CHAPTER OUTLINE
Mechanisms of Signal Transduction
Classifications of Signal-Transducing Receptors
Nonreceptor Protein Tyrosine Kinases
Receptor Serine/Threonine Kinases
Nonreceptor Serine/Threonine Kinases
Intracellular Hormone Receptors
Phospholipases and Phospholipids in Signal Transduction
Phosphatases in Signal Transduction
High-Yield Terms
Signal transduction: refers to the movement of signals from outside the cell to inside, resulting in a change in the “state” of the cell
Growth factor: any of a family of proteins that bind to receptors with the primary result of activating cellular proliferation and/or differentiation
Cytokine: any of unique family of growth factor proteins primarily secreted from leukocytes
Chemokine: a subfamily of cytokines (chemotactic cytokines) that is capable of inducing chemotaxis
Interleukin: any of a family of multifunctional cytokines that are produced by a variety of lymphoid and nonlymphoid cells
Serpentine receptors: any of a family of G-protein–coupled receptors (GPCRs) so called because they span the plasma membrane 7 times
G-protein: any of a large family of proteins that bind and hydrolyze GTP in the act of transmitting signals, includes the heterotrimeric and the monomeric G-protein families
Nuclear receptors: intracellular receptors that bind lipophilic ligands and then bind to specific DNA sequences in target genes regulating their expression
Mechanisms of Signal Transduction
Signal transduction at the cellular level refers to the movement of signals from outside the cell to inside. The movement of signals can be simple, like that associated with receptor molecules of the acetylcholine class: receptors that constitute channels which, upon ligand interaction, allow signals to be passed in the form of small ion movement, either into or out of the cell. These ion movements result in changes in the electrical potential of the cells that, in turn, propagates the signal along the cell. More complex signal transduction involves the coupling of ligand-receptor interactions to many intracellular events. These events include phosphorylations by tyrosine kinases and/or serine/threonine kinases. Protein phosphorylations change enzyme activities and protein conformations. The eventual outcome is an alteration in cellular activity and changes in the program of genes expressed within the responding cells.
Growth Factors
Growth factors are classified as substances that bind to receptors with the primary result of activating cellular proliferation and/or differentiation. The vast majority of growth factors are proteins that bind to extracellular ligand-binding domains of transmembrane receptors (Table 40-1). However, some steroid hormones have growth factor activity. Steroid hormones bind intracellular receptors (see later). Many growth factors are quite versatile, stimulating cellular division in numerous different cell types; while others are specific to a particular cell type. Individual growth factor proteins tend to occur as members of larger families of structurally and evolutionarily related proteins. For example, there are at least 18 members of the fibroblast growth factor (FGF) family of proteins.
High-Yield Concept
Cytokines that are secreted from lymphocytes are termed lymphokines, whereas those secreted by monocytes or macrophages are termed monokines. A large family of cytokines is produced by various cells of the body. Many of the lymphokines are more commonly known as interleukins (ILs), since they are not only secreted by leukocytes but are also able to affect the cellular responses of leukocytes.
Cytokines and Chemokines
Cytokines are a unique family of growth factors (Table 40-2). Secreted primarily from leukocytes, cytokines stimulate both the humoral and cellular immune responses, as well as the activation of phagocytic cells.
Specifically, interleukins are growth factors targeted to the cells of hematopoietic origin. Several growth factors are also considered members of the cytokine family, such as the members of the colony-stimulating factor (CSF) family of proteins that stimulate the proliferation of specific pluripotent stem cells of the bone marrow, and TNF-α and some members of the large TGF-β family.
Proteins are classified as chemokines according to shared structural characteristics such as small size and the presence of 4 cysteine residues in conserved locations that are key to forming their 3-dimensional shape. The nomenclature for cytokines and chemokines is a mix of the widely accepted, but slightly misleading, designations. For example, interleukin-8 (IL-8) is actually a chemokine but the interleukin name remains in common usage.
High-Yield Concept
Chemokines represent a subfamily of cytokines whose name is derived from chemotactic cytokines. These proteins are capable of inducing chemotaxis in nearby responsive cells.
Like the protein family of growth factors, cytokines and chemokines elicit their effects through interaction with the ligand-binding domain of transmembrane receptors.
Classifications of Signal-Transducing Receptors
Signal-transducing receptors are of 3 general classes:
1. Receptors that penetrate the plasma membrane and have intrinsic enzymatic activity. Receptors that have intrinsic enzymatic activities include those that are tyrosine kinases (eg, PDGF, insulin, EGF, and FGF receptors), tyrosine phosphatases (eg, CD45 [cluster determinant-45] protein of T cells and macrophages), guanylate cyclases (eg, natriuretic peptide receptors), and serine/threonine kinases (eg, activin and TGF-β receptors). Receptors with intrinsic tyrosine kinase activity are capable of autophosphorylation as well as phosphorylation of other substrates. Additionally, several families of receptors lack intrinsic enzyme activity, yet are coupled to intracellular tyrosine kinases by direct protein-protein interactions.
2. Receptors that are coupled, inside the cell, to GTP-binding and hydrolyzing proteins (termed G-proteins). Receptors of the class that interact with G-proteins have a structure that is characterized by 7 transmembrane spanning domains. These receptors are termed serpentine receptors. Examples of this class are the adrenergic receptors, odorant receptors, and certain hormone receptors (eg, glucagon, angiotensin, vasopressin, and bradykinin).
3. Receptors that are found intracellularly and upon ligand binding migrate to the nucleus, where the ligand-receptor complex directly affects gene transcription. Because this class of receptors is intracellular and functions in the nucleus as transcription factors, they are commonly referred to as the nuclear receptors. Receptors of this class include the large family of steroid and thyroid hormone receptors. Receptors in this class have a ligand-binding domain, a DNA-binding domain, and a transcriptional regulatory domain.
Receptor Tyrosine Kinases
The receptor tyrosine kinase (RTK) family of transmembrane ligand-binding proteins is comprised of 59 members in the human genome. Each of the RTKs exhibit similar structural and functional characteristics. Most RTKs are monomers and their domain structure includes an extracellular ligand-binding domain, a transmembrane domain, and an intracellular domain possessing the tyrosine kinase activity (Figure 40-1). The insulin and insulin-like growth factor receptors are the most complex in the RTK family, being disulfide-linked heterotetramers.
FIGURE 40-1: A diagrammatic representation of several members of the receptor tyrosine kinase (RTK) family. Several members of each receptor subfamily are indicated below each representative. The Roman numerals above the first 7 subtypes correspond to those subtypes described in Table 40-3. These RTK subtypes do not represent the entire RTK family. Reproduced with permission of themedicalbiochemistrypage, LLC.
The amino acid sequences of the tyrosine kinase domains of RTKs are highly conserved with those of cAMP-dependent protein kinase (PKA) within the ATP-binding and substrate-binding regions. RTK proteins are classified into families based upon structural features in their extracellular portions, as well as the presence or absence of a kinase domain insert. The extracellular domains include the cysteine-rich domains, immunoglobulin-like domains, leucine-rich domains, Kringle domains, cadherin domains, fibronectin type III repeats, discoidin I–like domains, acidic domains, and EGF-like domains. Based upon the presence of these various extracellular domains, the RTKs have been subdivided into at least 20 different families (Table 40-3).
Many receptors that have intrinsic tyrosine kinase activity as well as the tyrosine kinases that are associated with cell surface receptors contain tyrosine residues that, upon phosphorylation, interact with other proteins of the signaling cascade. These other proteins contain a domain of amino acid sequences that are homologous to a domain first identified in the kinase encoded by the SRC proto-oncogene. These domains are termed SH2 domains (SRC homology domain 2). Another conserved protein-protein interaction domain identified in many signal-transduction proteins is related to a third domain in SRC identified as the SH3 domain.
The interactions of SH2 domain–containing proteins with RTKs, or receptor-associated tyrosine kinases, leads to tyrosine phosphorylation of the SH2-containing proteins. Phosphorylation of SH2-containing proteins that have enzymatic activity results in an alteration (either positively or negatively) in that activity. Several SH2-containing proteins that have intrinsic enzymatic activity include phospholipase C-γ (PLC-γ), the proto-oncogene RAS-associated GTPase-activating protein (rasGAP), phosphatidylinositol-3-kinase (PI3K), protein phosphatase-1C (PTP1C), as well as members of the SRC family of protein tyrosine kinases (PTKs).
Nonreceptor Protein Tyrosine Kinases
There are numerous intracellular protein tyrosine kinases (PTKs) that are responsible for phosphorylating a variety of intracellular proteins on tyrosine residues following activation of cellular growth and proliferation signals. There are 2 distinct families of nonreceptor PTKs. The archetypal PTK family is related to the SRC protein. The SRC protein is a tyrosine kinase first identified as the transforming protein in Rous sarcoma virus. The second family is related to the Janus kinase (JAK). Most proteins in these 2 families couple to transmembrane receptors that do not themselves have intrinsic enzymatic activity. This class of receptors includes all of the cytokine receptors.
Receptor Serine/Threonine Kinases
The receptors for the TGF-β superfamily of ligands have intrinsic serine/threonine kinase (RSK) activity. There are more than 30 multifunctional proteins of the TGF-β superfamily, which also includes the activins, inhibins, and the bone morphogenetic proteins (BMPs). This superfamily of proteins can induce and/or inhibit cellular proliferation or differentiation and regulate migration and adhesion of various cell types.
At least 17 RSTKs have been isolated and can be divided into 2 subfamilies identified as the type I and type II receptors. Ligand first binds to a type II receptor, which then leads to interaction with a type I receptor. When the complex between ligand and the 2 receptor subtypes forms, the type II receptor phosphorylates the type I receptor, leading to initiation of the signaling cascade.
Nonreceptor Serine/Threonine Kinases
There are more than 500 different types of kinases in the human genome and at least 125 of those are members of the serine/threonine kinase family, many of which are nonreceptor serine/threonine kinases (STKs). The 2 most commonly encountered STKs in the context of metabolic biochemistry are cAMP-dependent protein kinase (PKA) and protein kinase C (PKC). Additional serine/threonine kinases important for signal transduction are the MAP kinases, whose designation refers to mitogen-activated protein kinase. MAP kinases are also called ERKs for extracellular signal-regulated kinases.
G-Proteins
G-proteins are so called because their activities are regulated by binding and hydrolyzing GTP. When a G-protein is bound to GTP, it is in the active state and when the GTP is hydrolyzed to GDP the protein is in the inactive state.
There are 2 major classes of G-protein: the heterotrimeric (or simply the trimeric) family that are composed of 3 distinct subunits (α, β, and γ), and the monomeric class that are related to the archetypal member Ras (originally identified as the rat sarcoma oncogene). This latter class of G-protein is also referred to as the Ras superfamily or the small GTPase family of G-proteins. The structure and function of the monomeric G-proteins is similar to that of the α-subunit of the trimeric G-proteins.
High-Yield Concept
The G-proteins possess intrinsic GTPase activity that is regulated by interaction with membrane-associated signal-transducing receptors (termed G-protein–coupled receptors, GPCRs) or with intracellular effector proteins.
Trimeric G-Proteins
All the known cell surface receptors that are of the G-protein–coupled receptor class interact with trimeric G-proteins. The α-subunit of the trimeric class of G-proteins is responsible for the binding of GDP/GTP. When G-proteins are activated by receptors or intracellular effector proteins, there is an exchange of GDP for GTP turning on the G-protein which enables it to transmit the original activating signal to downstream effector proteins. In the trimeric class of G-protein, when associated receptor activation stimulates the GDP/GTP exchange in the α-subunit, the protein complex dissociates into separate α- and βg-activated complexes (Figure 40-2). The released and activated βg-complex serves as a docking site for interaction with downstream effectors of the signal-transduction cascade. Once the α-subunit hydrolyzes the bound GTP to GDP, it reassociates with the βγ complex thereby terminating its activity.
FIGURE 40-2: A diagrammatic representation of the activation of trimeric G-proteins upon ligand binding to typical G-protein–coupled receptors. Upon ligand binding to a GPCR, there is an activated exchange of GDP bound to the α-subunit for GTP catalyzed by an associated guanine nucleotide exchange factor (GEF). The resultant GTP-associated α-subunit can then activate downstream effector proteins. In some cases, G-protein βγ-subunits also regulate the activity of downstream effectors. Hydrolysis of GTP to GDP during the G-protein activation of effectors as a result of the action of GTPase-activating proteins (GAPs) results in termination of the activity of the α-subunit. Reproduced with permission of themedicalbiochemistrypage, LLC.
The GTPase activity of G-proteins is augmented by GTPase-activating proteins (GAPs) and the GDP/GTP exchange reaction is catalyzed by guanine nucleotide exchange factors (GEFs). Within the small GTPase family (Ras family) of G-proteins there are guanosine nucleotide dissociation inhibitors (GDIs) that maintain the G-protein in its inactive state.
The Gα-subunits are a family of 39-52 kDa proteins that share 45% to 80% amino acid similarity. The distinction of the different types of α-subunits found in trimeric G-proteins is based upon the downstream signaling responses activated or inhibited as a result of G-protein activation. These classifications include Gs (since it is the α-subunit, these designations can also be written Gαs), Gi, Gq, and G12 (Table 40-4).
Monomeric G-proteins
The Ras superfamily of monomeric G-proteins comprises well over 100 different proteins. This superfamily is divided into 8 main families with each of these major families being comprised of several subfamilies. The 8 major families are Ras (33 members), Rho (20 members), Rab (70 members), Rap (5 members), Ran, Rheb (2 members), Rad, Rit (2 members), and Arf (30 members). The Ras subfamily is primarily responsible for regulation of events of cell proliferation, while the Rho subfamily is involved in the regulation of cell morphology through control of cytoskeletal dynamics. The Rab subfamily is involved in membrane-trafficking events and the Rap subfamily is involved in control of cell adhesion. While the Ran subfamily is responsible for the regulation of nuclear transport, the Rheb subfamily gets its name from the original member identified as Ras homolog expressed in brain. The Rheb proteins are involved in the regulation of mTOR (mammalian target of rapamycin, see Chapter 46). The Arf subfamily is involved in intracellular vesicle transport.
G-Protein Regulators
The activity state of G-proteins is regulated both by the rate of GTP exchange for GDP and by the rate at which the GTP is hydrolyzed to GDP. The former process is catalyzed by guanine nucleotide exchange factors GEFs. The activity of G-proteins with respect to GTP hydrolysis is regulated by a family of proteins termed GTPase-activating proteins, GAPs. Both of these G-protein regulatory protein classes are termed regulators of G-protein signaling, RGS. Two clinically important proteins of the GAP family are the neurofibromatosis type-1 (NF1) susceptibility locus protein and the BCR locus (break point cluster region gene). The NF1 gene is a tumor suppressor and the BCR locus is rearranged in the Philadelphia chromosome (Ph+) observed with high frequency in chronic myelogenous leukemias (CMLs) and acute lymphocytic leukemias (ALLs).
G-Protein–Coupled Receptors
There are several different classifications of receptors that couple signal transduction through G-proteins. These classes of receptor are termed G-protein–coupled receptors (GPCRs).
There are at least 791 identified GPCRs in the human genome. Many do not have known ligands and are referred to as orphan GPCRs. The GPCR superfamily consists of 3 defined families or classes (class A, B, and C) as well as a group termed “others.” The latter group consists of at least 92 GPCRs that include the adhesion, frizzled, and taste type-2 receptors.
Class A family: The class A GPCR family is referred to as the rhodopsin family. Class A contains the largest number of members compiled into at least 19 subclasses (subfamilies). Class A GPCRs include opsins, the vast majority of the odorant receptors (at least 290 receptors), and receptors for monoamines, purines, opioids, chemokines, some small peptide hormones, and the large glycoprotein hormones that consist of thyroid-stimulating hormone (TSH), luteinizing hormone (LH), and follicle-stimulating hormone (FSH).
Class B family: The class B GPCR family is referred to as the secretin-like receptor class. Class B is composed of 34 subclasses (subfamilies) and members include receptors for peptide hormones, such as parathyroid hormone (PTH), parathyroid hormone-related protein (PTHrP), and calcitonin. The class B family also contains the vast majority of the orphan GPCRs.
Class C family: The class C family is referred to as the metabotropic receptor or glutamate receptor-like family. Class C is composed of 8 subclasses (subfamilies) and all members form dimers and include the metabotropic glutamate receptors (mGluR), extracellular Ca2+-sensing receptors, taste (gustatory) receptors, and several odorant receptors, as well as the pheromone receptors.
High-Yield Concept
All GPCRs are composed of a similar structure that includes 7 membrane-spanning helices connected by 3 intracellular loops and 3 extracellular loops with an extracellular amino terminus and an intracellular carboxy terminus (Figure 40-3).
FIGURE 40-3: A diagrammatic representation of a typical member of the serpentine class of G-protein–coupled receptor. White, red, blue, and green spheres represent amino acids. Serpentine receptors are so called because they pass through the plasma membrane 7 times. Structural characteristics include the 3 extracellular loops (EL-1, EL-2, EL-3) and 3 intracellular loops (IL-1, IL-2, IL-3). Most GPCRs are modified by carbohydrate attachment to the extracellular portion of the protein. Shown is typical N-linked carbohydrate attachment. The different-colored spheres are involved in ligand binding and associated G-protein binding as indicated in the legend. Reproduced with permission of themedicalbiochemistrypage, LLC.
A characteristic feature of GPCR activity following ligand binding is a progressive loss of receptor-mediated signal transduction. This process is referred to as desensitization or adaptation. The events that reflect desensitization of a G-protein–coupled signaling system can involve the receptor itself, the G-protein associated with the receptor, and/or the downstream effector(s). In the majority of cases, it is impairment of the receptors’ ability to activate its G-protein that accounts for most desensitization. This process involves phosphorylation of the GPCRs on one or more intracellular domains. On a longer time scale (several hours after ligand binding), the short-term desensitization is augmented by receptor downregulation, which involves the loss of membrane-associated receptor through a combination of protein degradation, transcriptional, and posttranscriptional mechanisms.
Heterologous desensitization involves phosphorylation of GPCRs by second-messenger–dependent kinases, such as PKA and PKC. Homologous desensitization of GPCRs involves a family of kinases termed G-protein–coupled receptor kinases (GRKs). The GRKs constitute a family of 6 mammalian serine/threonine kinases that phosphorylate ligand-activated GPCRs as their primary substrates. These 6 kinases are identified as GRK1 (originally called rhodopsin kinase); GRK2 (originally called β-adrenergic receptor kinase-1, βARK1); GRK3 (originally called β-adrenergic receptor kinase-2, βARK2); GRK4 (originally called IT-11); GRK5; and GRK6.
Upon receptor phosphorylation by a GRK, there is binding to one of a family of cytoplasmic inhibitory proteins called the arrestins. In the rhodopsin system, this inhibitory protein is referred to as arrestin. In nonretinal tissues, there are 2 related inhibitory proteins known as β-arrestin-1 and β-arrestin-2. As a result of arrestin or β-arrestin binding, the GPCR is prevented from activating its G-protein and, therefore, its downstream effector(s).
There are at least 25 known disorders that are the result of defects in a variety of different GPCRs; some of these are described in Table 40-5.
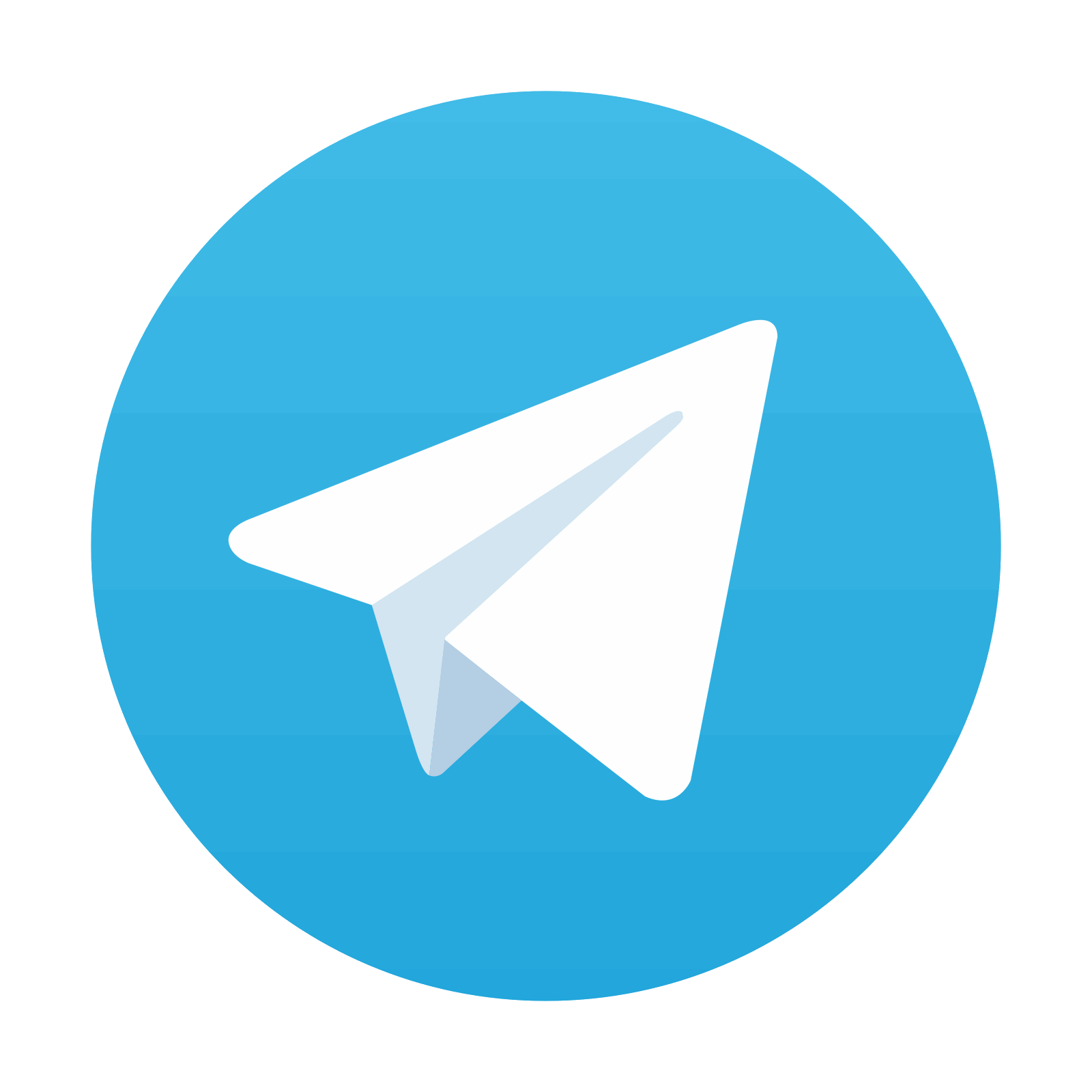
Stay updated, free articles. Join our Telegram channel

Full access? Get Clinical Tree
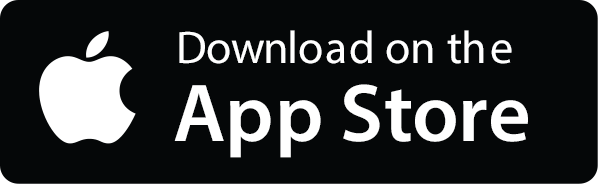
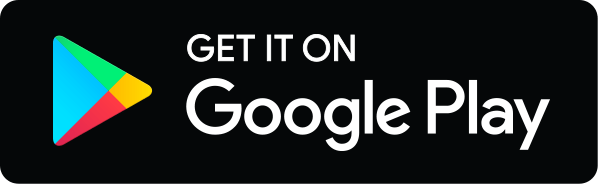