CHAPTER OUTLINE
Basics of Peptide Hormone Structure and Function
Receptors for Peptide Hormones
The Hypothalamic-Pituitary Axis
The Proopiomelanocortin Family
Human Chorionic Somatomammotropin
Irisin: Exercise-Induced Skeletal Muscle Hormone
High-Yield Terms
Endocrine glands: secrete chemicals or hormones into the circulation
Exocrine glands: excretes chemicals or hormones via ducts
Paracrine: refers to any substance secreted by one cell which acts on cells next to or in close proximity to the source
Autocrine: refers to any substance secreted by one cell which acts on the secreting cell itself
Cretinism: a condition of severely stunted physical and mental growth due to untreated congenital deficiency of thyroid hormones
Thyrotoxicosis: the condition resulting from hyperthyroidism leading to excess production and release of thyroid hormoness
Basics of Peptide Hormone Structure and Function
The integration of metabolic and hemostatic functions is carried out by the nervous system, the immune system, and the endocrine system. The endocrine system is composed of a number of tissues that secrete their products, endocrine hormones, into the circulatory system; from there they are disseminated throughout the body, regulating the function of distant tissues and maintaining homeostasis. In a separate but related system, exocrine tissues secrete their products into ducts and then to the outside of the body or to the intestinal tract. Classically, endocrine hormones are considered to be derived from amino acids, peptides (Table 49-1), or sterols (Chapter 50) and to act at sites distant from their tissue of origin. However, some secreted substances act at a distance (classical endocrines), close to the cells that secrete them (paracrine), or directly on the cell that secreted them (autocrine).
Hormones are normally present in the plasma and interstitial tissue at concentrations in the range of 10−7 M to 10−10 M. Because of these very low physiological concentrations, sensitive protein receptors have evolved in target tissues to sense the presence of very low concentrations of ligands. In addition, systemic feedback mechanisms have evolved to regulate the production and activity of endocrine hormones.
Once a hormone is secreted by an endocrine tissue, it generally binds to a specific plasma protein carrier, with the complex being disseminated to distant tissues. Plasma carrier proteins exist for all classes of endocrine hormones. Carrier proteins for peptide hormones prevent hormone destruction by plasma proteases. Carriers for steroid and thyroid hormones allow these very hydrophobic substances to be present in the plasma at concentrations several hundred-fold greater than their solubility in water would permit. Carriers for small, hydrophilic amino acid-derived hormones prevent their filtration through the renal glomerulus, greatly prolonging their circulating half-life.
Many amino acid and peptide hormones are elaborated by neural tissue, with ultimate impact on the entire system. The hypothalamic-releasing hormones (archaically called releasing factors) represent the archetype family of neutrally derived peptide hormones. Releasing hormones are synthesized in neural cell bodies of the hypothalamus and secreted at the axon terminals into the portal hypophyseal circulation, which directly bathes the anterior pituitary (see below). The pituitary hormones are carried via the systemic circulation to target tissues throughout the body. At the target tissues they generate unique biological activities.
Receptors for Peptide Hormones
With the exception of the thyroid hormone receptors, the receptors for amino acid–derived and peptide hormones are located in the plasma membrane. Receptor structure for amino acid and peptide hormones is varied and includes single transmembrane–spanning receptors, G-protein–coupled receptors (GPCRs), and multisubunit receptors.
Upon hormone binding signals are transduced to the interior of the cell, where second messengers and phosphorylated proteins generate appropriate metabolic responses. The main second messengers are cAMP, Ca2+, inositol triphosphate (IP3), and diacylglycerol (DAG). The generation of cAMP occurs via activation of GPCRs whose associated G-proteins (Gs) activate adenylate cyclase generating cAMP and the subsequent activation of PKA.
Peptide hormone GPCRs also couple to G-protein (Gq) activation of phospholipase C-β (PLCβ), which then hydrolyzes membrane phosphatidylinositol 4,5-bisphosphate (PIP2) to produce 2 messengers: IP3, which is soluble in the cytosol, and DAG, which remains in the membrane phase. Cytosolic IP3 binds to sites on the ER, opening Ca2+ channels and allowing stored Ca2+ to flood the cytosol. There it activates numerous enzymes, many by activating their calmodulin or calmodulin-like subunits. DAG has 2 roles: it binds and activates protein kinase C (PKC), and it opens Ca2+ channels in the plasma membrane, reinforcing the effect of IP3. Like PKA, PKC phosphorylates serine and threonine residues of many proteins, thus modulating their catalytic activity.
Only one receptor class, that for the natriuretic peptides (eg, atrial natriuretic peptide, ANP: also sometimes called atrial natriuretic factor, ANF) has been shown to be coupled to the production of intracellular cGMP. The receptors for the natriuretic factors are integral plasma membrane proteins, whose intracellular domains catalyze the formation of cGMP following ligand binding. Intracellular cGMP activates a protein kinase G (PKG), which phosphorylates and modulates enzyme activity, leading to the biological effects of the natriuretic factors.
The Hypothalamic-Pituitary Axis
The hypothalamus is located below the thalamus and just above the brain stem and is composed of several domains (nuclei) that perform a variety of functions. The hypothalamus forms the ventral portion of the region of the brain called the diencephalon. Anatomically the hypothalamus is divided into 3 broad domains termed the posterior, tuberal, and anterior regions. Each of these 3 regions is further subdivided into medial and lateral areas. The various nuclei of the hypothalamus constitute the functional domains of the various hypothalamic areas. One important function of the hypothalamus is to link the central nervous system to the endocrine system via the pituitary gland (also termed the hypophysis). The hypothalamus is involved in the control of certain metabolic processes as well as other functions of the autonomic nervous system. The hypothalamus synthesizes and secretes a variety of neurohormones, referred to as hypothalamic-releasing hormones (or factors), that act upon the pituitary to direct the release of the various pituitary hormones (Figure 49-1).
FIGURE 49-1: Diagrammatic representation of the interactions between the hypothalamus and the pituitary. The hypothalamic releasing and inhibiting hormones exert their effects on the release of anterior pituitary hormones. Oxytocin and vasopressin (antidiuretic hormone) are released directly from hypothalamic axons that terminate in the posterior pituitary, and the hormones are secreted from there directly into the systemic circulation. Reproduced with permission of themedicalbiochemistrypage, LLC.
The pituitary gland has 2 lobes called the posterior and anterior lobes. Each lobe secretes peptide hormones that exert numerous effects on the body. The posterior pituitary excretes the 2 hormones, oxytocin and vasopressin. The anterior pituitary secretes 6 hormones: (1) adrenocortiocotropic hormone (ACTH, also called corticotropin), (2) thyroid-stimulating hormone (TSH), (3) follicle-stimulating hormone (FSH), (4) luteinizing hormone (LH), (5) growth hormone (GH), and (6) prolactin (PRL). The hormone ACTH is derived from a large precursor protein identified as proopiomelanocortin (POMC). The secretion of the anterior pituitary hormones is under control of the hypothalamus, hence the description of the system as the hypothalamic-pituitary axis. The secretion of the hormones ACTH, TSH, FSH, LH, and GH are stimulated by signals from the hypothalamus, whereas PRL secretion is inhibited by hypothalamic signals.
High-Yield Concept
The cell types that synthesize and secrete the various anterior pituitary hormones are specialized secretory cells. The cells that secrete ACTH are called corticotropes (or corticotrophs). The cells that secrete thyroid-stimulating hormone are called thyrotropes (or thyrotrophs). The cells that secrete follicle-stimulating hormone and luteinizing hormone are called gonadotropes (or gonadotrophs). The cells that secrete prolactin are called lactotropes (or lactotrophs). The cells that secrete growth hormone are called somatotropes (or somatotrophs).
The secretion of anterior pituitary hormones results in response to hypophysiotropic hormones that are carried in the portal hypophysial vessels from the hypothalamus to the pituitary. These hypothalamic hormones are commonly referred to as releasing or inhibiting hormones. There are 7 hypothalamic releasing and inhibiting hormones: (1) corticotropin-releasing hormone (CRH), (2) thyrotropin-releasing hormone (TRH), (3) gonadotropin-releasing hormone (GnRH), (4) luteinizing hormone–releasing hormone (LHRH), (5) growth hormone–releasing hormone (GRH or GHRH), (6) growth hormone release–inhibiting hormone (GIH, more commonly called somatostatin), and (7) prolactin release–inhibiting hormone (is in fact the catecholamine, dopamine). Hypothalamic extracts also contain a prolactin-releasing substance (sometimes referred to as prolactin-releasing hormone, PRH). Several peptides found in the hypothalamus (eg, TRH) can stimulate prolactin secretion, so it is as yet unclear whether PRH is the physiologic prolactin-releasing substance. GnRH has been shown to stimulate the release of both FSH and LH and as a consequence the term GnRH is more appropriately used than LHRH.
The hypothalamic-releasing and -inhibiting hormones are secreted from the median eminence of the hypothalamus. The GnRH-secreting neurons are primarily in the medial preoptic area of the hypothalamus. The somatostatin-secreting neurons reside in the periventricular nuclei. The TRH-secreting and CRH-secreting neurons are found in the medial parts of the periventricular nuclei. The GRH-secreting neurons reside in the arcuate nuclei, which is the same region that contains dopamine-secreting neurons. Most of the receptors for the hypophysiotropic hormones are GPCRs.
Additional important hypothalamic peptides include the orexins, melanin-concentrating hormone (MCH), members of the pancreatic polypeptide (PP) family, cocaine- and amphetamine-regulated transcript (CART) peptide, Agouti-related peptide (AgRP), galanin (GAL), and galanin-like peptide (GALP). The functions of each of these peptides are discussed in Chapter 44.
Vasopressin and Oxytocin
The principal hormones of the posterior pituitary are the nonapeptides oxytocin and vasopressin. Vasopressin is also called arginine vasopressin (AVP) or antidiuretic hormone (ADH). The amino acid sequences of vasopressin and oxytocin differ by only 2 amino acids. Both of these hormones are synthesized as prohormones in neural cell bodies of the hypothalamus and mature as they pass down axons in association with carrier proteins termed neurophysins. The axons terminate in the posterior pituitary, and the hormones are secreted directly into the systemic circulation. The neurophysins themselves are derived from the oxytocin and vasopressin preproproteins. The oxytocin preproprotein contains neurophysin I and the vasopressin preproprotein contains neurophysin II.
Vasopressin is also known as antidiuretic hormone (ADH), because it is the main regulator of body fluid osmolarity. The designation arginine vasopressin (AVP) is used when discussing vasopressins from different mammals as marsupials and pigs produce a vasopressin peptide where the arginine is replaced by a lysine and is thus, referred to as lysine vasopressin. The secretion of vasopressin is regulated in the hypothalamus by osmoreceptors which sense water and Na+ concentration and stimulate increased vasopressin secretion when plasma osmolarity increases. The secreted vasopressin increases the reabsorption rate of water in kidney tubule cells, causing the excretion of urine that is concentrated in Na+ and thus yielding a net drop in osmolarity of body fluids.
Vasopressin binds plasma membrane receptors which activate signaling events through G-proteins coupled to the cAMP second messenger system or through the PLCβ pathway. There are 3 kinds of vasopressin receptors designated V1A, V1B (also called V3), and V2. The V1A (gene symbol = AVPR1A) and V1B (gene symbol = AVPR1B) receptors signal via hydrolysis of PIP2 resulting in increased intracellular Ca2+ concentration. The V2 receptors activate adenylate cyclase and result in increased cAMP levels. V1 receptors are found in blood vessels and vasopressin binding triggers vascular contraction resulting in increased blood pressure. The V2 receptors are found primarily in the collecting ducts of the kidneys and are responsible for triggering vasopressin-mediated water retention, thereby affecting osmolarity. Mutations in the gene encoding the V2 receptor (gene symbol = AVPR2) are responsible for X-linked nephrogenic diabetes insipidus.
High-Yield Concept
Vasopressin deficiency leads to production of large volumes of watery urine and to polydipsia. These symptoms are diagnostic of a condition known as diabetes insipidus. Diabetes insipidus has numerous causes that include effects on both the pituitary and kidneys.
Oxytocin is produced in the magnocellular neurosecretory cells of the hypothalamus and then stored in axon terminals of the anterior pituitary. While stored in the pituitary, oxytocin is bound to neurophysin I in Herring bodies. Secretion of oxytocin is stimulated by electrical activity of the oxytocin cells of the hypothalamus. The actions of oxytocin are elicited via the interaction of the hormone with high affinity receptors. The oxytocin receptor is a GPCR whose affinity for ligand is dependent upon Mg2+ and cholesterol, both of which act as positive allosteric regulators. Oxytocin secretion in nursing women is stimulated by direct neural feedback obtained by stimulation of the nipple during suckling. This response to oxytocin is referred to as the “let-down response.” Its physiological effects include the contraction of mammary gland myoepithelial cells, which induces the ejection of milk from mammary glands. The other primary action of oxytocin is the stimulation of uterine smooth muscle contraction leading to childbirth.
In males the circulating levels of oxytocin increase at the time of ejaculation. It is believed that the increase in oxytocin levels causes increased contraction of the smooth muscle cells of the vas deferens, thereby propelling the sperm toward the urethra.
The Gonadotropins
The glycoprotein hormones are the most chemically complex family of the peptide hormones and include TSH and the gonadotropins, FSH, LH, and hCG. All members of the family are highly glycosylated. Each of the glycoprotein hormones is an (α:β) heterodimer, with the α-subunit being identical in all members of the family. The biological activity of the hormone is determined by the β-subunit, which is not active in the absence of the α-subunit. The α-subunit gene is identified as chorionic gonadotropin, alpha: CGA. All members of the glycoprotein hormone family transduce their intracellular effects via GPCRs coupled to adenylate cyclase activation. The gonadotropins bind to cells in the ovaries and testes, stimulating the production of the steroid sex hormones estrogen, testosterone (T), and dihydrotestosterone (DHT).
FSH and LH
The synthesis and release of FSH and LH is controlled by the action of the hypothalamic-releasing factor, GnRH. The function of GnRH is to induce an episodic release of both FSH and LH that determines the onset of puberty and ovulation in females. GnRH binds to its receptor on gonadotropes and initiates a signaling cascade that results in the release of FSH and LH.
The control of the hypothalamic-pituitary axis at the level of FSH and LH is controlled by several additional proteins including follistatin, activin, and leptin. Follistatin is a protein that binds to and inhibits proteins of the transforming growth factor-β family (TGFβ) of which activin is a member. Therefore, follistatin inhibits the activity of activin on promoting FSH synthesis and release.
In females, FSH stimulates follicular development and estrogen synthesis by granulosa cells of the ovary. In males, FSH promotes testicular growth and within the Sertoli cells of the testes it enhances the synthesis and secretion of T and DHT. In females, LH induces thecal cells of the ovary to synthesize estrogens and progesterone and promotes estradiol secretion. The surge in LH release that occurs in midmenstrual cycle is the responsible signal for ovulation. Continuous LH secretion stimulates the corpus luteum to produce progesterone. In males, LH binds to Leydig cells of the testes to induce the secretion of T.
hCG
Human chorionic gonadotropin (hCG) is produced only during pregnancy. The actions of hCG are exerted on luteal cells of the ovary. Initially the developing embryo synthesizes and secretes hCG. Following implantation the cells of the syncytiotrophoblast produce and secrete hCG. The production of hCG increases markedly after implantation; its appearance in the plasma and urine is one of the earliest signals of pregnancy and the basis of many pregnancy tests. The role of hCG during pregnancy is to prevent disintegration of the corpus luteum so as to maintain the synthesis of progesterone by this tissue.
Thyroid-Stimulating Hormone
Secretion of TSH is stimulated by thyrotropin-releasing hormone (TRH) from the hypothalamus (Figure 49-2). TRH, a tripeptide, is synthesized by neurons in the supraoptic and supraventricular nuclei of the hypothalamus and stored in the median eminence. TRH is transported to the anterior pituitary via the pituitary portal circulation and binds to a specific receptor located on thyroid-stimulating hormone (TSH) and prolactin-secreting cells. Binding of TRH to its receptor activates a typical PLCγ-mediated signaling cascade. The TRH-induced signaling leads to TSH secretion as well as increased TSH transcription and posttranslational glycosylation. The TRH-mediated release of TSH is pulsatile with peak secretion being exerted between midnight and 4 AM.
FIGURE 49-2: The hypothalamic-hypophysial-thyroidal axis. TRH produced in the hypothalamus reaches the thyrotrophs in the anterior pituitary by the hypothalamo-hypophysial portal system and stimulates the synthesis and release of TSH. In both the hypothalamus and the pituitary, it is primarily T3 that inhibits TRH and TSH secretion, respectively. T4 undergoes monodeiodination to T3 in neural and pituitary as well as in peripheral tissues. Gardner DG, Shoback D. Greenspan’s Basic and Clinical Endocrinology, 9th ed., Figure 7-15, New York: McGraw-Hill; 2011.
The synthesis and release of TSH is controlled by two principal pathways. The first is exerted by the level of triiodothyronine (T3; see Chapter 50) within thyrotropic cells which regulates TSH expression, translation, and release. The second mode of regulation is that exerted by TRH. While in the circulation TSH binds to receptors on the basal membrane of thyroid follicles triggering a signaling cascade that results in increased secretion of the thyroid hormones, T3, and thyroxine (T4). TSH-binding to its receptor also results in increased TSH synthesis and thyroid cell growth. TSH causes an increase in the synthesis of a major thyroid hormone precursor, thyroglobulin. Thyroglobulin is glycosylated and contains more than 115 tyrosine residues, which become iodinated and are used to synthesize T3 and T4 (see Chapter 50).
Numerous congenital and acquired forms of hypothyroidism and hyperthyroidism are the result of alterations in the expression, processing, and function of the TSH receptor (TSHR). The most common TSHR disorder resulting in hyperthyroidism (thyrotoxicosis) is Graves disease (Clinical Box 49-1).
When hypothyroidism is evident in conjunction with sufficient iodine intake, it is either autoimmune disease (Hashimoto thyroiditis) or the consequences of treatments for hyperthyroidism that are the cause. In the embryo, thyroid hormone is necessary for normal development and hypothyroidism in the embryo is responsible for cretinism, which is characterized by multiple congenital defects and mental retardation. Because the neurological consequences of congenital hypothyroidism are severe, neonatal screening for thyroid hormone levels at birth is routine. Most infants born with congenital hypothyroidism appear normal at birth. However, if left untreated the symptoms will include a thick protruding tongue, poor feeding, prolonged jaundice (which exacerbates the neurological impairment), hypotonia (recognized as “floppy baby syndrome”), episodes of choking, and delayed bone maturation resulting in short stature.
The Proopiomelanocortin Family
POMC is expressed in both the anterior and intermediate lobes of the pituitary gland. The primary protein product of the POMC gene is a 285-amino-acid precursor that can undergo differential processing to yield at least 8 peptides, dependent upon the location of synthesis and the stimulus leading to their production. POMC is produced in the pituitary, the ARC of the hypothalamus, the nucleus of the solitary tract (NTS), as well as in several peripheral tissues such as the skin and reproductive organs. Within the brain, neurons that respond to POMC-derived peptides (termed POMCm neurons) are critical in the regulation of overall energy balance via the melanocortin peptides, primarily α-MSH (see Chapter 44).
CLINICAL BOX 49-1: GRAVES DISEASE
Graves disease is the most common cause of hyperthyroidism in the developed world representing 50% to 80% of all cases. In the United States, Graves disease also represents the most common autoimmune disease. Graves disease occurs at highest frequency in women, individuals with other autoimmune disorders, and smokers. The disease results from an autoimmune defect in genetically susceptible individuals resulting in thyroid hormone excess (thyrotoxicosis) and thyroid gland hyperplasia. These individuals produce thyroid-stimulating autoantibodies (TSAb; also called thyroid-stimulating immunoglobulins, TSIs), which bind to and activate the TSH receptor (TSHR). TSAbs bind to the TSHR and mimic the TSH stimulation of thyroid follicular cells. The hyperactivated thyroid then secretes excessive T3 and T4. The onset of Graves disease is most often acute due to the sudden production of TSHR antibodies. Patients will experience classical symptoms of hyperthyroidism including weight loss in the face of increased appetite, irritability, insomnia, diarrhea, muscle weakness, heart palpitations, heat intolerance, and increased sweat production. The clinical features of Graves disease are thyrotoxicosis, goiter (enlarged thyroid gland), an ophthalmopathy in the form of exophthalmos (eyes bulge out), and dermopathy in the form of pretibial myxedema (localized lesions of the skin, primarily in the lower legs, resulting from the deposition of hyaluronic acid). Untreated Graves disease poses serious risks that includes psychiatric illness, cardiac disease, arrthymia, and sudden cardiac death. The most serious complication is myxedema coma. This condition occurs more frequently in older women who also have underlying pulmonary and vascular disease. Myxedema coma manifests with hypothermia, hypoventilation, hypoglycemia, hyponatremia, progressive weakness, and stupor. The condition can lead to shock and death. Treatment of Graves disease involves the use of thionamides (propylthiouracil, PTU and methimazole, MMI: these drugs block thyroid hormone synthesis), radioactive iodine therapy, or surgical removal of the thyroid gland.
High-Yield Concept
Graves disease represents the most common form of hyperparathyroidism. At the other end of the spectrum are disorders that lead to hypothyroidism. Deficiency in iodine is the most common cause of hypothyroidism worldwide. Indeed the practice of producing iodized table salt was to stem the occurrence of hypothyroidism.
The processing of POMC involves glycosylations, acetylations, and extensive proteolytic cleavage at sites shown to contain regions of basic protein sequence (Figure 49-3). The proteases that recognize these cleavage sites are tissue-specific, so, for example, the physiologically active product of the anterior pituitary is ACTH.
FIGURE 49-3: Processing of the POMC precursor protein. Cleavage sites consist of the sequences, Arg-Lys, Lys-Arg, or Lys-Lys. Enzymes responsible for processing of POMC peptides include prohormone convertase 1/3 (PC1/3), prohormone convertase 2 (PC2), carboxypeptidase E (CPE), peptidyl α-amidating monooxygenase (PAM), N-acetyltrasferase (N-AT), and prolylcarboxypeptidase (PRCP). Adrenocorticotropic hormone (ACTH) and β-lipotropin are products generated in the corticotrophic cells of the anterior pituitary under the control of corticotrophin-releasing hormone (CRH). Alpha-melanocyte–stimulating hormone (α-MSH), corticotropin-like intermediate lobe peptide (CLIP), γ-lipotropin, and β-endorphin are products generated in the intermediate lobe of the pituitary under the control of dopamine. α-, β- and γ-MSH are collectively referred to as melanotropin or intermedin. The blue-shaded boxes represent the heptapeptide sequence that constitutes the MSH core. Reproduced with permission of themedicalbiochemistrypage, LLC.
Adrenocorticotropic Hormone
Adrenocorticotropic hormone (ACTH) is the main physiologically active product of the actions of the hypothalamic-releasing hormone (CRH) on the anterior pituitary. Although CRH is the primary stimulus for ACTH release, other hormones also exert effects on ACTH release. CRH stimulates a pulsatile secretion of ACTH with peak levels seen before waking and declining as the day progresses. Negative feedback on ACTH secretion is exerted by cortisol at both the hypothalamic and anterior pituitary levels. Thus, the primary product of the systemic actions of ACTH regulates the further actions of this corticotropic hormone. Additional factors that influence ACTH secretion include physical, emotional, and chemical stresses. These stressors include pain, cold exposure, acute hypoglycemia, trauma, depression, and surgery. The stress-mediated increases in ACTH secretion are the result of the actions of vasopressin and CRH.
The biological role of ACTH is to stimulate the production of adrenal cortex steroids, principally the glucocorticoids, cortisol, and costicosterone. ACTH also stimulates the adrenal cortex to produce the mineralocorticoid, aldosterone, as well as the androgen, androstenedione. ACTH exerts its effects on the adrenal cortex by binding to a specific receptor that is a member of the melanocortin receptor family, melanocortin-2 receptor (MC2R). The main cortical cell response to ACTH is increased activity of CYP11A1 (also called P450-linked side chain-cleaving enzyme, P450ssc, 20,22-desmolase, or cholesterol desmolase), which converts cholesterol to pregnenolone during steroid hormone synthesis (Chapter 50).
Secondary adrenal insufficiency occurs in patients with deficiencies in pituitary ACTH production or secretion. Whereas, primary adrenal insufficiency (adrenal hypoplasia) is characteristic of Addison disease (see Clinical Box 50-1), which was originally diagnosed as the result of lesions in the adrenal glands caused by tuberculosis. Secondary adrenal insufficiency is characterized by weakness, fatigue, nausea, vomiting, and anorexia. On the opposite side of the abnormal ACTH spectrum are the adrenal hyperplasias. These include the congenital adrenal hyperplasias (CAH; see Chapter 50) and Cushing syndrome (see Clinical Box 50-2).
Growth Hormone
Growth hormone (GH), human chorionic somatomammotropin, hCS (also called human placental lactogen, hPL), and prolactin (PRL) comprise the growth hormone family. All have about 200 amino acids, 2 disulfide bonds, and no glycosylation. Although each has special receptors and unique characteristics to their activity, they all possess growth-promoting and lactogenic activity.
Mature GH is synthesized in acidophilic pituitary somatotropes as a single polypeptide chain. Because of alternate RNA splicing, a small amount of a somewhat smaller molecular form is also secreted. Expression of GH occurs only in the pituitary. The growth-promoting effects of GH are due to induced secretion of insulin-like growth factor 1 (IGF-1). The metbolic effects of GH include the promotion of gluconeogenesis and amino acid uptake by cells, and it induces the breakdown of tissue lipids which provides the energy needed to support the stimulated protein synthesis induced by increased amino acid uptake.
There are a number of genetic deficiencies associated with GH. GH-deficient dwarfs lack the ability to synthesize or secrete GH, and these short-statured individuals respond well to GH therapy. Pygmies lack the IGF-1 response to GH but not its metabolic effects. Laron dwarfs have normal or excess plasma GH, but lack liver GH receptors and have low levels of circulating IGF-1. The defect in these individuals is clearly related to an inability to respond to GH by the production of IGF-1. The production of excessive amounts of GH before epiphyseal closure of the long bones leads to gigantism, and when GH becomes excessive after epiphyseal closure, acral bone growth leads to the characteristic features of acromegaly.
Human Chorionic Somatomammotropin
Human chorionic somatomammotropin (hCS) is produced by the placenta late in gestation. At its peak, hCS is secreted at a rate of about 1 g/d, the highest secretory rate of any known human hormone. However, little hCS reaches the fetal circulation. The amount of hCS that is secreted is proportional to the size of the placenta. Low levels of hCS during pregnancy are a sign of placental insufficiency. The biological actions of hCS are similar to those of GH suggesting that it functions as a maternal growth hormone of pregnancy. The hormone induces the retention of potassium, calcium, and nitrogen; decreases glucose utilization; and increases lipolysis.
Prolactin
Prolactin (PRL) is produced by acidophilic pituitary lactotropes. Prolactin is known to bind zinc (Zn2+) and the binding of this metal stabilizes prolactin in the secretory pathway. Prolactin is the lone tropic hormone of the pituitary that is routinely under negative control by prolactin release–inhibiting hormone (PIH or PIF), which is now known to be dopamine. Decreased hypophyseal dopamine production, or damage to the hypophyseal stalk, leads to rapid upregulation of PRL secretion. Prolactin does not appear to play a role in normal gonadal function, yet hyperprolactinemia in humans results in hypogonadism.
Prolactin secretion increases during pregnancy and promotes breast development in preparation for the production of milk and lactation. During pregnancy the increased production of estrogen enhances breast development, but it also suppresses the effects of prolactin on lactation. Following parturition, estrogen (as well as progesterone) levels fall allowing lactation to occur. This is to ensure that lactation is not induced until the baby is born.
Irisin: Exercise-Induced Skeletal Muscle Hormone
Irisin, named after the Greek Goddess Iris, messenger of the Olympian Gods, was discovered in studies aimed at defining mechanisms by which exercise results in improvement in metabolic status in obesity and Type 2 diabetes (T2D). Exercise has been shown to increase whole body energy expenditure in excess of the calories that are required for the actual work performed. The role of exercise in improving metabolic status in tissues other than skeletal muscle suggested that a protein was secreted from exercising muscle and acting on tissues such as adipose tissue and liver. This secreted protein is irisin and it derived by proteolytic cleavage of a proprotein encoded by the fibronectin type III domain-containing protein 5 (FNDC5) gene. The highest basal levels of expression of FNDC5 are seen in brain and heart, with low basal expression in liver, lung, skeletal muscle, and testis.
Irisin expression and secretion is induced in response to exercise and activates profound changes in the subcutaneous white adipose tissue (WAT), stimulating expression of uncoupling protein 1 (UCP1), and results in a broad program of brown fat-like development. These BAT-like cells induced in WAT are most commonly referred to as beige or brite fat cells. Importantly, this causes a significant increase in total body energy expenditure and resistance to obesity-linked insulin resistance. The actions of irisin recapitulate many of the most important benefits of exercise and muscle activity.
The therapeutic potential of irisin is obvious. Exogenously administered irisin can induce the browning of subcutaneous fat and thermogenesis, and it presumably could be prepared and delivered as an injectable polypeptide. Increased formation of brown or beige/brite fat has been shown to have antiobesity and antidiabetic effects.
Natriuretic Hormones
Natriuresis refers to enhanced urinary excretion of sodium. This can occur in certain disease states and through the action of diuretic drugs. At least 3 natriuretic hormones have been identified. Atrial natriuretic peptide (ANP) was the first cardiac natriuretic hormone identified. This hormone is secreted by cardiac muscle when sodium chloride intake is increased and when the volume of the extracellular fluid expands. A brain natriuretic peptide (BNP) has been identified and found in human heart and blood (but not human brain). Human BNP is also referred to as ventricular natriuretic factor but is still identified as BNP. In humans, a third natriuretic peptide (CNP) is present in the brain but not in the heart.
The action of ANP is to cause natriuresis presumably by increasing glomerular filtration rate (its exact mechanism of action remains unclear). ANP induces relaxation of the mesangial cells of the glomeruli and thus may increase the surface area of these cells so that filtration is increased. Alternatively, ANP might act on tubule cells to increase sodium excretion. Other effects of ANP include reducing blood pressure, decreasing the responsiveness of adrenal glomerulosa cells to stimuli that results in aldosterone production and secretion, inhibition of secretion of vasopressin, and decrease in vascular smooth muscle cell responses to vasoconstrictive agents. These latter actions of ANP are counter to the effects of angiotensin II. ANP also lowers renin secretion by the kidneys, thus lowering circulating angiotensin II levels.
Three different ANP receptors have been identified: ANPR-A, ANPR-B, and ANPR-C. Both ANPR-A and ANPR-B proteins span the plasma membrane and their intracellular domains possess intrinsic guanylate cyclase activity. When ANP binds there is an increase in guanylate cyclase activity leading to production of cyclic GMP (cGMP), which serves as the second messenger for ANP (or BNP and CNP) signaling.
Renin-Angiotensin System
The renin-angiotensin system is responsible for regulation of blood pressure. The intrarenal baroreceptor system is a key mechanism for regulating renin secretion. A drop in pressure results in the release of renin from the juxtaglomerular cells of the kidney. Renin secretion is also regulated by the rate of Na+ and Cl− transport across the macula densa. The higher the rate of transport of these ions, the lower the rate of renin secretion. The only function for renin is to cleave a 10-amino-acid peptide from the N-terminal end of angiotensinogen (Figure 49-4). This decapeptide is called angiotensin I. Angiotensin I is then cleaved by the action of angiotensin-converting enzyme (ACE) removing two amino acids from the C-terminal end of angiotensin I generating the bioactive hormone, angiotensin II.
FIGURE 49-4: Steps in the production of angiotensin peptides by the renin-angiotensin system (ACE, angiotensin-converting enzyme).
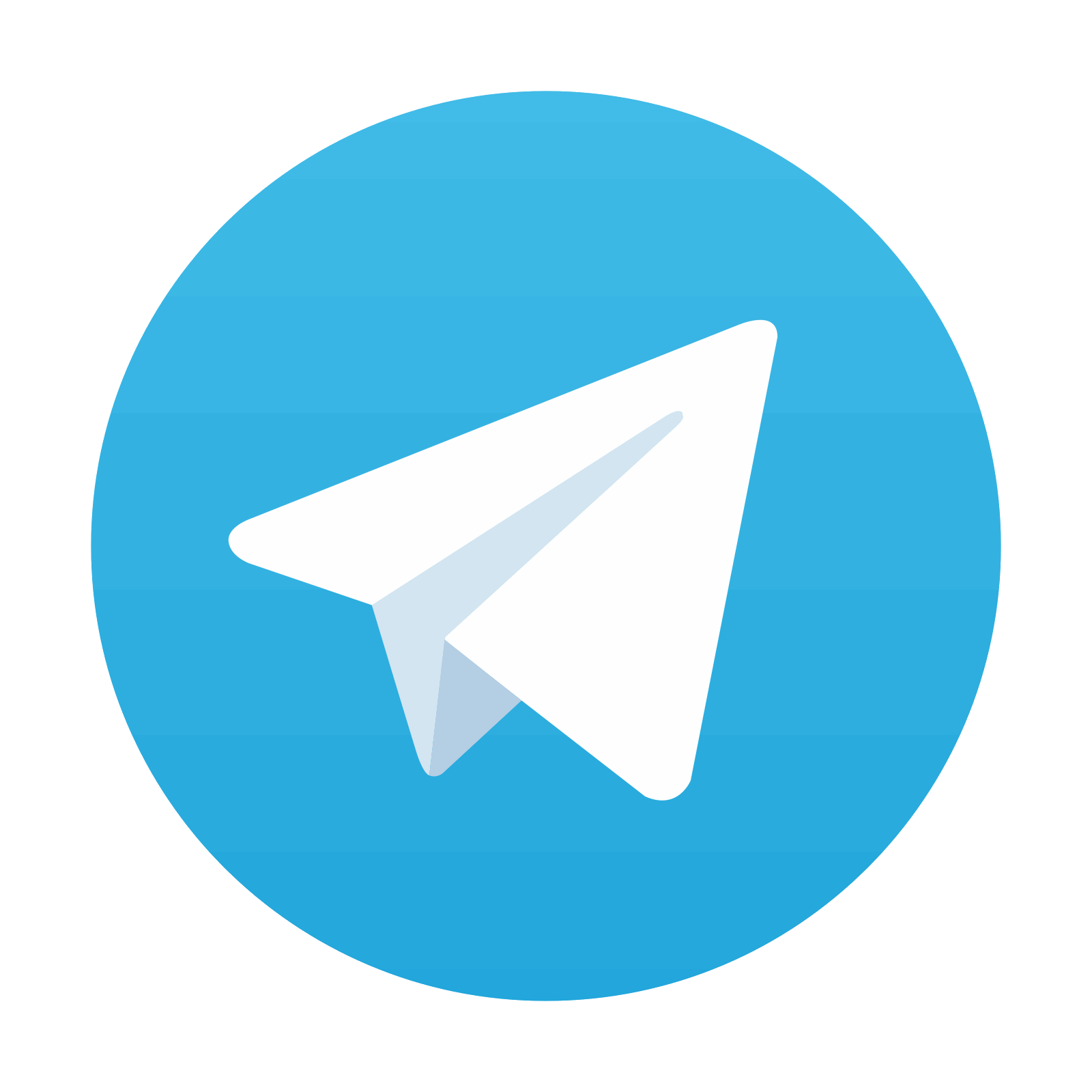
Stay updated, free articles. Join our Telegram channel

Full access? Get Clinical Tree
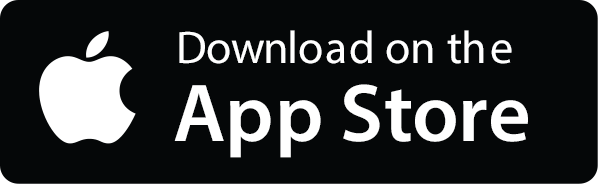
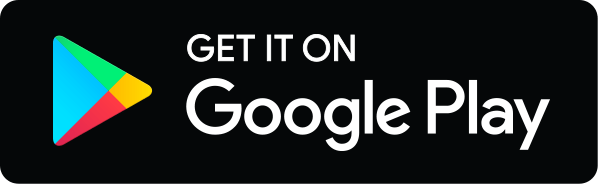