to 80% of preinjury levels.
Revascularization (angiogenesis or growth of new blood vessels) must take place to ensure survival of the new tissue. Vascularization occurs through the development of new circulatory networks in the wound and reattachment of existing vessels. The formation of extracellular matrix, the development of endothelial cells, and the presence of lactic acid and heparin are a few of the factors that stimulate revascularization.
Wound closure or contraction is the final phase of healing in soft-tissue injuries. Contraction begins soon after injury and is completed in approximately 2 weeks. Myofibrils assist in wound closure. Interaction between extracellular matrix and granulation tissue results in a contractile unit called a fibronexus. Cytoplasmic actin binds to the fibronexus and draws tissue together to ensure a stable wound. As tension increases across the wound, collagen fibers are reoriented and collagen phagocytosis increases. Complete organization and concentration of collagen may require more than 40 weeks. In the case of rupture of soft-tissue structures, surgical intervention may be necessary to ensure that the traumatized tissue is in close enough proximity to allow healing.
Bone Injuries and Infections
Bone and Joint Trauma
The skeletal system is subject to alterations in function from mechanical stress and infection. The purposes of the skeletal system are to protect internal organs, contribute to mineral homeostasis, produce blood cells, and provide muscle attachment sites and thus facilitate body movement. Bone is one of the body’s hardest structures, as well as one of its most dynamic and metabolically active tissues. Bone is vascular with a capacity for repair. It adapts to mechanical demands placed on it and alters its configuration in response to those mechanical stresses.
Types of Bone
Two basic forms of bone are present in the human body: cortical bone and cancellous bone. Cortical bone forms the cortex, or outer shell, of the bone. Cortical bone is designed to tolerate compression and shearing forces, but tension forces may exceed the tolerance of cortical bone. Most fractures are due to tension failures in which bone is pulled apart. With bending, twisting, or straight tension, stress may exceed the bone’s tolerance, and a fracture occurs on the convex side of the bend. Cancellous bone, which has a spongy or lattice-like appearance, is found in the interior of bones. Unlike cortical bone, cancellous bone does not tolerate compression stress. Cancellous bone provides structural support to cortical bone and increases a bone’s potential to withstand stress.
Fracture
A fracture is a break in continuity of a bone, an epiphyseal plate, or a cartilaginous joint surface. Trauma generating enough energy to fracture a bone also produces force sufficient to traumatize adjacent soft tissue. With that concept in mind, the remainder of this section will address injury to the bony component of the musculoskeletal system.
Types of Fracture
Fracture type reflects the type of tension stress placed on bone (Figure 51-7). A transverse fracture occurs in a straight line at approximately a 90-degree angle to the longitudinal axis of the bone. Spiral fractures are the result of rotational forces and cause bone to separate in the form of an S around the bone. Longitudinal fractures split bone along its length. Oblique fractures result from a rotational force, but unlike spiral fractures, the break is along an oblique course (45-degree angle) and does not rotate around the entire bone. Comminuted fractures consist of more than one fracture line and more than two bone fragments. These fragments may be shattered or crushed. Comminuted fractures often present considerable treatment problems because of associated soft-tissue damage and multiple bone fragments. An impacted fracture is caused by excessive force that telescopes or drives one fragment into another. A greenstick fracture is an incomplete break in the bone with the intact side of the cortex flexed. It is usually seen in children. A stress fracture is a failure of one cortical surface of the bone, often caused by repetitive activity such as running. Without proper treatment, a stress fracture can become a complete fracture with two distinct fragments. An avulsion fracture is the separation of a small fragment of bone at the site of attachment of a ligament or tendon.
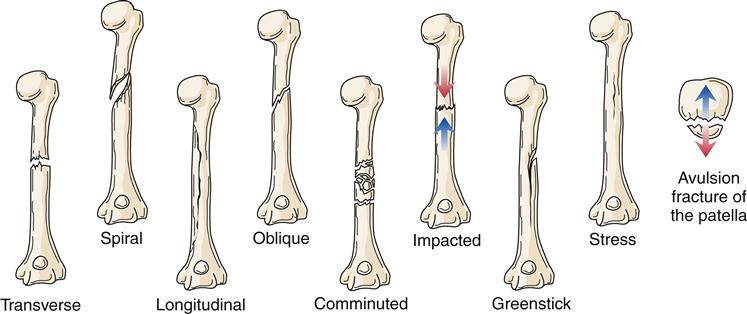
Of special concern are fractures at or near a joint line in children. This location of a fracture may suggest an epiphyseal growth plate fracture (Figure 51-8). With epiphyseal injuries, the potential for disruption of growth of the long bones is present. Proper reduction and fixation are necessary to avoid growth disturbance in fractures through the growth plate. Crush injury to the epiphyseal plate commonly leads to premature growth cessation. Cancellous bone does not tolerate compression stress; it buckles and then cracks. Therefore, crush or compression fractures (Figure 51-9) are consistent with cancellous bone trauma. In children, a compression injury to cancellous bone of the metaphysis of a long bone is identified as a buckle fracture, where bone buckles and eventually cracks. In adults, compression fractures are often found in a vertebral body of the spine, especially in older individuals with osteoporosis.
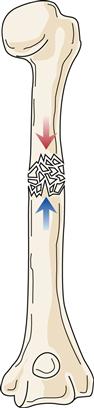
Extent of Fracture
Fractures can be classified according to extent and depth. A displaced fracture is one in which the ends of fracture fragments are separated. In a nondisplaced fracture, the fracture fragments remain in alignment and position. With a depressed fracture, the fragment is displaced below the level of the surface of the bone, usually in the skull. A complete fracture is one in which the fracture line disrupts bone continuity through the whole thickness of the bone, including the cortex (Figure 51-10). In an incomplete fracture, the cortex of the bone buckles or cracks; however, bone continuity is not disrupted. Incomplete fractures tend to occur in the more flexible, growing bones of children. Fractures can also be classified as open (compound) or closed (simple) (Figure 51-11). An open fracture occurs when bone is broken and an external wound leads to the fracture site. These fractures present an increased risk of infection and are therefore difficult to manage. A closed fracture is a fracture in which the fragments do not extend through mucous membrane or skin and skin is not broken.
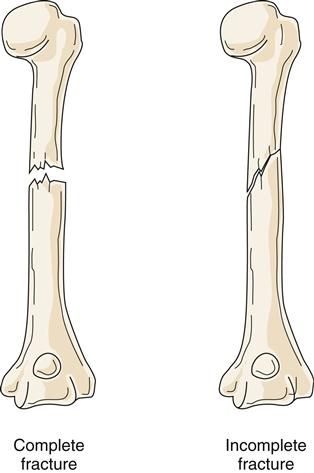
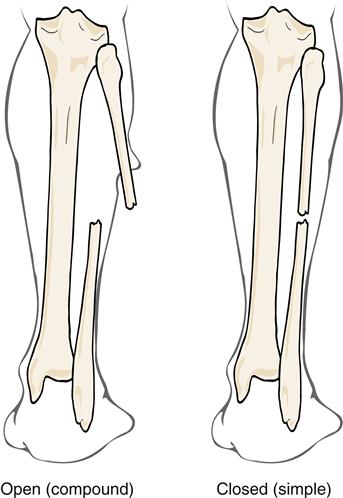
In cases of open fractures, a wound classification system may be used that ranges from type I to type IIIC in increasing degree of severity. Type I is a wound smaller than 1 cm, moderately clean with minimal contamination. The fracture is a simple transverse or an oblique fracture with a bone spike piercing the skin. Soft-tissue damage is minimal. Type II wounds are larger than 1 cm with moderate contamination. The fracture might be a moderate comminution or crush injury with moderate soft-tissue damage. Type III wounds have a high degree of contamination. The fracture is severely comminuted and unstable. It is accompanied by much soft-tissue damage involving muscle, skin, and neurovascular structures. Traumatic amputations would be classified here. With type IIIA wounds, soft-tissue coverage of the fracture is sufficient. Segmental or severely comminuted fractures occur with these wounds. Type IIIB wounds with open fractures include extensive injury or loss of soft tissue, as well as periosteal stripping and bone exposure. Fractures are severely comminuted. Massive contamination is found with such wounds. Type IIIC wounds include any open fracture associated with arterial injury requiring repair regardless of the extent of soft-tissue injury.2
Diagnosis of Fracture
In addition to a careful history and physical examination, diagnosing a fracture most often starts with plain radiographs of the skeletal area in question. It is essential to obtain views from at least two angles, preferably at 90 degrees from each other. Whenever possible, more than two views should be taken, which may increase the sensitivity of finding a fracture. Radiographs must be of optimal quality (proper exposure and angles) and include the entire bone or joint in question.
Occult nondisplaced fractures (including stress fractures) may not be seen on plain radiographs acutely. If a fracture is suspected but not easily seen, one may choose to immobilize the area as if a fracture was confirmed and then repeat the plain films in 1 to 2 weeks. Fine fractures not seen on acute films may become evident on later studies. Alternatively, computed tomography (CT) or magnetic resonance imaging (MRI) can be very useful to reveal occult fractures or nondisplaced fractures in areas not easily seen on plain films (such as the scaphoid of the wrist or the femoral neck).6
Treatment of Fracture
Initial management of a simple fracture may include icing, elevating, and immobilizing the affected limb as soon as possible to minimize soft-tissue injury. Two main goals of treatment often include fracture reduction and immobilization. Fracture reduction means restoring the limb as close to the normal anatomic position as possible. This may need to be considered emergently if there are any concerns for neurovascular compromise or potential for ischemia to the distal limb. The purpose of immobilization is to maintain the proper alignment of the reduced fracture until adequate bone healing occurs.7
Fracture immobilization may be accomplished through different means depending on the nature and severity of the fracture. For simple nondisplaced fractures, a hard cast may be the most useful. For injuries that involve considerable soft-tissue damage, splinting may be preferable over a cast because of the risk of compartment syndrome (see Complications of Fractures). Functional bracing can sometimes be used to allow joint movement while still securing the bone (such as in some types of humeral fractures).
In some circumstances, surgical intervention to reduce and immobilize the injured bone may be preferred or even required such as in cases of open fractures when debridement of the wound is needed or when multiple injuries are present. Intraarticular fractures often require surgical fixation to preserve joint motion and fractures with severely displaced bone may require surgical reduction and fixation. In other instances, when a rapid return to mobility is desired, surgical fixation may have an advantage over nonsurgical treatment.7 Internal fixation may include plates and screws attached to bone or intramedullary nails placed within long bones. External fixation utilizes fixation devices applied temporarily until an injury or the patient is stabilized and a more permanent treatment initiated. This may be needed in the setting of multiple fractures, compromised blood supply, contaminated open fractures, or extensive surrounding tissue damage.
Further treatment options may also include bone grafting to bridge wider gaps in a displaced fracture or electrical bone stimulation, which utilizes negative current applied through the use of a bone stimulator to induce bone formation.
Other treatment considerations must include indications for antibiotic prophylaxis in the setting of open fractures, tetanus prophylaxis, pain medication, and eventual rehabilitation of the soft-tissue structures surrounding the fracture.
Healing Process
When a fracture occurs, the continuity of both cortical and cancellous bone is usually compromised. The five stages of fracture healing are described in Chapter 50.
Healing in a cortical bone
At the time of fracture in a cortical bone, blood vessels in the haversian systems are torn. After a period of bleeding, clotting occurs at the fracture site and for a short distance on both sides of the fracture. Because of lack of circulation, a small section of bone distal to the fracture site undergoes necrosis (Figure 51-12). The avascular bone eventually is replaced by living bone through resorption and bone deposition. The majority of bleeding occurs from arteries in the periosteal sleeve.
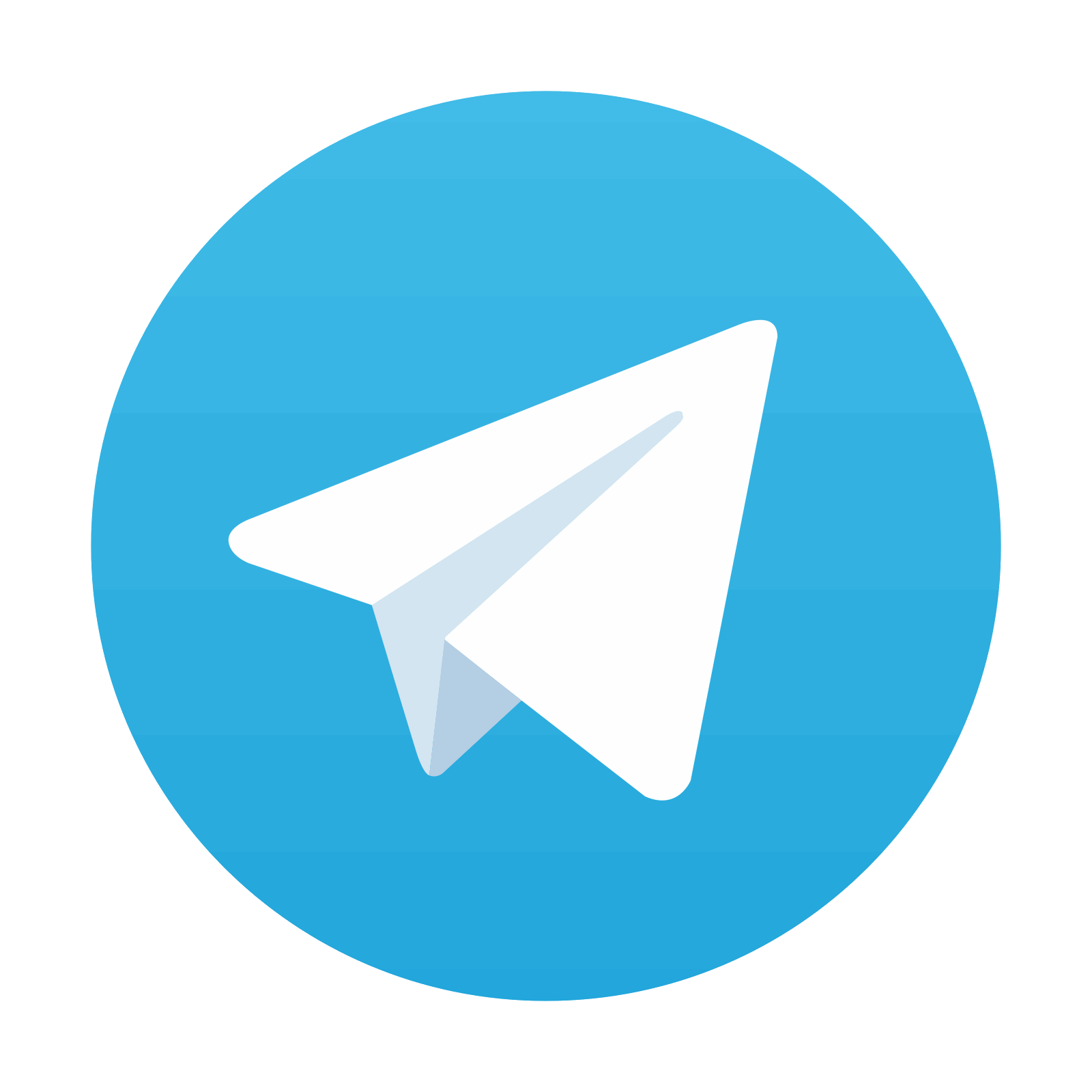
Stay updated, free articles. Join our Telegram channel

Full access? Get Clinical Tree
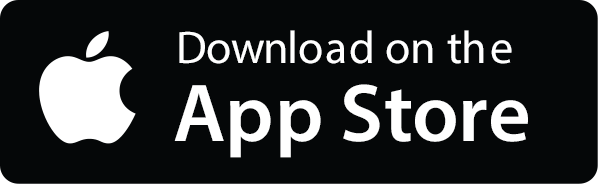
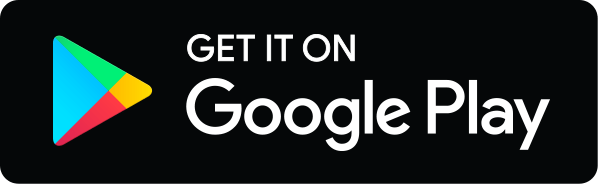