CHAPTER OUTLINE
High-Yield Terms
Basement membrane: a thin sheet of fibers that underlies the epithelium, which lines the cavities and surfaces of organs including skin, or the endothelium lining the interior surface of blood vessels
Basal lamina: one of the layers of the basement membrane
Ground substance: the noncellular components of the extracellular matrix (ECM) composed of a complex mixture of glycosaminoglycans (GAGs), proteoglycans, and glycoproteins
Glycosaminoglycan: a family of large polymers containing a repeat disaccharide structure, most often attached to a core protein forming a proteoglycan
Mucopolysaccharidosis: any of a large family of inherited diseases that result from defects in lysosomal hydrolases responsible for the degradation of GAGs
The Extracellular Matrix
A substantial portion of the volume of tissues is extracellular space, which is largely filled by an intricate network of macromolecules constituting ECM. The ECM is composed of 2 major classes of biomolecules: glycosaminoglycans (GAGs), most often covalently linked to protein forming the proteoglycans, and fibrous proteins which include collagen, elastin, fibronectin, and laminin. These components are secreted locally and assembled into the organized meshwork that is the ECM.
Connective tissue (Figure 39-1) refers to the matrix composed of the ECM, cells (primarily fibroblasts), and ground substance that is tasked with holding other tissues and cells together forming the organs. Ground substance is a complex mixture of GAGs, proteoglycans, and glycoproteins (primarily laminin and fibronectin), but generally does not include the collagens. In most connective tissues, the matrix constituents are secreted principally by fibroblasts, but in certain specialized types of connective tissues, such as cartilage and bone, these components are secreted by chondroblasts and osteoblasts, respectively.
FIGURE 39-1: Components of typical connective tissue. In addition to the extracellular matrix, typical connective tissues contain cells (primarily fibroblasts) all of which is surrounded by ground substance. Mescher AL. Junqueira’s Basic Histology Text and Atlas. 13th ed. New York: McGraw-Hill; 2013.
Collagens
Collagens, the major protein comprising the ECM, were introduced in Chapter 5. There are at least 30 different collagen genes dispersed through the human genome (Table 39-1). These 30 genes generate proteins that combine in a variety of ways to create over 28 different types of collagen fibrils. Types I, II, and III collagens are the most abundant, accounting for nearly 90% of all the collagen in the body. Type IV collagen forms a 2-dimensional reticulum and is a major component of the basal lamina. Collagens are predominantly synthesized by fibroblasts but epithelial cells also synthesize these proteins. Given the abundance and complexity of the collagens it is not surprising that a number on inherited disorders in connective tissue result from defects in any of the collagen genes. The Ehlers-Danlos syndromes (EDS) and the various types of osteogenesis imperfect (OI) are the most common forms of connective tissue disorders resulting from defective collagen genes (see Clinical Box 5-1).
The nomenclature for collagens involves the chain composition and the numbering of the collagen gene encoding that particular α-chain. For example, type I collagen proteins are encoded by the COL1A1 and COL1A2 genes with 2 of the triple helix proteins encoded by one gene and 1 by the other. Therefore, type I collagen fibrils are denoted [α1(I)]2[α(I)], where the Roman numeral designates the fibril as type I.
The ECM is not only critical for connecting cells together to form the tissue, but it is also a substrate upon which cell migration is guided during the process of wound healing as well as during embryonic development. In addition, the ECM is responsible for the relay of environmental signals to the cell surface.
The fundamental higher-order structure of collagens is a long and thin-diameter rod-like protein (Figure 39-2). Type I collagen, for instance, is 300-nm long, 1.5-nm in diameter, and consists of 3 coiled subunits composed of two α1(I) chains and one α2(I) chain. Each chain consists of 1050 amino acids wound around each other in a characteristic right-handed triple helix. Collagen proteins are rich in glycine, proline, and lysine, with many of the proline and lysine R-groups being modified. A characteristic feature of collagens is the spacing of glycine residues within the triple helical portion of the protein. There is a repeating sequence containing the glycine, denoted as (Gly-X–Y)n where X and Y can be any amino acid but many of the X position amino acids are proline and many of the Y positions are hydroxyproline.
FIGURE 39-2: Higher-order structural features of a typical collagen fibril. Each individual polypeptide chain is twisted into a left-handed triple helix comprising the Gly-X-Y residues. The triple helical structure of processed collagen is formed by right-handed coiling of 3 polypeptides into a superhelix. Reproduced with permission of themedicalbiochemistrypage, LLC.
All collagens are synthesized as longer precursor alpha (α) proteins called procollagens (Figure 39-3). Type I procollagen contains an additional 150 amino acids at the N-terminus and 250 at the C-terminus. These prodomains are globular and form multiple intrachain disulfide bonds. The disulfides stabilize the proprotein allowing the triple helical section to form. Collagen fibers begin to assemble in the ER and Golgi complexes. The signal sequence is removed and numerous modifications take place in the collagen chains. Specific proline residues are hydroxylated by prolyl 4-hydroxylase and prolyl 3-hydroxylase. Specific lysine residues also are hydroxylated (hLys) by lysyl hydroxylase. Both prolyl hydroxylases are absolutely dependent upon vitamin C as a cofactor. Glycosylations of the O-linked type also occurs on many of the hLys residues during Golgi transit. Following completion of the processing, the procollagens are secreted into the extracellular space where extracellular enzymes remove the prodomains. The collagen molecules then polymerize to form collagen fibrils. Accompanying fibril formation is the oxidation of certain lysine residues by the extracellular enzyme lysyl oxidase forming reactive aldehydes. These reactive aldehydes form specific cross-links between 2 chains, thereby stabilizing the staggered array of the collagens in the fibril.
FIGURE 39-3: Synthesis of collagen. Each collagen is composed of a α-chain encoded by 1 of the 30 different α-collagen genes. Synthesis, hydroxylation, and glycosylation of the procollagen protein occurs during transit through the ER. Final assembly into structural fibrils occurs after procollagen is secreted into the ECM. Mescher AL. Junqueira’s Basic Histology Text and Atlas. 13th ed. New York: McGraw-Hill; 2013.
Overall, the various collagens are grouped according to the major structures formed through subunit interactions. These groupings consist of the fibril-forming, the sheet-forming, and the anchoring (or linking) collagens. For example, type I collagen, the most abundant collagen, is a member of the fibril-forming group and forms large fibrils that constitute the framework for the dermis, tendons, and organ capsules. Although there are 28 known different types of collagen, those that have been best characterized either by structure or by the three main classifications are outlined in Table 39-2.
Elastin and Fibrillin
The ECM of tissues that undergo significant stretching and/or bending contains significant quantities of the protein elastin found in a specialized type of fibril called elastic fibers. Elastic fibers are composed of large masses of cross-linked elastin interspersed with another ECM protein called fibrillin. The walls of large arteries are particularly abundant with elastin (and thus elastic fibers) which allows them to undergo continual deformation and reformation during changes in intravascular pressure. The lungs and the skin are additional organs whose tissues are rich in elastin and elastic fibers.
Elastin is synthesized as the precursor, tropoelastin. Tropoelastin has 2 major types of alternating domains. One domain is hydrophilic and rich in Lys (K) and Ala (A), while the other domain is hydrophobic and rich in Val (V), Pro (P), and Gly (G) where these amino acids are frequently contained in repeats of either VPGVG or VGGVG. The hydrophobic domains of elastin are responsible for its elastic character. Tropoelastin is expressed, then secreted as a mature protein into the extracellular matrix and accumulates at the surface of the cell. After secretion and alignment with ECM fibrils, numerous K residues are oxidized by lysyl oxidases, a reaction which initiates cross-linking of elastin monomers. This process of elastin cross-linking, induced by lysyl oxidases, is the same as occurs in the cross-linking of collagens. Although lysyl oxidase activity promotes elastin cross-linking, the process is unique in that 3 lysine-derived aldehydes (allysyl) cross-link with an unmodified lysine forming a tetrafunctional structure called a desmosine. The highly stable cross-linking of elastin is what ultimately imparts the elastic properties to elastic fibers (Figure 39-4).
FIGURE 39-4: Molecular basis of elasticity. Subunits of the glycoprotein elastin are joined by covalent bonds formed among lysine residues of different subunits, catalyzed by lysyl oxidase. This produces an extensive and durable cross-linked network of elastin. (Such bonds give rise to the unusual amino acids desmosine and isodesmosine.) Each elastin molecule in the network has multiple random-coil domains which expand and contract; this allows the entire network to stretch and recoil like a rubber band. Mescher AL. Junqueira’s Basic Histology Text and Atlas. 13th ed. New York: McGraw-Hill; 2013.
Defects in the elastin gene are found associated with the inherited disorder known as Williams-Beuren syndrome, which is characterized by connective tissue dysfunction that plays a causative role in the supravalvular aortic stenosis typical of this disorder. Defective elastin is also associated with a group of skin disorders called cutix laxa. In these disorders the skin has little to no elastic character and hangs in large folds.
The other major protein in elastic fibers is fibrillin 1. Fibrillin 1 serves as the scaffold in elastic fibers upon which cross-linked elastin is deposited. Inherited defects in the fibrillin 1 gene result in the connective tissue disorder called Marfan syndrome, MFS (Clinical Box 39-1).
Fibronectin
Fibronectin is a major fibrillar glycoprotein of the ECM where its role is to attach cells to a variety of ECM types. Fibronectin is also found in a soluble compact nonfunctional form in the blood. The transformation from the compact form to the extended fibrillar form of fibronectin is referred to as fibrillogenesis. This transformation requires the application of mechanical forces generated by cells. This occurs as cells bind and exert forces on fibronectin through transmembrane receptor proteins of the integrin family (Figure 39-5).
CLINICAL BOX 39-1: MARFAN SYNDROME
Marfan syndrome (MFS) is an autosomal dominant disorder affecting the connective tissue. The symptoms of MFS are the result of inherited defects in the extracellular matrix glycoprotein fibrillin 1. The fibrillin 1 gene (symbol FBN1) is composed of 65 exons encoding a profibrillin protein of 2871 amino with a processed molecular weight of approximately 350,000 Da. There are 5 distinct structural regions in the fibrillin 1 protein. The largest of these structural regions comprises 75% of the total protein and is composed of 46 EGF-like repeats. These EGF-like repeats are cysteine-rich domains that were first identified in the epidermal growth factor (EGF) protein. Almost all of the EGF-like domains are encoded by individual exons. There are 8 additional cysteine motifs that share homology to a domain first identified in transforming growth factor–β1-binding protein (TGFBR1) called a TB domain. The fibrillin protein has also been shown to bind calcium. In addition to the defects in connective tissue, there are systemic effects in the cardiovascular, musculoskeletal, and ophthalmic systems and the integument in MFS patients. There are certain diagnostic criteria that allow for differentiating MFS from several related disorders such as congenital contractural arachnodactyly, which is caused by mutations in a highly homologous gene identified as FBN2 encoding the fibrillin 2 protein. The cardinal manifestations of MFS are tall stature with dolichostenomelia (condition of unusually long and thin extremities) and arachnodactyly (abnormally long and slender fingers and toes), joint hypermobility and contracture, deformity of the spine and anterior chest, mitral valve prolapse, dilatation and dissection of the ascending aorta, pneumothorax, and ectopia lentis (displacement of the crystalline lens of the eye). Correct diagnosis of MFS relies on the presence of a combination of manifestations in skeletal, cardiovascular, pulmonary, and ocular organ systems. Individuals with MFS are taller at all ages than would be predicted based on their kinship. The tall stature is the result of overgrowth of the long bones, which leads to the characteristic features of MFS such as disproportionately long legs, arms, and digits. As indicated above, this phenotype is called dolichostenomelia where one diagnostic feature is that the arm span will exceed the body height. The overgrowth of the tubular bones leads to deformity of the anterior chest. This can result in the ribs pushing the ribs either in (pectus excavatum) or out (pectus carinatum) or in on one side and out on the other. The skull is often elongated (termed dolichocephaly), the mandible underdeveloped with the palate narrow and highly arched, and the face is long and narrow. Because of ligament laxity the joints are hypermobile and there is often joint pain. Mitral valve prolapse (MVP) is the most common finding in children with MFS. The prolapse is due to redundancy of the valve tissue, elongation of the valve annulus (valve ring), and elongation of the chordae tendineae (these are the tendons that connect the papillary muscles to the tricuspid and mitral valves). MVP will progress to severe mitral regurgitation which requires surgical intervention usually before 10 years of age. Progressive dilation of the proximal portions of both the aorta and main pulmonary arteries occurs in MFS and are highly important diagnostic and prognostic indicators. The combination of the deformity of the anterior chest and pectus excavatum results in a reduction in lung volume, which can lead to severe restrictive pulmonary deficits. Spontaneous pneumothorax occurs in 4% to 5% of MFS patients. Obstructive sleep apnea is a common symptom in MFS. The ocular hallmark of MFS is displacement of the lens from the center of the pupil (ectopia lentis). Because of the presence of this symptom in a high percentage of MFS patients, displacement of the lens in the absence of any traumatic event should lead to a consideration of MFS. Later in life many MFS patients will suffer from cataracts and/or glaucoma.
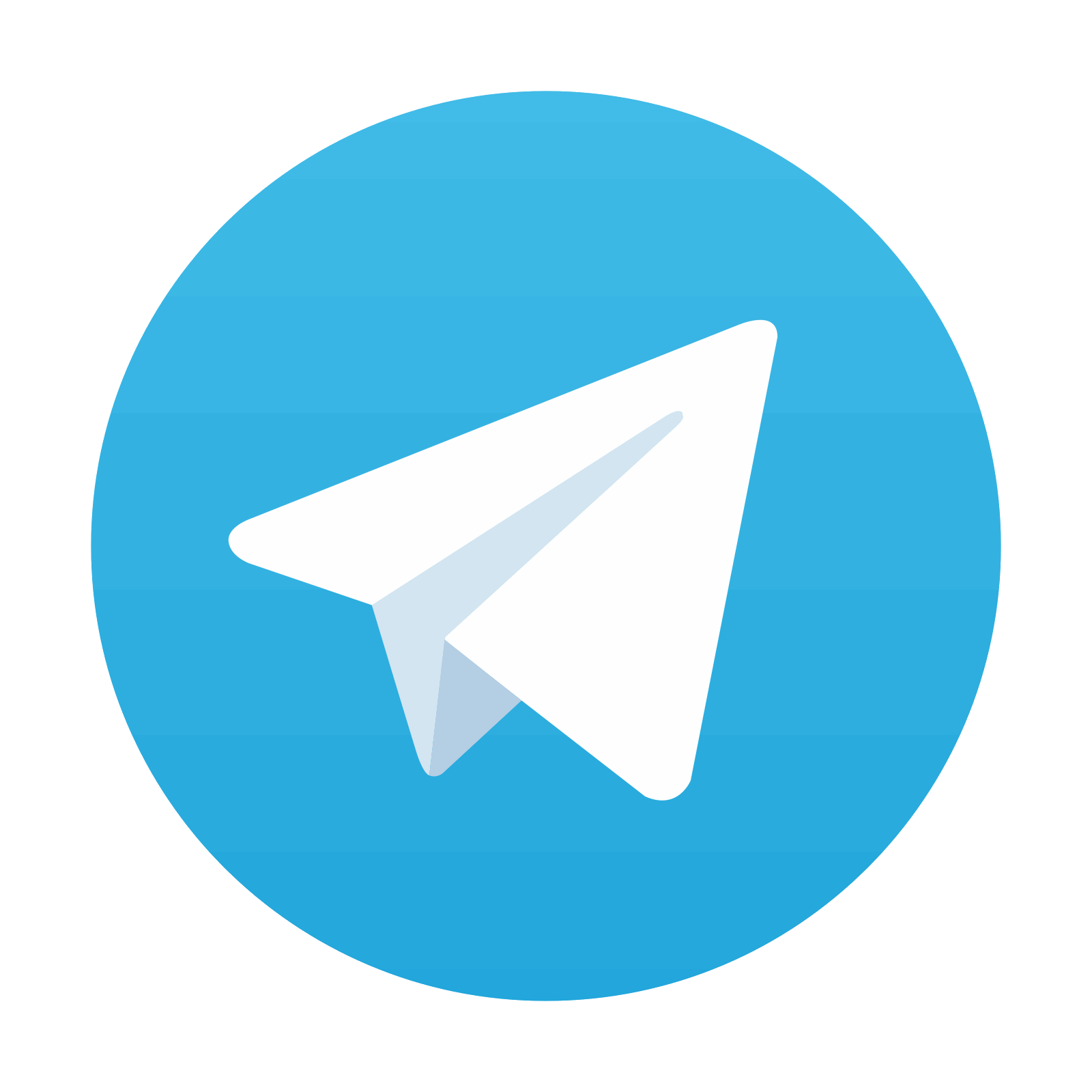
Stay updated, free articles. Join our Telegram channel

Full access? Get Clinical Tree
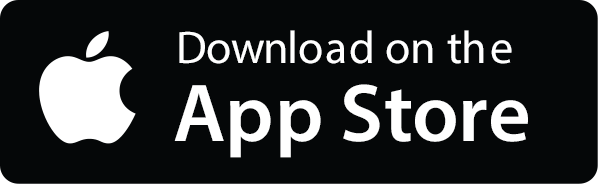
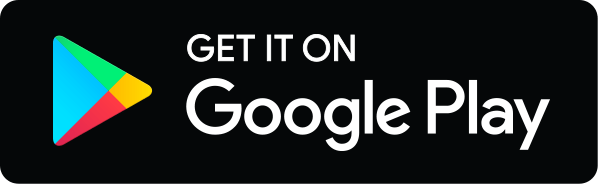