CHAPTER OUTLINE
Gut Hormones and Digestive Processes
Carbohydrate Digestion and Absorption
Protein Digestion and Peptide and Amino Acid Absorption
Lipid Digestion and Absorption
High-Yield Terms
Serous fluid: a watery pale yellow transparent fluid enriched in proteins and water produced by cells of serous glands
Mucus: a viscous mixture covering mucus membranes, produced and secreted by cells of mucus membranes, consists of glycoproteins termed mucins, water, and several other proteins such as antiseptic enzymes and immunoglobulins
Chyme: represents the semifluid mass of partially digested food expelled by the stomach into the duodenum
Enterocytes: columnar epithelial cells of the intestines responsible for nutrient uptake
Goblet cell: glandular epithelial cells that secrete the glycoproteins termed mucins
Chief cell: gastric chief cells are those cells in the stomach that secrete the zymogen and pepsinogen; the parathyroid gland also contains chief cells
Parietal cell: stomach glandular epithelial cells that secrete gastric acid in response to histamine, acetylcholine, and gastrin stimulation
Oxyntic cell: another name for parietal cells
Duct cell: specialized cells lining the pancreatic ducts that secrete bicarbonate
Acinar cell: a cell of an acinus which is any cluster of cells that resembles a many-lobed berry-like structure that is the terminus of an exocrine gland; acinar cells of pancreas are the duct cells that secrete bicarbonate and digestive enzymes
Enteroendocrine cell: specialized endocrine cells found in the intestines and pancreas that produce various gastrointestinal hormones and bioactive peptides
Enterochromaffin-like cell (ECL): neuroendocrine cells that secrete histamine and are found in gastric glands of the gastric mucosa
Gut microbiota: refers to the complex mixture of microorganisms (bacteria, fungi, viruses) that reside within the gastrointestinal tract
Prebiotic: refers to any nondigestible substance in food that stimulates the growth and/or activity of intestinal system bacteria
Probiotic: refers to live bacteria that can be consumed in order to confer a health benefit
Overview of Digestive System
The gastrointestinal (GI) system, with respect to the processes of food ingestion and digestion, comprises the hollow organs that include the oral cavity, the esophagus, the stomach, and the small and large intestines (Table 43-1). In addition to these hollow organs, ingestion and digestion of food requires several accessory glands and other organs that add secretions to the hollow organs of the GI. The primary accessory organs necessary for digestion are the salivary glands present within the oral cavity, the pancreas, and the liver.
The GI organs are separated from each other at key locations by specialized structures termed sphincters. Entry of food from the oral cavity to the esophagus requires passage through the upper esophageal sphincter. The bolus of food then enters the stomach by passage through the lower esophageal sphincter. When food is propelled from the stomach into the duodenum of the small intestine, it passes through the pyloric sphincter. Upon leaving the small intestines at the level of the ileum, digested food passes through the ileocecal valve into the large intestine, also referred to as the colon. Within the colon, waste is stored until it reaches the rectum which triggers nerve impulses to initiate defecation.
Each portion of the GI performs a specialized task in the overall process of digestion. The mouth begins the process by chopping and grinding the food into small pieces while simultaneously lubricating it and initiating the process of fat and carbohydrate digestion. When we swallow, the food is propelled by muscles in the esophagus past the lower esophageal sphincter into the stomach. The process of swallowing food is completely voluntary but all the rest of the events of digestion are carried out via involuntary processes. The primary function of the esophagus is to serve as a conduit from the mouth to the stomach. Once food enters the stomach it can be stored temporarily (in the upper stomach or fundus) or continued to be digested by stomach contractions that churn the food and mix it with acid and proteases in the lower stomach. Digestion continues in the small intestine after food is propelled from the stomach past the pyloric sphincter. The small intestine is also the major site for the absorption of nutrients in the food. The principal function of the large intestine is to reabsorb fluids and electrolytes and to store fecal matter prior to expulsion from the body (Figure 43-1).
FIGURE 43-1: Digestive system overview. Reproduced with permission of themedicalbiochemistrypage, LLC.
Mouth and Esophagus
Even before we place food in our mouth our visual and olfactory senses trigger the release of salivary fluids in preparation for mixing with the food. The primary contents of saliva include water, enzymes, and electrolytes (Table 43-2). Saliva is secreted from the parotid, submandibular, and sublingual glands within the oral cavity. There is a pair of parotid glands and these are the largest of the salivary glands. These glands are located posterior to the mandibular ramus and in front of the mastoid process of temporal bone. The parotid gland secretes saliva through Stensen ducts into the oral cavity. Parotid secretions are mainly serous in nature and facilitate mastication and swallowing. Enzymes present in saliva are also responsible for the digestion of starches.
The submandibular glands are also a pair of glands. These glands are located beneath the lower jaw and superior to the digastric muscles which lie below the body of the mandible. Submandibular secretions are a mixture of both serous fluid and mucus that enters the oral cavity via Wharton’s ducts. Roughly 70% of the saliva present in the oral cavity is derived from the submandibular glands.
High-Yield Concept
On the gross level, the GI system can be divided into the upper GI and the lower GI. The upper GI includes the mouth, esophagus, and the stomach. The lower GI includes the small and large intestines and the rectum.
The sublingual glands are also a pair of glands that reside beneath the tongue and anterior to the submandibular glands. The salivary secretion produced by the sublingual glands is mainly mucus in nature. The sublingual glands do not have striated ducts like the parotid and submandibular ducts. Sublingual fluid is released from 8 to 20 excretory ducts and this fluid constitutes approximately 5% of saliva entering the oral cavity.
Chewing food is designed to chop, mash, and mix the contents of the mouth. The mixing of food and saliva represents the initial process of digestion. The action of α-amylase, on starch, releases glucose into the mouth. Glucose is sweet tasting which enhances the flavor of the food making it much more palatable and rewarding. When we swallow, the food is passed through the pharynx past the upper esophageal sphincter into the esophagus. Muscles in the esophagus then propel the food past the lower esophageal sphincter and into the stomach. The esophagus passes through the posterior mediastinum in the thorax and enters the abdomen through a hole in the diaphragm at the level of the tenth thoracic vertebrae. The esophagus is divided into three distinct sections referred to as cervical, thoracic, and abdominal. As a consequence of the actions of the inferior pharyngeal constrictor muscle, entry to the esophagus occurs only when swallowing or vomiting.
Stomach
The role of the stomach in overall digestive processes is complex and unique. The unique digestive functions of the stomach are related to its structure and to the types of secretory products produced by various specialized exocrine cells. The major secretory product of the stomach is gastric acid, HCl. In addition to acid, specialized cells of the stomach secrete the hormone ghrelin, water, mucus, bicarbonate, numerous anions and cations, intrinsic factor, and the zymogen pepsinogen. The stomach is tasked with storing ingested food, mixing ingested material with digestive juices and gastric acid, and then slowly emptying its contents past the pyloric sphincter into the duodenum.
High-Yield Concept
The process of swallowing food is a completely voluntary event. Once food enters the rest of the GI system all of the subsequent events of digestion occur via completely involuntary processes that are controlled by the enteric, parasympathetic, and sympathetic nervous systems.
In addition to being responsible for digesting food, the stomach also absorbs water-soluble and lipid-soluble substances. The digested contents of the stomach are referred to as chyme. Chyme is the semifluid material produced by the conversion of large solid particles into smaller particles via the combined peristaltic movements of the stomach and contraction of the pyloric sphincter. Chyme is generated by the propulsive, grinding, and retropulsive movements associated stomach peristalsis. The combination of the grinding action of the antrum, the retropulsion, and the squeezing of the chime through the pyloric sphincter into the duodenum is required for the emulsification of dietary fat and this plays an important role in the overall digestion and absorption of fat.
The stomach is anatomically divided into 3 major segments termed the fundus, the corpus (body), and the antrum (Figure 43-2). In addition, the stomach comprises two functional areas, the oxyntic gland and the pyloric gland areas. The oxyntic gland area comprises 80% of the stomach, encompassing both the fundus and the corpus. The pyloric gland area encompasses the antrum. An area of the stomach just distal to the lower esophageal sphincter is sometimes referred to as the cardia but whether it represents a normal or pathophysiological domain is an area of debate. The upper region of the stomach is called the fundus and this is where the bolus of food can be temporarily stored for up to an hour. The corpus is the largest segment of the stomach and the mucosa of this region contain many distinct exocrine and enteroendocrine cell types. The mucosal secretory cells of the fundus and corpus include mucus cells, parietal (oxyntic) cells, chief cells, and enteroendocrine cells. Chief cells are also called zymogen or peptic cells and are the cells that secrete the digestive enzymes, the pepsins. The primary function of mucus secretion by stomach epithelial cells is to protect the epithelium against shear stress and gastric acid. There are five or six distinct types of enteroendocrine cells in the mucosal layer of the stomach which includes enterochromaffin-like cells (ECL) that secrete histamine.
FIGURE 43-2: Organization of the major stomach segments. The 3 major structural areas are denoted by the dashed lines. The cardia is indicated in parentheses due to the fact that there is controversy as to whether or not this constitutes a normal functional region of the stomach. The 2 major functional areas, the oxyntic gland area and the pyloric gland area, are denoted in the large brackets. The major cell types in these 2 functional domains are also indicated. ECL, enterochromaffin-like cells that secrete histamine; D, D cells that secrete somatostatin; G, G cells that secrete gastrin. Reproduced with permission of themedicalbiochemistrypage, LLC.
The last segment of the stomach is the antrum. The antrum is devoid of oxyntic glands and thus the acid secreting parietal cells. The antrum (also called the pyloric antrum) contains both exocrine and enteroendocrine cells. The antrum enteroendocrine cells include G cells which secrete gastrin and D cells which secrete somatostatin. Both of these hormones are involved in regulated gastric acid production and secretion. Gastrin stimulates gastric acid secretion, whereas somatostatin inhibits gastrin release and, therefore, parietal cell acid secretion.
High-Yield Concept
In addition to digestion, the stomach also plays a critical role in the sensations of appetite and satiety. These latter functions are related to the hormone ghrelin as well as to vagal nerve responses to the stomach stretching in response to uptake of the bolus of food from the esophagus.
The wall of the stomach is composed of 4 distinct layers. The gastric mucosa is the layer that lines the inside of the stomach. The subsequent layers include the submucosa, the muscularis externa, and then the serosa. The mucosal layer of the stomach is composed of an epithelium that harbors the various secretory glands. The submucosa consists of fibrous connective tissue that separates the mucosa from the muscularis externa. The muscularis externa of the stomach contains 3 layers of smooth muscle, whereas the rest if the GI tract contains only 2 layers of smooth muscle. The serosa is the outer layer of the stomach consisting of connective tissue that is continuous with the peritoneum.
The mucosal lining of the corpus of the stomach contains 2 major types of specialized glands, the pyloric and oxyntic glands. The oxyntic glands are composed of parietal cells that secrete gastric acid, exocrine cells (chief) that secrete pepsinogen, and mucus secreting cells. The acid secreting parietal cells are also called oxyntic cells. In addition, parietal cells of the fundus and corpus secrete intrinsic factor. Intrinsic factor is a glycoprotein that binds to vitamin B12 within the small intestine allowing it to be absorbed. Initially vitamin B12 is protected from gastric acid by binding salivary haptocorrin. After passing into the duodenum the haptocorrin is degraded by pancreatic proteases and the vitamin is then bound to intrinsic factor. The pyloric glands are located in the antrum segment of the stomach and contain cells that are responsible for secretion of gastrin (G cells), somatostatin (D cells), and mucus.
Gastric acid production and secretion is the major exocrine function of the stomach (Figure 43-3). The production and secretion of gastric acid (HCl), by oxyntic cells, as well as the zymogen pepsinogen, by chief cells, is controlled by both hormonal- and nerve-mediated processes. The nervous system components that impact the gastrointestinal tract (see below) are complex and consist of autonomic, parasympathetic (vagus), sympathetic (prevertebral ganglia), and enteric nerve fibers possessing both efferent (motor) and afferent (sensory) neurons. The enteric nervous system functions autonomously all along the GI tract as well as interacting with the CNS via vagal and prevertebral ganglia.
FIGURE 43-3: Gastric acid secretion by oxyntic cells. Stimulation of parietal cells by acetylcholine or gastrin binding triggers the release of intracellular calcium which in turn triggers the fusion of tubulovesicles containing the H+,K+-ATPase (proton pump) with the parietal cell membrane allowing transport of protons into the canaliculus of the cell and out into to lumen of the stomach. Histamine binding to parietal cells activates adenylate cyclase resulting in increased cAMP and activation of PKA which also triggers tubulovesicle fusion to the parietal cell membrane. CCK-2, cholecystokinin-2 (and gastrin) receptor; M3, muscarinic acetylcholine (ACh) receptor 3; H2, histamine receptor 2; CFTR, cystic fibrosis transmembrane conductance regulator. Reproduced with permission of themedicalbiochemistrypage, LLC.
The principal stimulants for acid secretion are histamine, gastrin, and acetylcholine (ACh) released from postganglionic enteric neurons. Each of the 3 stimulators of acid secretion has different levels of functional importance, but histamine is thought to be the primary stimulus. Histamine is synthesized from the amino acid histidine via the action of histidine decarboxylase (see Chapter 30). Histamine is released from ECL cells in response to gastrin binding to these cells in fundal glands. The released histamine then binds to H2 receptors on parietal cells. The H2 receptor is a Gs-type GPCR that activates adenylate cyclase, leading to elevation of intracellular cAMP concentrations and activation of PKA. With respect to gastric acid production, PKA activation leads to phosphorylation of cytoskeletal proteins involved in transport of the H+/K+-ATPase (proton pump) from the cytoplasm to the apical plasma membrane of the parietal cell.
The gastric H+/K+-ATPase is a member of the P-type ATPase family (see Chapter 7). The H+K+-ATPase is the molecular pump of gastric acid secretion and it is solely responsible for the secretion of hydrogen ions into the lumen of the gastric glands and ultimately the stomach. The H+/K+-ATPase transports one hydrogen ion (H+) out of the cytoplasm of the parietal cell in exchange for one potassium ion (K+) obtained from the lumen of the gastric gland. The concentration of hydrogen ion in the lumen of gastric glands is such that the pH is on the order of 0.8, making it the most acidic environment in the human body.
The hydrogen ions are generated within the parietal cell from the dissociation of water. Water and CO2 are combined to form carbonic acid via the action of carbonic anhydrase. Carbonic acid rapidly ionizes to H+ and bicarbonate ion. This bicarbonate is then transported to the blood, across the parietal cell basolateral membrane, in exchange for chloride ion. The chloride ions are transported into the lumen of the gastric glands via the action of anion exchangers of the solute carrier family. The gastric chloride exchanger is encoded by the SLC26A9 gene. Within the lumen of the gastric gland the hydrogen ions and chloride ions combine to form gastric acid (HCl).
Like all P-type ATPase’s, the H+/K+-ATPase is able to transport ions against a concentration gradient by utilizing the energy derived from the hydrolysis of ATP. During ATP hydrolysis a phosphate is transferred to the H+/K+-ATPase resulting in a conformational change in the pump. The conformational changes that occur to the H+/K+-ATPase in response to phosphorylation and dephosphorylation ensure that ion transport can only occur unidirectionally at any given point in time.
Gastric acid secretion occurs via 3 distinct phases, in response to ingestion of food, termed the cephalic, gastric, and intestinal phases. Even though ingestion of food stimulates a large increase in gastric acid production, there is a small, referred to as basal, level of acid production at all times.
The cephalic phase involves the CNS and is the result of responses to thinking of food, smelling food, and tasting food. These CNS responses transmit impulses to both parietal and enteroendocrine G cells (gastrin secreting) of the stomach via the vagal nerve. The vagal nerve endings contain acetylcholine (ACh) which, when released, binds to muscarinic-type (M3) receptors on parietal cells directly stimulating acid production and secretion. Acetylcholine can also indirectly stimulate gastric acid secretion by inhibiting the release of somatostatin (see below) through activation of M2 and M4 muscarinic receptors on D cells.
Vagal nerve endings on the stomach also contain gastrin-releasing peptide (also known as bombesin or neuromedin B). The release of gastrin-releasing peptide triggers G cells to release gastrin. Gastrin then binds to receptors on parietal cells indirectly stimulating acid secretion. Gastrin exists in 2 major forms: little gastrin (17 amino acids) and big gastrin (34 amino acids), both of which result from a single precursor protein of 101 amino acid. Both forms of gastrin function by binding to the cholecystokinin-2 receptor (CCK2 or CCKB). The C-terminal tetrapeptide of both gastrins and cholecystokinin (CCK) are identical and possess all the biological activities of both gastrin and CCK. The cephalic phase accounts for 40% of total gastric acid secretion.
The distension (stretching) of the stomach as a result of food intake represents the gastric phase. The gastric phase of acid production is also stimulated by digestive products such as peptides. The distension of the stomach results in the stimulation of mechanoreceptors. Mechanoreceptor activation then causes parietal cell stimulation via both enteric (direct) and vagal nerve reflexes. Afferent and efferent impulses from the vagus nerve in the stomach mediate vago-vagal reflexes within the brain via the dorsal vagal complex. The gastric phase accounts for 50% of total gastric acid secretion.
The intestinal phase of gastric acid secretion only accounts for about 10% of the total. This phase is stimulated by protein digestion products within the duodenum. Circulating amino acids stimulate gastric acid secretion through their actions on the parietal cells.
Just as the production of gastric acid is physiologically significant with respect to digestion, the inhibition of gastric acid secretion is also physiologically significant. The secretion of gastric acid should only occur during digestion as it can damage the gastric and duodenal mucosa if inappropriately secreted or secreted in excess. Dysregulation of acid secretion is a significant cause of ulcerative conditions, therefore, the body has developed an elaborate system for regulating the amount of acid secreted by the stomach. Gastric luminal pH is a sensitive regulator of acid secretion and protein in digested food is an excellent buffer. The buffering capacity of food maintains the luminal pH above 3. However, when the stomach is empty, or if acid production is excessive gastric pH can fall below 3. When the pH falls, enteroendocrine D cells of the antrum secrete somatostatin which inhibits gastrin release from oxyntic cells. Acidification of the duodenal lumen also triggers mechanisms to inhibit gastric acid production. Under low pH conditions duodenal enteroendocrine S cells release secretin which inhibits the release of gastrin (Clinical Box 43-1).
Pancreas
The pancreas is a highly specialized organ found behind the stomach and it is surrounded by the small intestine, liver, and spleen (Figure 43-4). The organ is about six inches long and is largest at the end attached to the duodenum. This widest part is called the head of the pancreas, the middle section is called the neck and the body of the pancreas, and the thin end is called the tail.
CLINICAL BOX 43-1: DISTURBANCES IN GASTRIC ACID HOMEOSTASIS
Increases in both basal and stimulated gastric acid production are the leading causes of ulcers in the mucosal lining of the gastrointestinal tract. These ulcers are collectively referred to as peptic ulcers. Four times as many peptic ulcers arise in the duodenum (called duodenal ulcers) versus the stomach itself (called gastric ulcers). The major cause of disrupted gastric acid homeostasis (accounting for as much as 70%-90% of such ulcers) is infection with Helicobacter pylori (H pylori), a helical-shaped bacterium that lives in the acidic environment of the stomach. Around 4% of all gastric ulcers are caused by malignant tumors called gastrinomas. Stress ulcers are most often found in the proximal stomach and result from mucosal ischemia and altered mucosal defense. Although duodenal ulcers are the result of increased acid secretion, gastric ulcers are more often associated with normal or decreased basal and stimulated acid production. Gastric ulcers are, therefore, most often caused by altered gastric mucosal defense which explains the propensity for NSAID (nonsteroidal anti-inflammatory drug)-induced ulcers to occur in the stomach. Gastric ulcers are classified into four groups according to their location and concomitant association with duodenal ulcer. Type I ulcers are found in the corpus and are generally characterized by low acid secretion, particularly at night. Type II ulcers occur in the antrum and are characterized by low, normal, or high acid secretion. Type III ulcers occur in close proximity to the pylorus and are associated with high acid output and duodenal ulcers. Type IV ulcers occur in the cardia and are characterized by low acid secretion. Treatment for gastric ulcers involves removing the injurious agent (e.g., NSAIDs or H pylori) and inhibiting acid secretion. Antacids were the first therapeutic approach for ulcers. Unfortunately in order to neutralize luminal acid adequately, they need to be taken frequently which results in noncompliance and adverse effects. Although antacids with high neutralizing capacity, given after meals and at bedtime, can accelerate ulcer healing, pain is not significantly. The histamine H2 receptor antagonist (H2RA), cimetidine, was the first in this class of new peptic ulcer treatments. H2RAs block both histamine-stimulated acid secretion and that induced by gastrin, which functions via stimulation of histamine release from ECL cells. The development of H+/K+-ATPase inhibitors (proton pump inhibitors, PPIs), such as omeprazole, revolutionized the therapeutic management of gastric acid. PPIs directly inhibit the acid pump and therefore, they are capable of reducing both basal and stimulated gastric acid secretion independent of stimulus. PPIs are much more effective than H2RAs at reducing gastric pH as well as being more effective in aiding the healing of duodenal ulcers and preventing their recurrence. In addition to these more common conditions that cause abnormal gastric acid production resulting in ulcers, there are several uncommon conditions. The best characterized of these latter conditions is Zollinger-Ellison syndrome, ZES. ZES results from gastrin-producing tumors (gastrinomas) that result in hypersecretion of gastric acid. In any patient manifesting with refractory erosive esophagitis, multiple peptic ulcers, ulcers in the distal duodenum or jejunum, and recurrent ulcers after acid-reducing surgery the possibility of ZES should be highly suspected. Patients with a family history of multiple endocrine neoplasia type 1 (MEN1), or any of the endocrinopathies associated with MEN1, that are presenting with multiple peptic ulcers should also be suspected of having ZES since approximately 25% of patients with ZES have MEN1. MEN1 is an autosomal dominant disorder characterized by pancreatic endocrine tumors, pituitary adenomas, and hyperparathyroidism. The PPIs are used as the therapies of choice in the treatment of ZES, although very high doses are sometimes required to reduce the acid hypersecretion sufficiently. Recently, gastroesophageal reflux disease (GERD) has become the most important acid-related clinical disorder. The pathogenesis of GERD involves acid in the wrong anatomical location, not excess acid production. Numerous treatments for GERD have been attempted but surgery is the most effective. Aside from surgery, the use of PPIs is the most used drugs for treatment of GERD.
FIGURE 43-4: Structure of the pancreas. Used with permission from Widmaier EP, Raff H, Strang KT. Vander’s Human Physiology: The Mechanisms of Body Function. 11th ed. New York: McGraw-Hill; 2008).
High-Yield Concept
The response of the stomach to the effects of ACh, gastrin, and histamine occur via a phenomenon referred to as potentiation. Potentiation is the effect observed where the response to 2 stimulants is greater than the effect of either stimulant alone.
The pancreas is composed primarily of 2 distinct types of secretory cells, endocrine and exocrine. The endocrine cells secrete a variety of hormones such as glucagon and insulin which have been discussed in several of the metabolic biochemistry chapters as well as in Chapters 46, 46, and 49. The exocrine pancreas is involved in the secretion of numerous enzymes (Table 43-3), electrolytes, and other substances involved in digestion. The exocrine pancreas has ducts that are arranged in clusters called acini (singular acinus). Pancreatic digestive secretions are expelled into the lumen of the acinus, and then accumulate in intralobular ducts that drain into the main pancreatic duct. The main pancreatic duct (pancreatic duct of Wirsung) joins the common bile duct coming from the gallbladder forming the ampulla of Vater. The ampulla is connected to the duodenal papilla through which the pancreatic juice, also containing bile, enters the small intestine.
In addition to enzymes described in Table 43-3, the pancreas also secretes various cations, anions, water, and bicarbonate ion into the small intestine. Together with the pancreatic enzymes, the secretions constitute what is referred to as pancreatic juice. The cells of the pancreas tasked with electrolyte (eg, bicarbonate) secretion are called duct cells. Pancreatic bicarbonate is critical to neutralizing the gastric acid entering the small intestine from the stomach. The production and secretion of pancreatic bicarbonate is essentially the same as for the process described for gastric acid secretion by parietal cells. Like parietal cell bicarbonate production, pancreatic bicarbonate is dependent on the enzyme carbonic anhydrase. The content of bicarbonate in pancreatic juice (around 115 mEq/L) is approximately 5-times higher than what is normally present in plasma. This high concentration of bicarbonate makes the pH of pancreatic juice around 8.0.
Exocrine glands of the pancreas also contain cells termed goblet cells. Goblet cells are tasked with the production and secretion of mucus into the pancreatic juice. Goblet cells are found nearest the distal end of the pancreatic ducts and they can constitute as much as 25% of the epithelial cells at the distal end of the main pancreatic duct. Pancreatic mucus provides lubrication to the ducts as well as mechanical protection. In addition, the mucins (high-molecular-weight glycoproteins, see Chapter 39) present in pancreatic mucus serve an immunologic function by binding pathogens and interacting with immune cells.
Control of the secretory function of the acinar and duct cells of the pancreas is exerted via nervous and humoral mechanisms. The primary neural stimulus for pancreatic cell secretion of enzymes and bicarbonate is ACh released from postganglionic parasympathetic nerve endings. The primary hormonal triggers for acinar cell secretion are gastrin, cholecystokinin (CCK), and secretin. Gastrin is secreted by gastric G cells whereas, CCK is secreted by duodenal enteroendocrine I cells and secretin by S cells. All 3 hormones are secreted in response to the ingestion of food.
Cholecystokinin and ACh are the most important regulators of acinar protein secretion. Secretin is the most important regulator of pancreatic duct cell secretion of bicarbonate. The action of CCK on acinar cells exhibits a biphasic response in that induced secretion occurs at a particular dose of hormone but secretion is inhibited at higher doses. The CCKA receptor has the highest affinity for CCK and this receptor exists in both low affinity and high affinity states in acinar cells. Low concentrations of CCK activate the high affinity state receptor which induces acinar cell secretion, whereas high concentrations of CCK activate the low affinity state receptor leading to inhibition of secretion. ACh activates acinar cell secretion by binding to muscarinic (M3) receptors.
High-Yield Concept
Lysophosphatidylcholine (LPC, lyso-PC) is a bioactive pro-inflammatory lipid generated by the action of sPLA2. In cases of acute pancreatitis it is believed that pancreatic sPLA2 is inappropriately activated within the pancreatic ducts resulting in inflammation in the tissue. In acute pancreatitis the leakage of pancreatic enzymes into the blood increases. Measurement of plasma levels of pancreatic lipase and α-amylase can be of diagnostic value in cases of suspected pancreatitis.
As indicated earlier, the hormone secretin is involved in the response to acidification of the contents of the intestines. When the pH of intestinal fluid falls below around 4.5, S cells of the duodenum release secretin. At the level of the stomach secretin inhibits gastric acid production by inhibiting the release of gastrin and GIP. At the level of the pancreas, secretin stimulates pancreatic duct cells to secrete bicarbonate which when released into the duodenum increases the pH.
Intestine
The intestines comprise a long hollow organ that runs from the stomach to the anus. The intestines are divided into three major anatomical and functional segments termed the small intestine, the large intestine, and the rectum. The small intestine is further divided into three functionally distinct segments. These segments, from the stomach to the large intestines are the duodenum, jejunum, and ileum. The small intestines are around 20 ft in length with an average diameter of 1 in. The large intestine, which is commonly referred to as the colon, is also subdivided into distinct segments. Beginning with the segment attached to the ileum these segments are the cecum, ascending colon, transverse colon, descending colon, and sigmoid colon. The large intestines are around 5 ft in length with an average diameter of 3 in.
Similar to the layers of the stomach, the intestines are layered from inside the lumen to out with glandular epithelium and muscularis mucosa (together comprising the mucosal layer), submucosa, muscularis externa (only 2 layers whereas the stomach has 3), and lastly the serosa (Figure 43-5). The muscularis externa, which is composed of both longitudinal and circular smooth muscles, provides the continuous peristalsis of the intestines that promotes the movement of digested food along the length of the gut to the rectum and the movement of digested material out of and along the gut.
FIGURE 43-5: Macroscopic view of a section of the duodenum. Reproduced with permission of themedicalbiochemistrypage, LLC.
The primary function of the intestines is the digestion of food and the absorption of nutrients. Almost all of the nutrients in food, the amino acids, sugars, fats, and vitamins, are absorbed through the small intestines. The duodenum is the segment of the small intestine that secretes the pancreatic digestive enzymes and bicarbonate, and bile via the ampulla of Vater. The primary secretory products of the duodenum are cholecystokinin, secretin, and GIP. Absorption of nutrients is the primary function of the jejunum, while the ileum is responsible for absorption of vitamin B12 and bile salts.
The primary function of the large intestines is the resorption of water from food wastes, thus creating fecal matter (the stool). The stool is then stored in the large intestines until it reaches the rectum where nerve impulses then stimulate the urge to defecate. In addition to water, the large intestines reabsorb sodium and any nutrients that may have escaped primary digestion in the ileum. The large intestine is also the site where gut bacteria (microbiota) are found. The importance of gut bacteria to human physiology is significant (see below), but in the context of digestion these microorganisms produce some vitamins and important nutrients and they digest molecules that cannot be degraded by human enzymes. Bacterial metabolism also accounts for the production of gases within the intestines.
Within the small intestine, the mucosal layer contains large numbers of invaginations termed villi. In addition, there are microvilli which dramatically increase the surface area of the small intestines and are the areas of the mucosal epithelium where nutrients are absorbed. In addition to the villi and microvilli, the small intestine contains numerous glands formed by pocket-like invaginations in the epithelium called crypts (crypts of Lieberkühn). Along the entire length of the intestinal glandular epithelium are goblet cells that produce mucus, which lubricates the intestines as well as protects the tissue from digestive enzymes. The other major cell types in intestinal crypts and villi are the enterocytes and the enteroendocrine cells (I, K, and L). Enterocytes are the cell types that absorb the nutrients in the gut. These cells also harbor the digestive enzymes that continue to digest food as it is being absorbed. Enterocytes contain a number of enzymes that are important for digestion, but these enzymes remain associated with the brush-border membranes and are not released into the lumen of the gut. These enzymes include enteropeptidase, disaccharidases, aminopeptidases, dipeptidases, nucleotidases, and nucleosidases.
Gallbladder
The gallbladder is a small organ lying just beneath the liver between the costal margin and the lateral margin of the rectus abdominis muscle. The organ is divided into 3 sections called the fundus, body, and neck. The neck of the gallbladder tapers where it connects to the biliary tree via the cystic duct. The cystic duct then joins the common hepatic duct forming the common bile duct. The gallbladder is the storage site for bile acids that were produced from cholesterol by the liver (Chapter 27). The consumption of food, particularly lipid- and protein-rich foods, stimulates the release of CCK from duodenal enteroendocrine I cells which then enters the circulation and binds to receptors on the gallbladder. The binding of CCK to gallbladder cells induces pulsatile secretion of bile into the common bile duct which then meets with the pancreatic duct at the ampulla of Vater where they are secreted into the duodenum. Bile salts are involved in the emulsification of lipids in the intestines aiding their digestion by the various pancreatic and duodenal lipases. Unused bile salts are returned to the gallbladder through the distal ileum and the portal circulation.
Gut hormones and digestive processes
The gastrointestinal system secretes numerous hormones and neuroendocrine molecules that are critical to the overall processes of digestion as indicated earlier. However, many of these same factors play important roles in the regulation of appetite and overall feeding behaviors. As a consequence of these activities many of the gut peptides and hormones and their receptors are targets for therapeutic intervention in obesity and diabetes. Many of the activities of the gut peptides and hormones are discussed in detail in Chapter 44 and only briefly outlined here in Table 43-4.
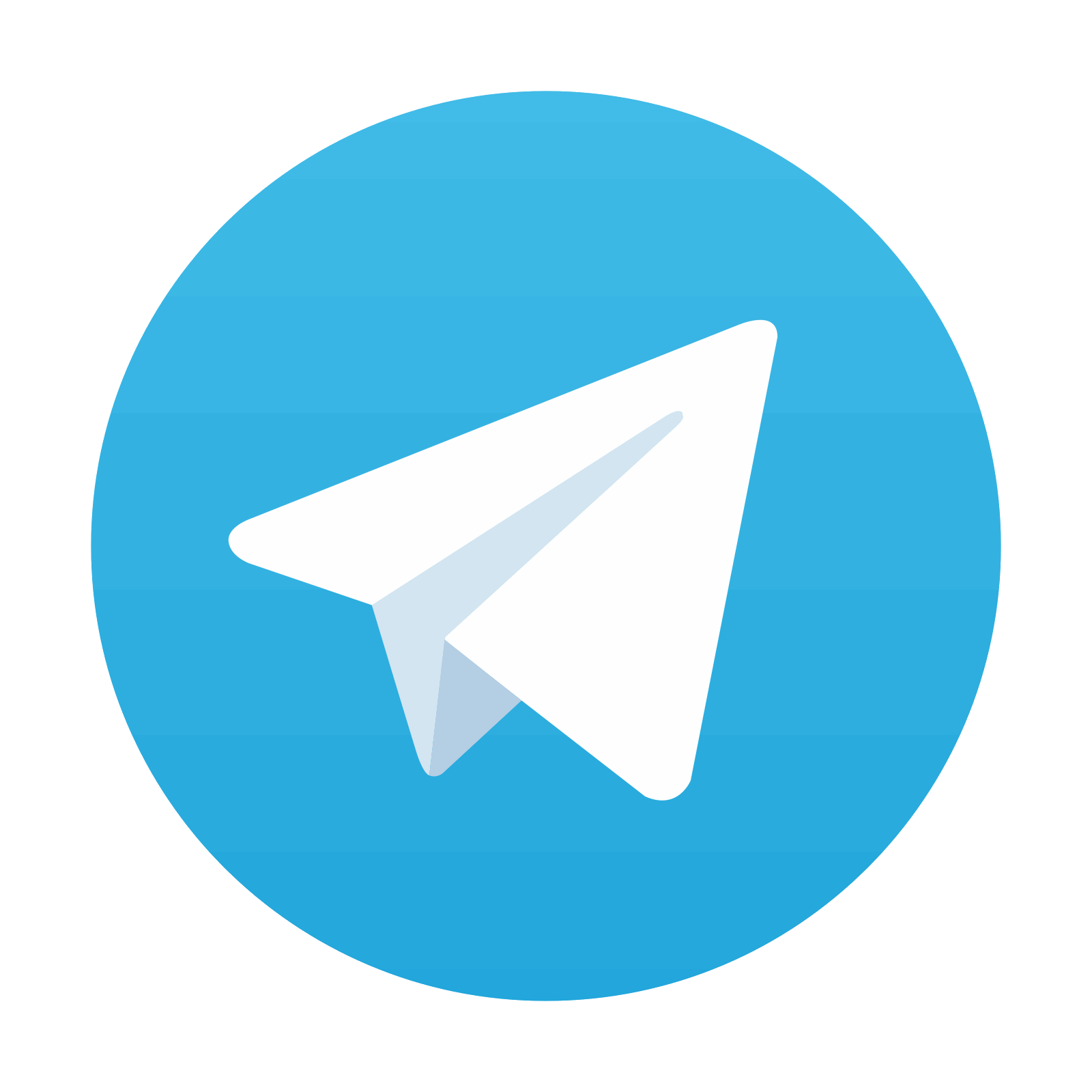
Stay updated, free articles. Join our Telegram channel

Full access? Get Clinical Tree
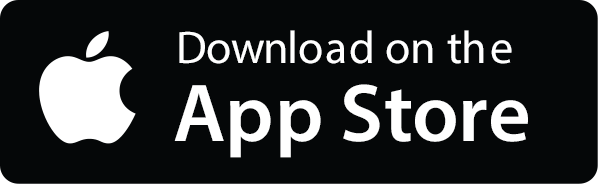
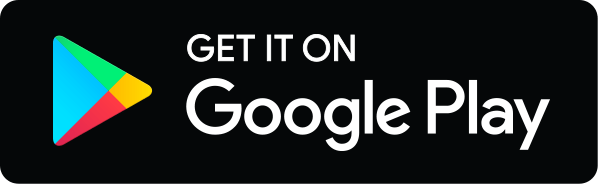