CHAPTER OUTLINE
Insulin Action and Endothelial Functions
Introduction to the Metabolic Syndrome
Genetic Factors in the Metabolic Syndrome
Metabolic Disruptions in the Metabolic Syndrome
Obesity and the Metabolic Syndrome
Testing for the Metabolic Syndrome
Treatment of the Metabolic Syndrome
High-Yield Terms
Artery: blood vessels that carry blood away from the heart
Vein: blood vessels that carry blood toward the heart
Capillary: are the smallest vessels and constitute the vessels of the microcirculation
Atherogenic: relating to the processes of initiating, increasing, and/or accelerating atherogenesis
Atheroma: represents result of the accumulation of leukocytes (mostly macrophages), lipids, and debris causing swelling of the arterial wall
Metabolic syndrome: a disorder that defines a combination of metabolic and cardiovascular risk determinants that leads to coronary artery disease (CAD)
Atherosclerosis: refers to the condition in which wall of larger arteries and veins thickens as a result of the accumulation of fatty material, cells, and extracellular matrix components
The Cardiovascular System
The cardiovascular system, also referred to as the circulatory system, is comprised of the heart and the blood vessels (Figure 48-1). The heart has 2 separate sides and each side has 2 chambers. The upper chamber on each side of the heart is called an atrium and the bottom chamber on each side is called a ventricle. The ventricles are the chambers that pump the blood with each contraction. One side of the heart (left ventricle) is designed to propel the oxygenated blood into the systemic circulation. Within the tissues the erythrocytes and the blood exchange O2 for the CO2 generated through metabolic processes. The other side of the heart (right ventricle) is designed to propel deoxygenated blood into the pulmonary circulation where the blood becomes oxygenated in the alveoli of the lungs. Within the lungs the CO2 is expelled during respiration and the erythrocyte hemoglobin becomes fully oxygenated once again.
FIGURE 48-1: Diagram of the cardiovascular system. Mescher AL. Junqueira’s Basic Histology Text and Atlas, 13th ed. New York, NY: McGraw-Hill; 2013.
The vasculature is composed of the arteries, veins, and the capillaries (Figure 48-2). Within all tissues the capillaries form a fine meshwork of tubules called the microvasculature. The capillaries are very small, thin-walled blood vessels where the exchange of gases, nutrients, and waste takes place between the cells and the blood. Arteries and veins are composed of a trilaminar structure. The inner layer of the vessels is called the intima (also called tunica intima) and is composed of a monolayer of endothelial cells. The endothelial cells are in continuous contact with the blood and as such these cells are the first to respond to the changing composition and environment of the blood. Cardiovascular disorders, such as atherosclerosis, result from the consequences of abnormal interactions between components and cells in the blood and the endothelial cell layer of the vasculature.
FIGURE 48-2: Schematics of the structures of various types of blood vessels. A. Capillaries consist of an endothelial tube in contact with a discontinuous population of pericytes. B. Veins typically have thin medias and thicker adventitias. C. A small muscular artery features a prominent tunica media. D. Larger muscular arteries have a prominent media with smooth muscle cells embedded in a complex extracellular matrix. E. Larger elastic arteries have cylindrical layers of elastic tissue alternating with concentric rings of smooth muscle cells. Loscalzo JL. Harrison’s Cardiovascular Medicine, 2nd ed., Figure 1-1, New York: McGraw-Hill; 2013.
The middle layer of the vessels is called the media (also called tunica media) and is composed of layers of smooth muscle cells. The outermost layer is called the adventitia (tunica adventitia or tunica externa) which consists of a loose extracellular matrix interspersed with fibroblasts and nerve terminals as well as an occasional mast cell. Unlike the larger arteries and veins, capillaries do not have a trilaminar structure. Capillaries are comprised of a monolayer of endothelial cells with an occasional smooth muscle-like cell called a pericyte on the outer surface. This structure allows for rapid transfer of O2 from erythrocytes to the tissues and the delivery of CO2 from the tissues to the blood and erythrocytes (see Figure 6-3).
Tracing the flow of blood through the circulatory system demonstrates that deoxygenated blood enters the heart through either the superior vena cava or the inferior vena cava. The superior vena cava brings deoxygenated blood from the upper parts of the body to the heart while the inferior vena cava delivers deoxygenated blood from the lower parts of the body. Both of these large veins deliver the deoxygenated blood to the right atrium and then to the right ventricle where it is propelled via the pulmonary artery out to the lungs. Once oxygenated, the blood returns to the heart via the pulmonary vein where it enters the left atrium, followed by the left ventricle and finally it is propelled out of the heart into the aorta. From the aorta the blood flows to the many arteries of the body and is distributed to the tissues to deliver the oxygen.
Endothelial Cells
The endothelial cell produces many endogenous substances that play important roles in the regulation of its barrier and transport functions as well as its role in vascular tone and blood flow. Two of the more important molecules secreted by endothelial cells are prostacyclin (PCI2: an eicosanoid, see Chapter 22) and nitric oxide (NO), both of which induce vasodilation. Defective endothelial cell production of NO underlies the excessive vasoconstriction seen in many pathological conditions such as atherosclerosis. NO also plays an important role in the regulation of blood coagulation by limiting platelet adhesion to the endothelium thereby, limiting platelet activation which is critical to the onset of blood coagulation (see Chapter 51).
Endothelial cells also play important roles in the regulation of intravascular and tissue inflammatory processes. Normally, the endothelial cell surface does not allow for direct contact of white blood cells (leukocytes) such as monocytes and neutrophils. However, when endothelial cells are activated by proinflammatory cytokines released from leukocytes during injury or infection, or due to the release of bacterial products, they express a number of leukocyte adhesion molecules allowing monocytes and neutrophils to adhere. Adherence of these leukocytes establishes a local proinflammatory state. Adherent monocytes invade the intimal layer where they become macrophages and can continue to secrete factors that prolong the inflammatory state as well as to induce the underlying smooth cells to become activated. The release of proinflammatory cytokines increases the local production of reactive oxygen species (ROS) that leads to increased rates peroxidation of lipids in circulating lipoproteins, particularly low-density lipoprotein (LDL). This generates oxidized LDL (oxLDL) which, at sites of macrophage invasion, results in increased oxLDL uptake by the macrophages. The macrophages become infiltrated with fat converting them to foam cells which secret high levels of proinflammatory cytokines. This process then becomes progressive and in an individual with high circulating lipid content, such as in obesity and type 2 diabetes, the end result is severe coronary artery disease (CAD, also referred to as cardiovascular disease, CVD) and atherosclerosis. The proinflammatory state and the hyperlipidemia are 2 of the underlying causes of the metabolic syndrome (MetS).
Insulin Action and Endothelial Functions
The metabolic functions of insulin are primarily reflective of its role in glucose and lipid homeostasis in skeletal muscle, adipose tissue, and liver. However, insulin also exerts important functions in other nonclassical insulin target tissues such as the brain, pancreas, and the vascular endothelium. The ability of insulin to exert vasodilator action in the vascular endothelium as a result of increased NO production is an important component of the ability of this hormone to enhance glucose uptake by skeletal muscle. The insulin-mediated signaling pathway that triggers production of NO in vascular endothelium involves the same signaling proteins (PI3K, PKD, and Akt/PKB) that are components of metabolic regulatory pathways induced by insulin (see Chapter 46). Therefore, it is understandable why the same disruptions to insulin signaling that lead to insulin resistance result in endothelial dysfunction.
The production of NO in endothelial cells is the result of the activation of endothelial nitric oxide synthase (eNOS). The production and actions of NO and the various NOS enzymes involved are discussed in more detail in Chapter 31. With respect to insulin action, the activation of endothelial PKB leads to phosphorylation and activation of eNOS and thus increased NO production.
High-Yield Concept
Endothelial cells form the critical interface (barrier) between cells, macromolecules, and ions in the blood and the tissues. The failure of endothelial cells to carry out their highly selective barrier functions is an underlying cause of many cardiovascular disorders including pulmonary edema, hypertension, and most detrimental to survival, atherosclerosis.
High-Yield Concept
In addition to modulating vascular tone by activating signaling events in the underlying vascular smooth muscle cells, endothelial cell-derived NO reduces the production of proinflammatory cytokines, reduces leukocyte and monocyte recruitment and adhesion to the endothelium, inhibits the proliferation of vascular smooth muscle cells, inhibits apoptosis, and attenuates platelet aggregation.
Inactivation of endothelial cell NO production, as occurs due to insulin resistance, results in endothelial dysfunction and promotes the development of atherosclerosis. Elevated levels of circulating free fatty acids lead to impaired insulin signaling via the PI3K-PDK-PKB pathway in vascular endothelial cells.
Insulin exerts its mitogenic, growth-promoting, and differentiation effects via a signaling pathway that involves mitogen-activated protein kinase (MAPK) which is distinct from the PI3K-PDK-PKB pathway. The MAPK-induced pathway does not play a role in the production of NO by insulin. However, this MAPK-induced pathway plays a significant role in the development of atherosclerosis in the insulin resistant state. When insulin signaling via PI3K-PDK-PKB is impaired, the MAPK signaling pathway in endothelial cells is enhanced. In the endothelium MAPK activation by insulin results in increased expression of endothelin-1 (ET-1), plasminogen activator inhibitor type-1 (PAI-1), and the adhesion molecules intercellular adhesion molecule-1 (ICAM-1), vascular cell adhesion molecule-1 (VCAM-1), and E-selectin. ET-1 is a potent vasoconstrictor and contributes to endothelial cell dysfunction in the presence of insulin resistance. The increased expression of numerous cell adhesion molecules accelerates the adherence to the endothelium of proinflammatory leukocytes which in turn contributes to the development of atherosclerosis. Therefore, the molecules beneficial to vascular endothelial health that are induced by insulin (eg, NO) are reduced in the insulin resistant state and those that are proatherogenic (eg, ET-1, PAI-1) are increased.
Introduction to the Metabolic Syndrome
The hallmark feature of MetS is insulin resistance. Several other clinical abnormalities are also associated with MetS, including nonalcoholic fatty liver disease (NAFLD), atherosclerosis, oxidative stress, and polycystic ovary syndrome (PCOS). Although obesity, ectopic fat accumulation, and an inflammatory status are central to the pathology of MetS, not all obese individuals develop MetS and not all individuals with MetS are obese. These observations indicate that MetS has a multifactorial etiology that involves a series of complex interactions between a particular individual’s dietary habits, hormonal status, and genetic background (Figure 48-3). Increasing evidence indicates that the risk of MetS can be developmentally induced. Epidemiological studies in humans and animal models of MetS demonstrate an association between poor nutrition during fetal development and an increased risk of adult cardiovascular disease.
FIGURE 48-3: Concept map of the multifactorial interactions resulting in metabolic syndrome. Multiple interacting risk factors lead to global metabolic disruptions typified by insulin resistance, hyperlipidemia, and intravascular proinflammatory status. Progression of the metabolic disturbances in MetS ultimately leads to full-blown type 2 diabetes and eventually the development of cardiovascular diseases (CVD) such as atherosclerosis. Reproduced with permission of themedicalbiochemistrypage, LLC.
Given the complexities of the factors contributing to MetS, numerous health groups in various countries have defined the disorder with slightly different criteria. Some health organizations believe that insulin resistance is the single most important predictor for future development of type 2 diabetes and cardiovascular disease. For this reason MetS is sometimes also called the insulin resistance syndrome. In addition, there are significant differences in ethnic predisposition to the effects of the dysfunctions of MetS that some criteria must be assessed with this in mind. Of particular note is the use of waist circumference as an indicator of obesity. Certain criteria have been established by the American Heart Association and the National Heart, Lung and Blood Institute as defining the metabolic syndrome (Table 48-1).
High-Yield Concept
The metabolic syndrome, MetS (also once referred to as syndrome X), is a disorder that defines a combination of metabolic and cardiovascular risk determinants. These risk factors include insulin resistance, hyperinsulinemia, central adiposity (obesity associated with excess fat deposits around the waist), dyslipidemia, glucose intolerance, hypertension, proinflammatory status, procoagulation together with impaired fibrinolysis, and microalbuminemia.
Genetic Factors in the Metabolic Syndrome
Numerous epidemiological studies have implicated developmental origins in the progression to the metabolic syndrome. There appears to be a clear correlation between poor nutritional status of the mother during fetal development and the potential for increased risk for MetS to her child in later life. These correlations have also been observed in animal studies where prenatal diets in mothers impact their offspring. Excess caloric intake, by the mother, during fetal development as well as excessive nutritional intake by the mother during the preweaning period of postnatal development increases health risks in the child. Children born under these conditions experience increased obesity, adipocyte hypertrophy, reduced activity, insulin resistance, elevated blood pressure, endothelial cell dysfunction, and altered cardiovascular and renal function. These same children will progress to adulthood with impaired glucose tolerance, hyperinsulinemia, dyslipidemia, hypertension, resistance to the anorexic actions of the leptin on the hypothalamus, and develop NAFLD.
High-Yield Concept
There is now a clear link between maternal dietary status and future development of MetS in adult offspring. Likewise, because the risks of MetS in the general population, due to identified inherited polymorphisms is relatively small, the huge increase in the numbers of individuals with the disorder is most likely due to epigenetic changes that occur during early fetal development.
The role of epigenetics in transgenerational disease manifestation has been defined by several studies on the outcomes of children born to parents who experienced famine while pregnant. These children are far more likely to develop diabetes, obesity, and cardiovascular disease than children from parents of similar backgrounds who were not nutritionally deprived. Of striking significance is that the second-generation children (grandchildren of the starved mothers) were more likely to be born with low birth weight regardless of the nutritional status of their mothers. The potential for diet and nutrition to effect epigenetic changes in offspring on multiple generations has been conclusively demonstrated in animal models. In mice, the lack of adequate fetal nutrition results in a reduction in the methylation status of the promoter regions of several transcriptional regulators. Hypomethylation of genes is most often associated with increased transcriptional activation. One transcriptional regulator of key metabolic significance whose promoter has been found to be hypomethylated in offspring of nutritionally deprived mothers is peroxisome proliferator–activated receptor-α (PPARα). PPARα is highly expressed in the liver, skeletal muscle, heart, and kidney. Its function in the liver is to induce hepatic peroxisomal fatty acid oxidation during periods of fasting. Expression of PPARα is also seen in macrophage foam cells and vascular endothelium. Its role in these cells is the activation of anti-inflammatory and antiatherogenic effects.
Metabolic Disruptions in the Metabolic Syndrome
Although MetS is not exclusively associated with type 2 diabetes and the associated insulin resistance, the increasing prevalence of obesity and the associated development of type 2 diabetes places insulin resistance as a major contributor to the syndrome. The role of adipose tissue in MetS stems from the fact that the organ is active at secretion of numerous cytokines, termed adipokines. The most significant adipokines, with respect to MetS, are tumor necrosis factor-α (TNF-α), interleukin-6 (IL-6), leptin, and adiponectin. Leptin has received particular attention of late due to its role in obesity in addition to the fact that recent data indicates that plasma leptin levels are found to be predictive of the potential for cardiovascular pathology.
Many clinicians and researchers believe that insulin resistance underlies the cardiovascular pathologies of MetS. One primary reason for this is the role of insulin in fat homeostasis. A major role of insulin is to induce the storage of fuel. This can be as fat (triglycerides, TG) in adipose tissue or as carbohydrate in the form of glycogen in liver and skeletal muscle. The effect of insulin resistance at the level of fat homeostasis is an increase in circulating TG, referred to as dyslipidemia. Due to insulin resistance there is an increase in the delivery of peripheral fatty acids to the liver which in turn drives hepatic TG synthesis. These TG are then packaged very-low-density lipoprotein (VLDL) which are returned to the circulation. In the context of obesity and diabetes, the proinflammatory state of the vasculature leads to increased ROS production and subsequently oxLDL. As indicated earlier, the uptake of oxLDL by macrophages leads to foam cell production which are highly proinflammatory cells resident in the intimal layer of the vasculature.
An additional role of insulin resistance in the overall cardiac pathology associated with MetS relates to the normal role of insulin in platelet function. In platelets, insulin action leads to an increase in endothelial nitric oxide synthase (eNOS) activity that is due to its phosphorylation by AMPK. Activation of NO production in platelets leads to a decrease in thrombin-induced aggregation, thereby, limiting the procoagulant effects of platelet activation. This response of platelets to insulin function clearly indicates why disruption in insulin action is a major contributing factor in the development of hypertension, atherosclerosis, and MetS.
Taken together, the insulin resistance and its associated negative effects on metabolism, the increased levels of circulating TG, the reduced levels of high-density lipoproteins (HDLs), and hypertension, all contribute to the progression of atherosclerosis. With associated coagulation and fibrinolysis pathologies, the cardiovascular events of MetS can be devastating. Since many of these pathologies can be reversed with proper diet and exercise, lifestyle choices are critical to the development of MetS as well as to the treatment of the disorder.
Obesity and the Metabolic Syndrome
Evidence indicates that over 50% of obese adolescents go on to develop MetS. Also, given the high correlation between insulin resistance and development of MetS, it is not at all surprising that there is a strong link between obesity and MetS. Insulin resistance (identified as type 2 diabetes) is 5 to 6 times more common in individuals with a body mass index (BMI) greater than 30 kg/m2 than in individuals of normal weight.
Although the precise mechanism(s) by which obesity leads to the development of MetS is not fully understood, there are many overlapping pathways of metabolism that are disrupted in obesity that can be identified as important in the progression to MetS. Not the least of which is the role that obesity, in particular visceral obesity, plays in the progression to insulin resistance, the hallmark of MetS.
Testing for the Metabolic Syndrome
A number of clinical laboratory tests have been developed over the years and today are used to screen and/or diagnosis MetS or one of its many pathophysiological entities (Table 48-2). The results of these tests aid physicians in diagnosis, management, and preventative maintenance of MetS.
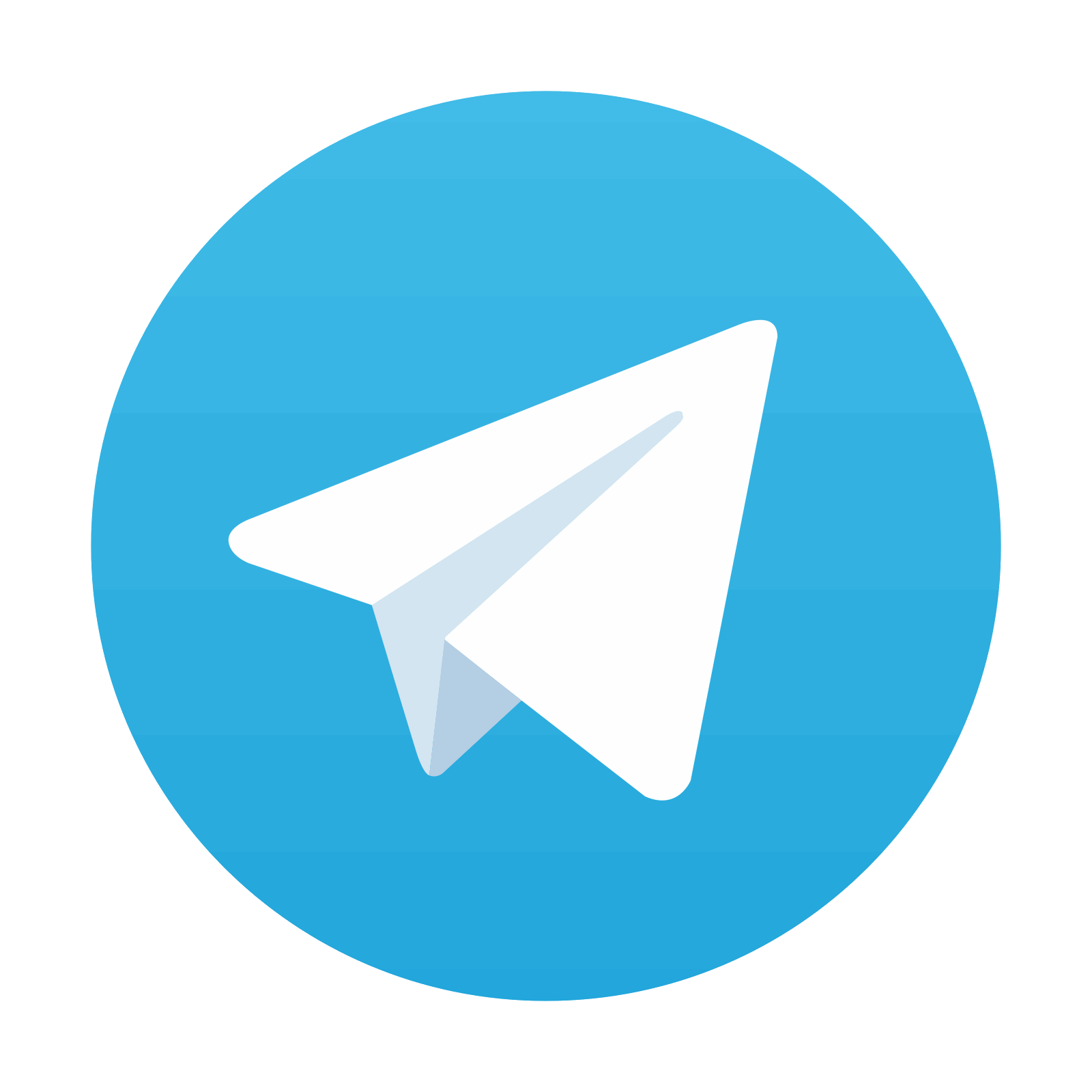
Stay updated, free articles. Join our Telegram channel

Full access? Get Clinical Tree
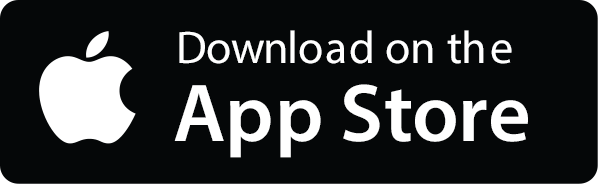
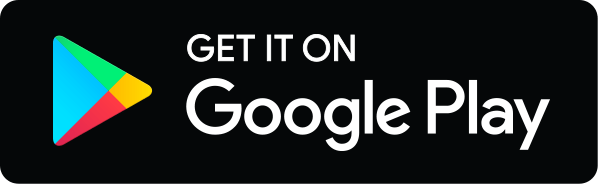