Acid-Base Balance
MECHANISMS THAT CONTROL pH OF BODY FLUIDS
Review of the pH Concept
Recall from Chapter 2 that water and all water solutions contain hydrogen ions (H+) and hydroxide ions (OH−). pH is a symbol used to represent the negative logarithm (exponent of 10) of the number of hydrogen ions (H+) present in 1 liter of a solution. It is expressed as a number between 0 and 14. In Figure 33-1 the pH value is shown on the right side of the scale and the corresponding logarithmic value is on the left. Take a moment to review the pH unit. pH indicates the degree of acidity or alkalinity of a solution. As the concentration of hydrogen ions increases, the pH goes down and the solution becomes more acid; a decrease in hydrogen ion concentration makes the solution more alkaline and the pH goes up. A pH of 7 indicates neutrality (equal amounts of H+ and OH−), a pH of less than 7 indicates acidity (more H+ than OH− and a pH greater than 7 indicates alkalinity (more OH− than H+). With a pH of about 1, gastric juice is the most acid substance in the body.
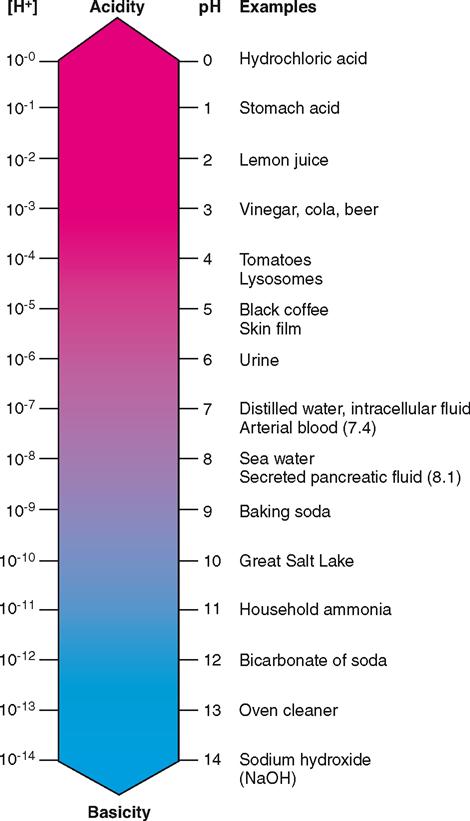
With a pH of 7.0, intracellular fluid is essentially neutral. Arterial and venous blood are slightly alkaline because both have a pH slightly higher than 7.0. The slight increase in acidity of venous blood (pH 7.36) compared with arterial blood (pH 7.40) results primarily from carbon dioxide entering venous blood as a waste product of cellular metabolism. Although any pH value above 7.0 is considered chemically basic, in clinical medicine the term acidosis is used to describe an arterial blood pH of less than 7.35 and alkalosis is used to describe an arterial blood pH greater than 7.45. The lungs remove the equivalent of more than 30 liters of carbonic acid each day from the venous blood by elimination of carbon dioxide, and yet 1 liter of venous blood contains only about 1/100,000,000 grams more hydrogen ions than does 1 liter of arterial blood. What incredible constancy! The pH homeostatic mechanism does indeed control effectively—astonishingly so.
Sources of pH-Influencing Chemicals
Acids and bases continually enter the blood as a result of absorbed foods and the metabolism of nutrients at the cellular level. Therefore, some kind of mechanism for neutralizing or eliminating these substances is necessary if blood pH is to remain constant. Although both acidic and basic components are important, the homeostasis of body pH depends largely on the control of hydrogen ion concentration in the extracellular fluid. Hydrogen ions are continually entering the body fluids from (1) carbonic acid, (2) lactic acid, (3) sulfuric acid, (4) phosphoric acid, and (5) acidic ketone bodies.
Carbonic and lactic acids are produced by the aerobic and anaerobic metabolism of glucose, respectively. Sulfuric acid is produced when sulfur-containing amino acids are oxidized, and phosphoric acid accumulates when certain phosphoproteins and ribonucleotides are broken down for energy purposes. Acidic ketone bodies, which include acetone, acetoacetic acid, and beta-hydroxybutyric acid, accumulate during the incomplete breakdown of fats. Each of these acids contributes hydrogen ions in varying amounts to the extracellular fluid and influences acid-base balance. Toxic accumulation of acidic ketone bodies is a common complication of untreated diabetes mellitus.
Minerals that remain after food has been metabolized are said to be either acid-forming minerals or base-forming minerals, depending on whether they contribute to formation of an acidic or basic medium when in solution. Acid-forming elements include chlorine, sulfur, and phosphorus—all are abundant in high-protein foods such as meat, fish, poultry, and eggs. They are also present in some grains such as wheat, corn, and oats. These foods are often designated as acid-forming foods.
After metabolism is complete, most mixed diets contain a surplus of acid-forming mineral elements that must be continually buffered to maintain acid-base balance. Extremely high-protein diets that produce a predominantly acid mineral residue when metabolized may tax the body’s ability to remain in acid-base balance if consumed over prolonged periods.
Mineral elements that are alkaline, or basic, in solution include potassium, calcium, sodium, and magnesium. All these elements are found in fruits and vegetables, which nutritionists often label as base-forming foods. The predominantly basic residue that results after metabolism of a strict vegetarian diet may also tax the ability of the body to maintain acid-base balance because of a high influx of alkaline components into the extracellular fluid.
Foods containing acids that cannot be metabolized, such as rhubarb (oxalic acid) and cranberries (benzoic acid), are said to be direct acid-forming foods, whereas antacids such as sodium bicarbonate and calcium carbonate are examples of direct base-forming substances. Box 33-1 gives some examples of acid-forming and base-forming foods.
Types of pH Control Mechanisms
The two major types of control systems listed in Table 33-1—chemical and physiological—operate to maintain the constancy of body pH.
TABLE 33-1
In the discussion that follows, buffer action is defined and the specific types of chemical and physiological buffer systems are discussed. The rapid-acting chemical buffers immediately combine with any added acid or alkali that enters the body fluids and thus prevent drastic changes in hydrogen ion concentration and pH. As explained later, all buffers act to prevent swings in pH even if hydrogen ion concentrations change. If the immediate action of chemical buffers cannot stabilize pH, the physiological buffers serve as a secondary defense against harmful shifts in pH of body fluids. pH shifts that are not halted by the immediate effects of chemical buffering cause the respiratory system to respond in 1 to 2 minutes, and changes in the rate and depth of breathing occur. For reasons explained later, such changes in carbon dioxide levels alter hydrogen ion concentration and help stabilize pH. If respiratory mechanisms are unable to stop the pH shift, a more powerful, but slower-acting, renal physiological buffer system involving the excretion of either an acid or alkaline urine will be initiated within 24 hours. Collectively, these mechanisms—buffers, respirations, and kidney excretion of acids and bases—might be said to make up the pH homeostatic mechanism. One example of the relationship between these three mechanisms is shown in Figure 33-2. The equilibrium between hydrogen ions, carbonic acid, bicarbonate, and carbon dioxide within red blood cells illustrates the interrelated nature of the pH control mechanisms. Note that an increase in body carbon dioxide levels results in excess acid formation in red blood cells, which is counteracted by a corresponding increase in elimination of both carbon dioxide by the lungs and excess hydrogen ion in the urine.
Effectiveness of pH Control Mechanisms—Range of pH
The most convincing evidence of the effectiveness of the pH control mechanism is the extremely narrow range of blood pH—normally 7.36 to 7.41. Maintaining the body’s pH within this narrow range is essential to sustain healthy life. Moving outside this pH range causes disruption of many of the body’s essential chemical processes.
BUFFER MECHANISMS FOR CONTROLLING pH OF BODY FLUIDS
Buffers Defined
In terms of action, a buffer is a substance that prevents marked changes in the pH of a solution when an acid or a base is added to it. Let us suppose that a small amount of a strong acid, hydrochloric acid, is added to a solution that contains a buffer (e.g., blood) and that its pH decreases from 7.41 to 7.27. If the same amount of hydrochloric acid were added to pure water containing no buffers, its pH would decrease much more markedly, from 7 to perhaps 3.4. In both instances, pH decreased after addition of the acid, but much less so with buffers present than without them. Stated another way, buffers do not prevent pH changes, but they do help to minimize them.
In terms of chemical composition, buffers consist of two kinds of substances and are therefore often referred to as buffer pairs.
Buffer Pairs Present in Body Fluids
Most of the body fluid buffer pairs consist of a weak acid and a salt of that acid. The main buffer pairs in body fluids are the following:
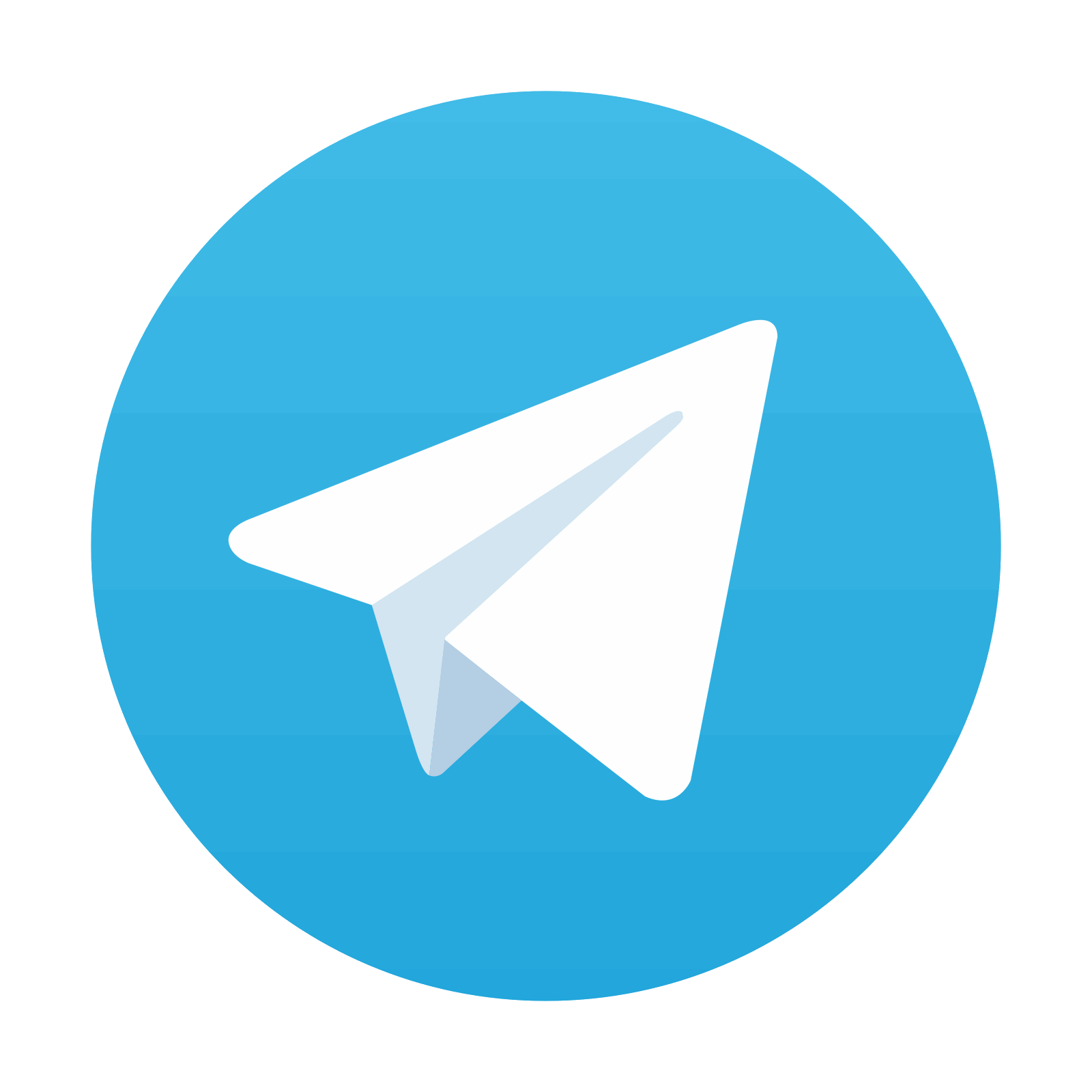
Stay updated, free articles. Join our Telegram channel

Full access? Get Clinical Tree
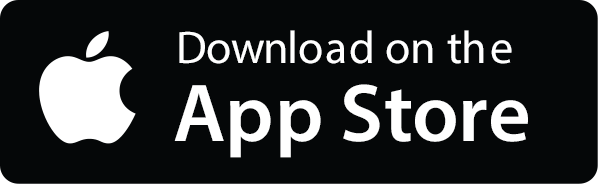
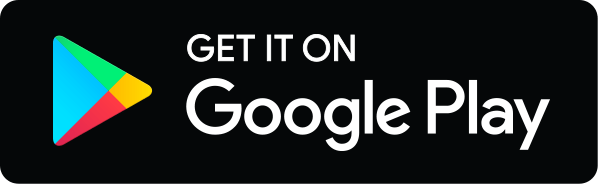