CHAPTER OUTLINE
Composition and Structure of Biological Membranes
Activities of Biological Membranes
The ABC Family of Transporters
High-Yield Terms
Lipid bilayer: a thin polar membrane made of 2 layers of lipid molecules
Integral membrane protein: a protein molecule that is permanently attached to the biological membrane
Peripheral membrane protein: a protein that adheres only temporarily to the biological membrane with which it is associated
Endoplasmic reticulum (ER): the ER functions as a packaging system working in concert with the Golgi apparatus, to create a network of membranes found throughout the whole cell
Golgi apparatus: the Golgi apparatus packages proteins inside the cell before they are sent to their destination; it is particularly important in the processing of proteins for secretion
Apical membrane: the layer of plasma membrane on the side toward the lumen of the epithelial cells
Basolateral membrane: the fraction of the plasma membrane at the basolateral side of the cell which faces adjacent cells and the underlying connective tissue
Gap junctions: intercellular channels designed for intercellular communication
Tight junctions: barriers that regulate the movement of solutes and water between various epithelial layers
Antiporter: protein or complex that couples the transport of 2 compounds across a membrane in opposite directions
Uniporter: protein or complex that transports 1 molecule of solute at a time down the solute gradient
Symporter: protein or complex that couples the transport of 2 compounds across a membrane in the same direction
Aquaporins: membrane water channels that facilitate rapid, highly selective water transport
Nar+/K+-ATPases: membrane transporters that utilize the energy of ATP hydrolysis to transport sodium into and potassium out of a cell
Cardiotonic steroids: endogenous digitalis-like factors that inhibit the Na+/K+-ATPases
ABC family transporters: ATP-binding cassette transporters that couple the energy of ATP binding and hydrolysis to substrate transport
SLC family transporters: solute carrier transporters that include transporters that function by secondary active transport and facilitative diffusion
The lipids that make up the components of membranes are of three major classes: glycerophospholipids, sphingolipids, and cholesterol.
Composition and Structure of Biological Membranes
Biological membranes are composed of lipid, protein, and carbohydrate that exist in a fluid state. Biological membranes are the structures that define and control the composition of the space that they enclose. All membranes exist as dynamic structures whose composition changes throughout the life of a cell. In addition to the outer membrane that results in the formation of a typical cell (this membrane is often referred to as the plasma membrane), cells contain intracellular membranes that serve distinct functions in the formation of the various intracellular organelles, for example, the nucleus and the mitochondria.
Sphingolipids and glycerophospholipids constitute the largest percentage of the lipid weight of biological membranes. The hydrocarbon tails of these two classes of lipid result in steric limitations to their packing such that they will form disk-like micelles. The structure of these micelles results from the interactions of the hydrophobic tails of the lipids and the exposure of the polar head groups to the aqueous environment. This orientation results in what is referred to as a lipid bilayer (Figure 7-1).
FIGURE 7-1: Diagrammatic representation of a section of a lipid bilayer composed of phospholipids. The hydrophobic fatty acids orient toward the middle of the bilayer, whereas the hydrophilic polar head groups orient toward the aqueous environment. Reproduced with permission of themedicalbiochemistrypage, LLC.
Lipid bilayers are essentially 2-dimensional fluids and the lipid components of the bilayer can diffuse laterally, and in fact evidence demonstrates that this lateral diffusion occurs readily. Lipids in the bilayer can also undergo transverse diffusion (also called a flip-flop) where the lipid diffuses from one surface to the other. However, because the flip-flop requires the polar head group to pass through the hydrocarbon core of the bilayer, the process is extremely rare. Enzymes have been identified that facilitate the flip-flop process and these enzymes are referred to as flippases.
The carbohydrates of membranes are attached either to lipid, forming glycolipids of various classes, or to proteins forming glycoproteins. The lipid and protein compositions of membranes vary from cell type to cell type as well as within the various intracellular compartments that are defined by intracellular membranes. Protein concentrations can range from around 20% to as much as 70% of the total mass of a particular membrane.
Biological membranes also contain proteins, glycoproteins, and glycolipids.
High-Yield Concept
Proteins associated with membranes are of 2 general types: (1) integral and (2) peripheral (Figure 7-2). Integral membrane proteins (also called intrinsic proteins) are tightly bound to the membrane through hydrophobic interactions and are inserted into and/or penetrate the lipid bilayer. In contrast, peripheral membrane proteins (also called extrinsic proteins) are only loosely associated with the membrane either through interactions with the polar head groups of the lipids or through interactions with integral membrane proteins.
FIGURE 7-2: Structure of the typical lipid bilayer of the plasma membrane. Integral proteins are those that pass through the bilayer. Peripheral proteins are associated with the inner surface of the plasma membrane. Most integral proteins are modified by carbohydrate addition to their extracellular domains. Membranes also contain carbohydrate-modified lipids (glycolipids) in addition to the more common phospholipids and cholesterol that constitute the bulk of the lipid content of the membrane. Reproduced with permission of themedicalbiochemistrypage, LLC.
Peripheral membrane proteins are most often, if not exclusively, found on the cytosolic face of the plasma membrane or the luminal surface of subcellular organelle membranes.
Proteins that are found associated with membranes can also be modified by lipid attachment (lipoproteins). The lipid portion of a lipoprotein anchors the protein to the membrane either through interaction with the lipid bilayer directly or through interactions with integral membrane proteins. Lipoproteins associated with membranes contain 1 of 3 types of covalent lipid attachment. The lipids are isoprenoids such as farnesyl and geranylgeranyl residues (see Chapter 37 for the mechanism of protein prenylation), fatty acids such as myristic and palmitic acid, and glycosylphosphatidylinositol, GPI (termed glipiated proteins) (see Chapter 38 for details).
Activities of Biological Membranes
Although biological membranes contain various types of lipids and proteins, their distribution between the two different sides of the bilayer is asymmetric. As a general example, the outer surface of the bilayer is enriched in phosphatidylethanolamine (PE), whereas the intracellular surface is enriched in phosphatidylcholine (PC). Carbohydrates, whether attached to lipid or protein, are almost exclusively found on the external surfaces of membranes. The asymmetric distribution of lipids and proteins in membranes results in the generation of highly specialized subdomains within membranes. In addition, there are highly specialized membrane structures such as the endoplasmic reticulum (ER), the Golgi apparatus, and vesicles (eg, endosomes and lysosomes). The most important vesicles are those that contain secreted factors. Membrane-bound proteins (eg, growth factor receptors) are processed as they transit through the ER to the Golgi apparatus and finally to the plasma membrane. As these proteins transit to the surface of the cell, they undergo a series of processing events that include glycosylation.
The vesicles that pinch off from the Golgi apparatus are termed coated vesicles. The membranes of coated vesicles are surrounded by specialized scaffolding proteins that will interact with the extracellular environment. There are 3 major types of coated vesicles that are characterized by their protein coats.
Clathrin-coated vesicles contain the protein clathrin and are involved in transmembrane protein, GPI-linked protein, and secreted protein transit to the plasma membrane.
High-Yield Concept
The membrane surface of cells that interacts with luminal contents is referred to as the apical surface or domain, the rest of the membrane is referred to as the basolateral surface or domain. The apical and basolateral domains do not intermix and contain different compositions of lipid and protein.
COPI (COP = coat protein) forms the surface of vesicles involved in the transfer of proteins between successive Golgi compartments. COPII forms the surface of vesicles that transfer proteins from the ER to the Golgi apparatus. Clathrin-coated vesicles are also involved in the process of endocytosis such as occurs when the LDL (low-density lipoprotein) receptor binds plasma LDL for uptake by the liver. The membrane location of these types of receptors is called a clathrin-coated pit.
In addition, certain cells have membrane compositions that are unique to one surface of the cell versus the other. For instance, epithelial cells have a membrane surface that interacts with the luminal cavity of the organ and another that interacts with the surrounding cells.
Most eukaryotic cells are in contact with their neighboring cells and these interactions are the basis of the formation of organs. Cells that are touching one another are in metabolic contact which is brought about by specialized tubular particles called junctions.
Gap junctions are intercellular channels designed for intercellular communication and their presence allows whole organs to be continuous from within. One major function of gap junctions is to ensure a supply of nutrients to cells of an organ that are not in direct contact with the blood supply. Gap junctions are formed from a type of protein called a connexin (Figure 7-3). Tight junctions are primarily found in the epithelia and are designed for occlusion. Tight junctions act as barriers that regulate the movement of solutes and water between various epithelial layers. At least 40 proteins have been found to be involved in the formation of the various tight junctions. These proteins are divided into 4 major categories: (1) scaffolding, (2) regulatory, (3) transmembrane, and (4) signaling. Adherens junctions are composed of transmembrane proteins that serve to anchor cells via interactions with the extracellular matrix. The proteins of adherens junctions are members of the various cadherin and integrin protein families. Related to the adherens junctions are the desmosomes and hemidesmosomes that are also involved in membrane-anchoring functions.
FIGURE 7-3: Schematic diagram of a gap junction. One connexon is made from 2 hemiconnexons. Each hemiconnexon is made from 6 connexin molecules. Small solutes are able to diffuse through the central channel, providing a direct mechanism of cell–cell communication. Murray RK, Bender DA, Botham KM, Kennelly PJ, Rodwell VW, Weil PA. Harper’s Illustrated Biochemistry, 29th ed. New York, NY: McGraw-Hill; 2012.
Given the predominant lipid nature of biological membranes, many types of molecules are restricted in their ability to diffuse across a membrane. This is especially true for charged ions, water, and hydrophilic compounds. The barrier to membrane translocation is overcome by the presence of specialized channels and transporters. Although channels and transporters are required to move many types of molecules and compounds across membranes, some substances can pass through from one side of a membrane to the other through a process of diffusion. Diffusion of gases such as O2, CO2, NO, and CO occurs at a rate that is solely dependent upon concentration gradients. Lipophilic molecules will also diffuse across membranes at a rate that is directly proportional to the solubility of the compound in the membrane.
High-Yield Concept
Mammalian cells contain three major types of cell junctions called gap junctions, tight junctions, and adherens junctions.
Membrane Channels
The definition of a membrane channel (also called a pore) is that of a protein structure that facilitates the translocation of molecules or ions across the membrane through the creation of a central aqueous channel in the protein. Channel proteins do not bind or sequester the molecule or ion that is moving through the channel. Membrane channels facilitate diffusion in both directions dependent upon the direction of the concentration gradient. Specificity of channels for ions or molecules is a function of the size and charge of the substance. The flow of molecules through a channel can be regulated by various mechanisms that result in opening or closing of the passageway.
Membrane channels are of 3 distinct types: (1) α-type channels, (2) β-barrel channels, and (3) the pore-forming toxins. The α-type channels are homo- or hetero-oligomeric structures that in the latter case consist of several different proteins. The α-type class of channel protein has between 2 and 22 transmembrane α-helical domains, thus the derivation of the name of this type of channel. Molecules move through α-type channels down their concentration gradients and thus require no input of metabolic energy. The transport of molecules through α-type channels occurs by several different mechanisms. These mechanisms include changes in membrane potential (termed voltage-regulated or voltage-gated), phosphorylation of the channel protein, intracellular Ca2+, G-proteins, and organic modulators. Some channels of this class are highly specific with respect to the molecule that moves across the membrane while others are not. In addition, there may be differences from tissue to tissue in the channel used to transport the same molecule. As an example, there are over 15 different K+-specific voltage-regulated channels in humans.
The aquaporins assemble in the membrane as homotetramers with each monomer consisting of 6 transmembrane α-helical domains forming the distinct water pore. Probably the most significant location of aquaporin expression is in the kidney. Loss of function of the renal aquaporins is associated with several disease states such as nephrogenic diabetes insipidus (NDI), acquired hypokalemia, and hypercalcemia (Figure 7-4).
FIGURE 7-4: Diagrammatic representation of the structure of an aquaporin. The pores that form in the aquaporins are composed of 2 halves referred to as hemipores. Amino acids of the pore that are critical for water transport are the asparagine (N), proline (P), and alanine (A) residues indicated in each hemipore. Reproduced with permission of themedicalbiochemistrypage, LLC.
The β-barrel channels (also called porins) are so named because they have a transmembrane domain that consists of β-strands forming a β-barrel structure. Porins are found in the outer membranes of mitochondria. The mitochondrial porins are voltage-gated anion channels that are involved in mitochondrial homeostasis and apoptosis.
The pore-forming toxins family of channels is a large class of proteins first identified in bacteria. Subsequently several proteins of this class were identified in mammalian cells. The defensins are a family of small cysteine-rich antibiotic proteins that are pore-forming channels found in epithelial and hematopoietic cells. The defensins are involved in host defense against microbes (hence the derivation of their name) and may be involved in endocrine regulation during infection.
High-Yield Concept
Aquaporins (AQP) are a family of α-type channels responsible for the transport of water across membranes.
High-Yield Concept
Uniporters transport a single molecule at a time, symporters simultaneously transport 2 different molecules in the same direction, and antiporters transport 2 different molecules in opposite directions.
The action of transporters is divided into 2 classifications: (1) passive-mediated transport (also called facilitated diffusion) and (2) active transport.
Membrane Transporters
Transporters are distinguished from channels because they catalyze (mediate) the movement of ions and molecules by physically binding to and moving the substance across the membrane. Transporter activity can be measured by the same kinetic parameters applied to the study of enzyme kinetics. Transporters exhibit specificity for the molecule being transported as well as show defined kinetics in the transport process. Transporters can also be affected by both competitive and noncompetitive inhibitors. Transporters are also known as carriers, permeases, translocators, translocases, and porters. Mediated transporters are classified based upon the stoichiometry of the transport process (Figure 7-5). Defects in many transporter-encoding genes are associated with the potential for severe clinical manifestiation (see Clinical Box 7-1).
FIGURE 7-5: Representations of the various mechanisms for the passage/transport of ions and molecules across biological membranes. Reproduced with permission of themedicalbiochemistrypage, LLC.
CLINICAL BOX 7-1: TRANSPORTER DEFECTS AND DISEASE
Defects in the expression and/or function of membrane transporters have been associated with clinically significant diseases. Whereas, the total number is too numerous to mention, several important disorders are presented.
ABCA1 is involved in the transport of cholesterol out of cells when HDLs are bound to their cell surface receptor, SR-B1 (see Chapter 28). One important consequence of the activity of ABCA1 in macrophages is that the efflux of cholesterol results in a suppression of inflammatory responses triggered by macrophages that have become foam cells due to cholesterol uptake. Defects in ABCA1 result in Tangier disease which is characterized by 2 clinical hallmarks: (1) enlarged lipid-laden tonsils and (2) low serum HDL.
ABCG5 and ABCG8 form an obligate heterodimer pair that functions to limit plant sterol and cholesterol uptake by the gut and mediate cholesterol efflux from the liver into the bile (see Chapter 25). Mutations in either ABCG5 or ABCG8 result in a rare genetic disorder identified as sitosterolemia (also called phytosterolemia). This disorder is characterized by unrestricted absorption of plant sterols (such as sitosterol) and cholesterol. Individuals afflicted with this disorder manifest with very high levels of plant sterols in the plasma and develop tendon and tuberous xanthomas, accelerated atherosclerosis, and premature coronary artery disease.
ABCB11 is also known as bile salt export protein (BSEP) which is involved in bile salt transport out of hepatocytes into the bile canaliculi (see Chapter 25). Defects in this gene are associated with progressive familial intrahepatic cholestasis type 2 (PFIC2). The symptoms of PFIC2 usually present in early infancy as failure to thrive, jaundice, and severe pruritus (unpleasant sensation eliciting a desire to scratch). The symptoms of PFIC2 progress continuously, leading ultimately to liver failure and the need for liver transplantation in order to survive.
ABCC2 was first identified as the canalicular multispecific organic anion transporter (CMOAT) and is also called multidrug-resistance-associated protein 2 (MRP2). Defects in the gene encoding ABCC2 result in Dubin-Johnson syndrome (see Chapter 33), which is a form of conjugated hyperbilirubinemia.
ABCD1 is involved in the import and/or anchoring of very-long-chain fatty acid-CoA synthetase (VLCFA-CoA synthetase) to the peroxisomes. Defects in the gene result in X-linked adrenoleukodystrophy (XALD).
ATP7A and ATP7B are copper-transporting ATPases that are related to SLC31A1. Defects in ATP7A result in Menkes disease and defects in ATP7B are associated with Wilson disease (see Chapter 42).
SLC6A19 is also called system B(0) neutral amino acid transporter 1 [B(0)AT1]. This transporter is involved in neutral amino acid transport with highest levels of expression in the kidney and small intestine. Deficiency in SLC6A19 leads to Hartnup disease (see Clinical Box 30-1), which results from impaired transport of neutral amino acids across epithelial cells in renal proximal tubules and intestinal mucosa. Symptoms include transient manifestations of pellagra-like light-sensitive rash, cerebellar ataxia, and psychosis.
SLC35C1 is also known as the GDP-fucose transporter (gene symbol = FUCT1). Defects in the FUCT1 gene result in a congenital disorder of glycosylation (CDG) (see Chapter 38). Specifically the disorder is a type II CDG identified as CDGIIc. Type II CDGs result from defects in the processing of the carbohydrate structures on N-linked glycoproteins. CDGIIc is also called leukocyte adhesion deficiency syndrome II (LAD II). LAD II is a primary immunodeficiency syndrome which manifests due to leukocyte dysfunction. Symptoms of LAD II include unique facial features, recurrent infections, persistent leukocytosis, defective neutrophil chemotaxis, and severe growth and mental retardation.
SLC40A1 is a multiple transmembrane-spanning protein involved in iron transport. The protein is highly expressed in the intestine, liver, and reticuloendothelial cells. SLC40A1 is more commonly known as ferroportin or insulin-regulated gene 1 (IREG1). The protein is required for the transport of dietary iron across the basolateral membranes of intestinal enterocytes. Defects in the SLC40A1 gene are associated with type 4 hemochromatosis (see Chapter 42).
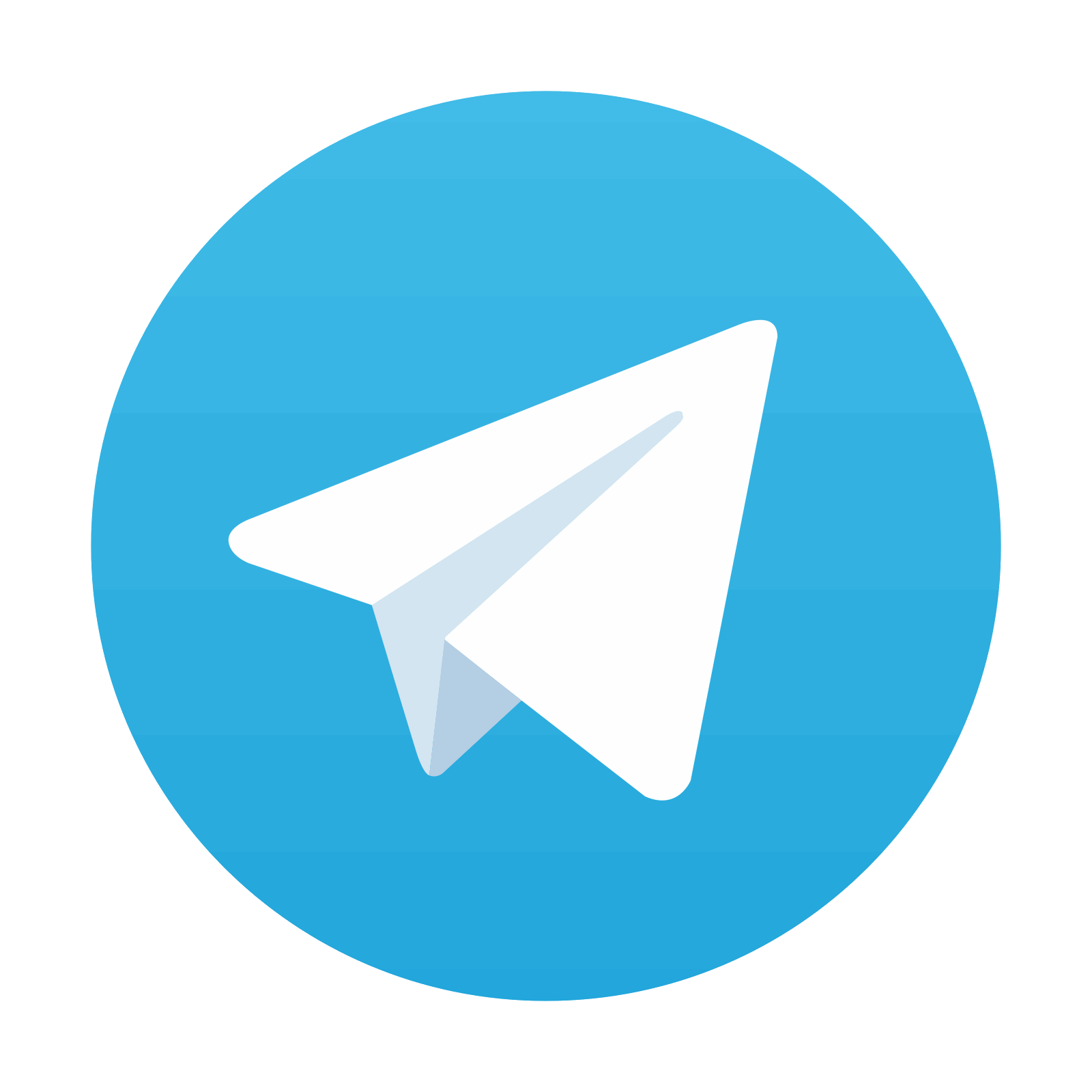
Stay updated, free articles. Join our Telegram channel

Full access? Get Clinical Tree
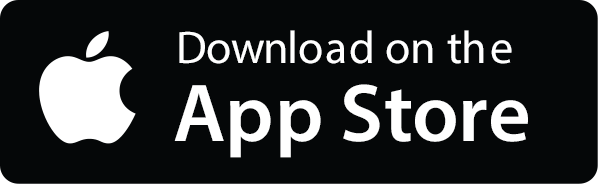
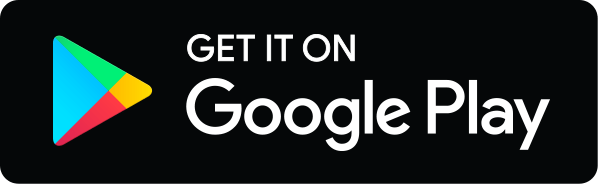