Agents Affecting Mineral Ion Homeostasis and Bone Turnover
PHYSIOLOGY OF MINERAL ION HOMEOSTASIS
CALCIUM
Elemental calcium is essential for a variety of biological functions. Its ionized form, Ca2+, is an important component of current flow across excitable membranes. Ca2+ is vital for muscle contraction, fusion, and release of storage vesicles. In the submicromolar range, intracellular Ca2+ acts as a critical second messenger (see Chapter 3). In extracellular fluid, millimolar concentrations of Ca2+ promote blood coagulation and support the formation and continuous remodeling of the skeleton.
In the face of millimolar extracellular Ca2+, intracellular free Ca2+ is maintained at a low level, ~100 nM in cells in their basal state, by active extrusion by Ca2+–ATPases, by Na+/Ca2+ exchange, and by accumulation into cellular storage networks such as the sarcoplasmic reticulum. Changes in cytosolic Ca2+ (whether released from intracellular stores or entering via membrane Ca2+ channels) can modulate effector targets, often by interacting with the Ca2+-binding protein calmodulin. The rapid association–dissociation kinetics of Ca2+ and the relatively high affinity and selectivity of Ca2+-binding domains permit effective regulation of Ca2+ over the 100 nM to 1 ~ range.
The body content of calcium in healthy adult men and women, respectively, is ~1300 and 1000 g, of which >99% is in bone and teeth. Ca2+ in extracellular fluids is stringently regulated within narrow limits. In adult humans, the normal serum Ca2+ concentration ranges from 8.5-10.4 mg/dL (4.25-5.2 mEq/L, 2.1-2.6 mM) and includes 3 distinct chemical forms of Ca2+: ionized (50%), protein-bound (40%), and complexed (10%). Thus, whereas total plasma Ca2+ concentration is ~2.5 mM, the concentration of ionized Ca2+ in plasma is ~1.2 mM. The various pools of Ca2+ are illustrated schematically in Figure 44–1. Only diffusible Ca2+ (i.e., ionized plus complexed) can cross cell membranes. Albumin accounts for some 90% of the serum Ca2+ bound to plasma proteins; a change of plasma albumin concentration of 1.0 g/dL from the normal value of 4.0 g/dL can be expected to alter total Ca2+ concentration by ~0.8 mg/dL. The remaining 10% of the serum Ca2+ is complexed with small polyvalent anions, primarily phosphate and citrate. The degree of complex formation depends on the ambient pH and the concentrations of ionized Ca2+ and complexing anions. Ionized Ca2+ is the physiologically relevant component, mediates calcium’s biological effects, and, when perturbed, produces the characteristic signs and symptoms of hypo- or hypercalcemia. The extracellular Ca2+ concentration is tightly controlled by hormones that affect calcium entry at the intestine and its exit at the kidney; when needed, these same hormones regulate withdrawal from the large skeletal reservoir.
Figure 44–1 Pools of calcium in serum. Concentrations are expressed as mg/dL on the left-hand axis and as mM on the right. The total serum calcium concentration is 10 mg/dL or 2.5 mM, divided into 3 pools: protein-bound (40%), complexed with small anions (10%), and ionized calcium (50%). The complexed and ionized pools represent the diffusable forms of calcium.
Calcium Stores. The skeleton contains 99% of total body calcium in a crystalline form resembling the mineral hydroxyapatite; other ions, including Na+, K+, Mg2+, and F–, also are present in the crystal lattice. The steady-state content of Ca2+ in bone reflects the net effect of bone resorption and bone formation.
Calcium Absorption and Excretion. In the U.S., ~75% of dietary Ca2+ is obtained from milk and dairy products. The adequate intake value for Ca2+ is 1300 mg/day in adolescents and 1000 mg/day in adults. After age 50, the adequate intake is 1200 mg/day. Figure 44–2 illustrates the components of whole-body daily Ca2+ turnover. Ca2+ enters the body only through the intestine. Active vitamin D–dependent Ca2+ transport occurs in the proximal duodenum, whereas facilitated diffusion throughout the small intestine accounts for most total Ca2+ uptake. This uptake is counterbalanced by an obligatory daily intestinal Ca2+ loss of ~150 mg/day that reflects the Ca2+ content of mucosal and biliary secretions and in sloughed intestinal cells. The efficiency of intestinal Ca2+ absorption is inversely related to calcium intake. Thus, a diet low in calcium leads to a compensatory increase in fractional absorption owing partly to activation of vitamin D. Disease states associated with steatorrhea, chronic diarrhea, or malabsorption promote fecal loss of Ca2+. Drugs such as glucocorticoids and phenytoin depress intestinal Ca2+ transport.
Figure 44–2 Whole body daily turnover of calcium. (Adapted with permission from Yanagawa N, Lee DBN. Renal handling of calcium and phosphorus. In: Coe FL, Favus MJ, eds. Disorders of Bone and Mineral Metabolism, New York: Raven Press; 1992, pp 3–40.)
Urinary Ca2+ excretion is the net difference between the quantity filtered at the glomerulus and the amount reabsorbed. About 9 g of Ca2+ are filtered each day, of which >98% is reabsorbed in the tubules. The efficiency of reabsorption is highly regulated by parathyroid hormone (PTH) and is influenced by filtered Na+, the presence of nonreabsorbed anions, and diuretic agents (see Chapter 25).
PHOSPHATE
Phosphate is present in plasma, extracellular fluid, cell membrane phospholipids, intracellular fluid, collagen, and bone tissue. More than 80% of total body phosphorus is found in bone; ~15% is in soft tissue. Additionally, phosphate is a dynamic constituent of intermediary and energy metabolism and as a key regulator of enzyme activity when transferred by protein kinases from ATP to phosphorylatable serine, threonine, and tyrosine residues. Biologically, phosphorus (P) exists in both organic and inorganic (Pi) forms. Organic forms include phospholipids and various organic esters. In extracellular fluid, the bulk of phosphorus is present as inorganic phosphate in the form of NaH2PO4 and Na2HPO4. The aggregate level of inorganic phosphate (Pi) modifies tissue concentrations of Ca2+ and plays a major role in renal H+ excretion. Within bone, phosphate is complexed with Ca2+ as hydroxyapatites and as calcium phosphate.
Absorption, Distribution, and Excretion. Phosphate is absorbed from and, to a limited extent, secreted into the GI tract. Phosphate is a ubiquitous component of ordinary foods; even an inadequate diet rarely causes phosphate depletion. Transport of phosphate from the intestinal lumen is an active, energy-dependent process that is regulated by several factors, primarily vitamin D, which stimulates absorption. In adults, about two-thirds of ingested phosphate is absorbed and is excreted almost entirely into the urine. In growing children, phosphate balance is positive, and plasma concentrations of phosphate are higher than in adults.
Phosphate excretion in the urine represents the difference between the amount filtered and that reabsorbed. More than 90% of plasma phosphate is freely filtered at the glomerulus, and 80% is actively reabsorbed, predominantly in the proximal convoluted tubule. Renal phosphate absorption is regulated by a variety of hormones and other factors; the most important are PTH and dietary phosphate, with extracellular volume and acid–base status playing lesser roles. Dietary phosphate deficiency upregulates renal phosphate transporters and decreases excretion, whereas a high-phosphate diet increases phosphate excretion; these changes are independent of any effect on plasma Pi, Ca2+, or PTH. PTH increases urinary phosphate excretion by blocking phosphate absorption. Expansion of plasma volume increases urinary phosphate excretion.
ROLE OF PHOSPHATE IN URINE ACIDIFICATION., Phosphate is concentrated progressively in the renal tubule and becomes the most abundant buffer system in the distal tubule and terminal nephron. The exchange of H+ and Na+ in the tubular urine converts Na2HPO4 to NaH2PO4, permitting the excretion of large amounts of acid without lowering the urine pH to a degree that would block H+ transport.
PHARMACOLOGICAL ACTIONS OF PHOSPHATE., Phosphate salts are employed as mild laxatives (see Chapter 46).
HORMONAL REGULATION OF CALCIUM AND PHOSPHATE HOMEOSTASIS
A number of hormones interact to regulate extracellular Ca2+ and phosphate balance. The most important are PTH and 1,25-dihydroxyvitamin D3 (calcitriol), which regulate mineral homeostasis by effects on the kidney, intestine, and bone (Figure 44–3).
Figure 44–3 Calcium homeostasis and its regulation by parathyroid hormone (PTH) and 1,25-dihydroxyvitamin D. PTH has stimulatory effects on bone and kidney, including the stimulation of 1α-hydroxylase activity in kidney mitochondria leading to the increased production of 1,25-dihydroxyvitamin D (calcitriol) from 25-hydroxycholecalciferol, the monohydroxylated vitamin D metabolite (Figure 44–5). Calcitriol is the biologically active metabolite of vitamin D.
PARATHYROID HORMONE
PTH is a polypeptide that helps to regulate plasma Ca2+ by affecting bone resorption/formation, renal Ca2+ excretion/reabsorption, and calcitriol synthesis (thus, GI Ca2+ absorption).
PTH is single polypeptide chain of 84 amino acids with molecular mass of ~9500 Da. Biological activity is associated with the N-terminal portion of the peptide; residues 1–27 are required for optimal binding to the PTH receptor and hormone activity. Derivatives lacking the first and second residue bind to PTH receptors but do not activate the cyclic AMP or IP3–Ca2+ signaling pathways. The PTH fragment lacking the first 6 amino acids inhibits PTH action.
SYNTHESIS AND SECRETION., PTH is synthesized as a 115–amino acid peptide called preproparathyroid hormone, which is converted to proparathyroid hormone by cleavage of 25 amino-terminal residues in the endoplasmic reticulum. Proparathyroid hormone is converted in the Golgi complex to PTH by cleavage of 6 amino acids. PTH(1-84) resides within secretory granules until it is discharged into the circulation. PTH(1-84) has a t1/2 in plasma of ~4 min; removal by the liver and kidney accounts for ~90% of its clearance. Proteolysis of PTH generates smaller fragments (e.g., a 33–36 amino acid N-terminal fragment that is fully active, a larger C-terminal peptide, and PTH[7-84]). PTH(7-84) and other amino-truncated PTH fragments are normally cleared from the circulation predominantly by the kidneys, whereas intact PTH is also removed by extrarenal mechanisms.
Physiological Functions., The primary function of PTH is to maintain a constant concentration of Ca2+ and Pi in the extracellular fluid. The principal processes regulated are renal Ca2+ and Pi absorption, and mobilization of bone Ca2+ (see Figure 44–3). The actions of PTH are mediated by at least 2 receptors: the PTH1 and the PTH2 receptor. Both of these are GPCRs that can couple with Gs and Gq in cell-type specific manners. PTH also can activate phospholipase D through a G12/13–RhoA pathway. A third receptor, the CPTH receptor, interacts with forms of PTH that are truncated in the amino-terminal region, contain most of the carboxy terminus, and are inactive at the PTH1 receptor; these CPTH receptors reportedly are expressed on osteocytes.
Regulation of Secretion., Plasma Ca2+ is the major factor regulating PTH secretion. As the concentration of Ca2+ diminishes, PTH secretion increases; hypocalcemia induces parathyroid hypertrophy and hyperplasia. Conversely, if the concentration of Ca2+ is high, PTH secretion decreases. Changes in plasma Ca2+ regulate PTH secretion by the plasma membrane–associated calcium-sensing receptor (CaSR) on parathyroid cells. The CaSR is a GPCR that couples with Gq and Gi. Occupancy of the CaSR by Ca2+ stimulates the Gq-PLC-IP3-Ca2+ pathway leading to activation of PKC; this results in inhibition of PTH secretion, an unusual case in which elevation of cellular Ca2+ inhibits secretion (another being the granular cells in the juxtaglomerular complex of the kidney, where elevation of cellular Ca2+ inhibits renin secretion). Simultaneous activation of the Gi pathway by Ca2+ reduces cyclic AMP synthesis and lowers the activity of PKA, also a negative signal for PTH secretion. Conversely, reduced occupancy of CaSR by Ca2+ reduces signaling through Gi and Gq, thereby promoting PTH secretion. Other agents that increase parathyroid cell cyclic AMP levels, such as β adrenergic receptor agonists and dopamine, also increase PTH secretion, but much less than does hypocalcemia. The active vitamin D metabolite, 1,25-dihydroxyvitamin D (calcitriol), directly suppresses PTH gene expression. Severe hypermagnesemia or hypomagnesemia can inhibit PTH secretion.
Effects on Bone., Chronically elevated PTH enhances bone resorption and thereby increases Ca2+ delivery to the extracellular fluid, whereas intermittent exposure to PTH promotes anabolic actions. The primary skeletal target cell for PTH is the osteoblast.
Effects on Kidney., In the kidney, PTH enhances the efficiency of Ca2+ reabsorption, inhibits tubular reabsorption of phosphate, and stimulates conversion of vitamin D to its biologically active form, 1,25-dihydroxy vitamin D3 (calcitriol; see Figure 44–3). As a result, filtered Ca2+ is avidly retained, and its concentration increases in plasma, whereas phosphate is excreted, and its plasma concentration falls. Newly synthesized 1,25-dihydroxy vitamin D3 interacts with specific high-affinity receptors in the intestine to increase the efficiency of intestinal Ca2+ absorption, thereby contributing to the increase in plasma (Ca2+).
Calcitriol Synthesis., The final step in the activation of vitamin D to calcitriol occurs in kidney proximal tubule cells. Three primary regulators govern the enzymatic activity of the 25-hydroxyvitamin D3-1 α-hydroxylase that catalyzes this step: Pi, PTH, and Ca2+ (see later for further discussion). Reduced circulating or tissue phosphate content rapidly increases calcitriol production, whereas hyperphosphatemia or hypercalcemia suppresses it. PTH powerfully stimulates calcitriol synthesis. Thus, when hypocalcemia causes a rise in PTH concentration, both the PTH-dependent lowering of circulating Pi and a more direct effect of the hormone on the 1 α-hydroxylase lead to increased circulating concentrations of calcitriol.
Integrated Regulation of Extracellular [Ca2+] by PTH., Even modest reductions of serum Ca2+ stimulate PTH secretion. With prolonged hypocalcemia, the renal 1 α-hydroxylase is induced, enhancing the synthesis and release of calcitriol that directly stimulates intestinal Ca2+ absorption (see Figure 44–3), and delivery of calcium from bone into the extracellular fluid is augmented. With prolonged and severe hypocalcemia, new bone remodeling units are activated to restore circulating Ca2+ concentrations, albeit at the expense of skeletal integrity. When plasma Ca2+ activity rises, PTH secretion is suppressed, and tubular Ca2+ reabsorption decreases. The reduction in circulating PTH promotes renal phosphate conservation, and both the decreased PTH and the increased phosphate depress calcitriol production and thereby decrease intestinal Ca2+ absorption. Finally, bone remodeling is suppressed. These integrated physiological events ensure a coherent response to positive or negative excursions of plasma Ca2+ concentrations.
VITAMIN D
Vitamin D is a hormone rather than a vitamin, and it plays an active role in Ca2+ homeostasis. The biological actions of vitamin D are mediated by the vitamin D receptor (VDR), a nuclear receptor. Vitamin D is the name applied to 2 related fat-soluble substances, vitamin D3 (cholecalciferol) and vitamin D2 (ergocalciferol) (Figure 44–4), that share the capacity to prevent or cure rickets. In humans there is no practical difference between the antirachitic potencies of vitamin D2 and vitamin D3. Therefore, “vitamin D” is used here as a collective term for vitamins D2 and D3.
Figure 44–4 Photobiology and pathways of vitamin D production and metabolism.
The principal provitamin found in animal tissues is 7-dehydrocholesterol, which is synthesized in the skin. Exposure of the skin to sunlight converts 7-dehydrocholesterol to cholecalciferol (vitamin D3). Ergosterol, present only in plants and fungi, is the provitamin for vitamin D2 (ergocalciferol). Vitamin D2 is the active constituent of a number of commercial vitamin preparations and is in irradiated bread and irradiated milk.
HUMAN REQUIREMENTS AND UNITS., Although sunlight provides adequate vitamin D supplies in the equatorial belt, in temperate climates insufficient cutaneous solar radiation, especially in winter, may necessitate dietary vitamin D supplementation. Serum levels of vitamin D vary widely, likely reflecting genetic background, diet, latitude, time spent out of doors, body size, developmental stage, and state of health, as well as plasma levels of vitamin D binding protein, a specific α-globulin. The actions of vitamin D may vary with the expression of components of the synthetic and action pathways of vitamin D. Other factors contributing to the rise of vitamin D deficiency may include diminished consumption of vitamin D–fortified foods owing to concerns about fat intake; reduced intake of dairy products; increased use of sunscreens and decreased exposure to sunlight to reduce the risk of skin cancer and prevent premature aging from exposure to ultraviolet radiation; and an increased prevalence and duration of exclusive breast-feeding (human milk is a poor source of vitamin D). There is no consensus regarding optimal vitamin D intake. The U.S. Institute of Medicine suggests achieving a serum level for 25-OH vitamin D of 50 nmol/L (20 ng/mL) and recommends a daily intake for most children and adults of 600 IU (15 µg) per day (Table 44–1).
Table 44–1
Recommended Daily Allowance of Ca2+ and Vitamin D
ADME., Vitamins D2 and D3 are absorbed from the small intestine. Bile is essential for adequate absorption of vitamin D (see Chapter 46). The primary route of vitamin D3 excretion is the bile. Patients who have intestinal bypass surgery or have or inflammation of the small intestine may fail to absorb vitamin D sufficiently to maintain normal levels; hepatic or biliary dysfunction also may seriously impair vitamin D absorption. Absorbed vitamin D circulates in the blood in association with vitamin D binding protein. The vitamin disappears from plasma with a t1/2 of 20-30 h but is stored in fat depots for prolonged periods.
METABOLIC ACTIVATION. Vitamin D requires modification to become biologically active. The primary active metabolite is 1α,25-dihydroxy vitamin D (calcitriol), the product of 2 successive hydroxylations (see Figure 44–4).
25-Hydroxylation of Vitamin D., The initial hydroxylation occurs in the liver to generate 25-OH-cholecalciferol (25-OHD, or calcifediol) and 25-OH-ergocalciferol, respectively. 25-OHD is the major circulating form of vitamin D3; it has a biological t1/2 of 19 days, and normal steady-state concentrations are 15-50 ng/mL.
1α-Hydroxylation of 25-OHD., After production in the liver, 25-OHD enters the circulation and is carried by vitamin D–binding globulin. Final activation occurs primarily in the kidney, where the enzyme 1 α-hydroxylase in the proximal tubules converts 25-OHD to calcitriol. This process is highly regulated (Figure 44–5). Calcitriol controls 1 α-hydroxylase activity by a negative-feedback mechanism that involves a direct action on the kidney, as well as inhibition of PTH secretion. The plasma t1/2 of calcitriol is estimated at 3-5 days in humans.
Figure 44–5 Regulation of 1 α-hydroxylase activity. Changes in the plasma levels of PTH, Ca2+, and phospate modulate the hydroxylation of 25-OH vitamin D to the active form, 1,25-dihydroxyvitamin D. 25-OHD, 25-hydroxycholecalciferol; 1,25-(OH)2-D, calcitriol; PTH, parathyroid hormone.
PHYSIOLOGICAL FUNCTIONS AND MECHANISM OF ACTION. Calcitriol augments absorption and retention of Ca2+ and phosphate. Calcitriol acts to maintain normal concentrations of Ca2+ and phosphate in plasma by facilitating their absorption in the small intestine, by interacting with PTH to enhance their mobilization from bone, and by decreasing their renal excretion. The actions of calcitriol are mediated by binding to cytosolic VDRs within target cells, and the receptor–hormone complex translocates to the nucleus and interacts with DNA to modify gene transcription. The VDR belongs to the steroid and thyroid hormone receptor superfamily. Calcitriol also exerts nongenomic effects.
Calcium is absorbed predominantly in the duodenum. In the absence of calcitriol, GI calcium absorption is inefficient and involves passive diffusion via a paracellular pathway. Ca2+ absorption is potently augmented by calcitriol. It is likely that calcitriol enhances all 3 steps involved in intestinal Ca2+ absorption: entry across mucosal membranes (possibly involving TRPV6 Ca2+ channels), diffusion through the enterocytes, and active extrusion across serosal plasma membranes. Calcitriol upregulates the synthesis of calbindin-D9K, calbindin-D28K, and the serosal plasma membrane Ca2+–ATPase. Calbindin-D9K enhances the extrusion of Ca2+ by the Ca2+–ATPase; the precise function of calbindin-D28K is unsettled.
The primary role of calcitriol is to stimulate intestinal absorption of Ca2+, which, in turn, indirectly promotes bone mineralization. Thus, PTH and calcitriol act independently to enhance bone resorption. Osteoblasts, the cells responsible for bone formation, express the VDR, and calcitriol induces their production of several proteins, including osteocalcin, a vitamin K–dependent protein that contains γ-carboxyglutamic acid residues, and interleukin-1 (IL-1), a lymphokine that promotes bone resorption. Thus, the current view is that calcitriol is a bone-mobilizing hormone but not a bone-forming hormone. Osteoporosis is a disease in which osteoclast responsiveness to calcitriol or other bone-resorbing agents is profoundly impaired, leading to deficient bone resorption.
Other Effects of Calcitriol., Effects of calcitriol extend well beyond calcium homeostasis. Receptors for calcitriol are distributed widely throughout the body. Calcitriol affects maturation and differentiation of mononuclear cells and influences cytokine production and immune function. Calcitriol inhibits epidermal proliferation and promotes epidermal differentiation and therefore is a potential treatment for psoriasis vulgaris (see Chapter 65).
CALCITONIN
Calcitonin is a hypocalcemic hormone whose actions generally oppose those of PTH. The thyroid parafollicular C cells produce and secrete calcitonin. Calcitonin is the most potent peptide inhibitor of osteoclast-mediated bone resorption and helps to protect the skeleton during periods of “calcium stress,” such as growth, pregnancy, and lactation.
Regulation of Secretion.
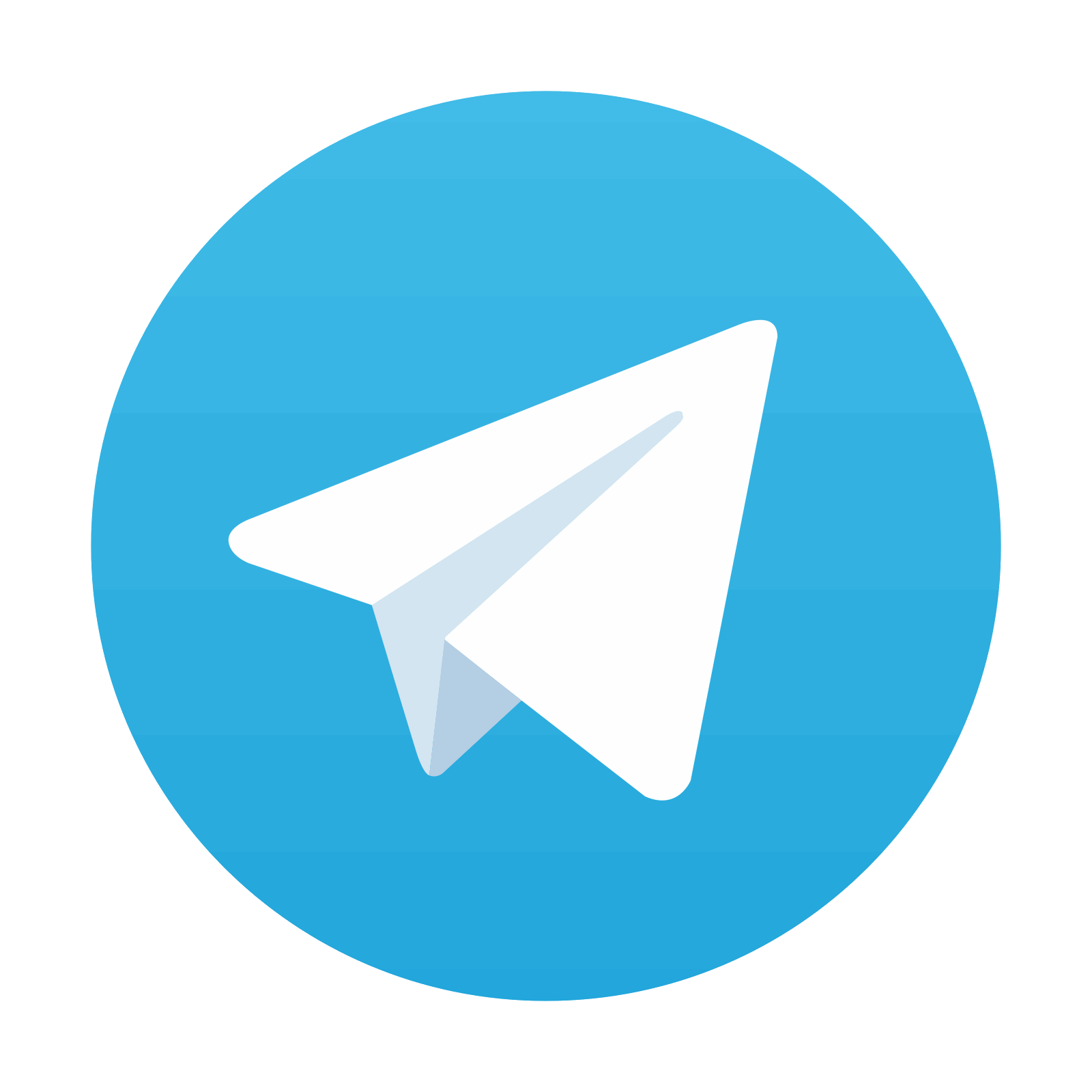
Stay updated, free articles. Join our Telegram channel

Full access? Get Clinical Tree
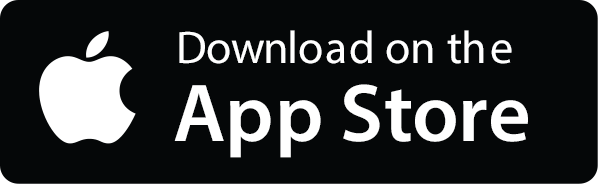
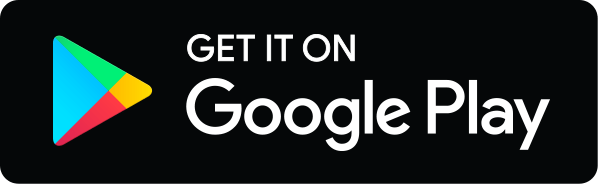