BACTERIOLOGY
The Enterobacteriaceae are among the largest bacteria, measuring 2 to 4 μm in length with parallel sides and rounded ends. Forms range from large coccobacilli to elongated, filamentous rods. The organisms do not form spores or demonstrate acid-fastness.
Rods are large
The cell wall, cell membrane, and internal structures are morphologically similar for all Enterobacteriaceae, and follow the cell plan described in Chapter 21 for Gram-negative bacteria. Components of the cell wall and surface, which are antigenic, have been extensively studied in some genera and form the basis of systems dividing species into serotypes. The outer membrane lipopolysaccharide (LPS) is called the O antigen. Its antigenic specificity is determined by variation in the sugars that form the long terminal polysaccharide side chains linked to the core polysaccharide and lipid A. Cell surface polysaccharides may form a well-defined capsule or an amorphous slime layer and are termed the K antigen (from the Danish Kapsel, capsule). Motile strains have protein peritrichous flagella, which extend well beyond the cell wall and are called the H antigen. Many Enterobacteriaceae have surface pili (fimbriae), which are antigenic proteins, but not part of formal typing systems.
O = LPS
K = polysaccharide capsule
H = flagellar protein
Facultative growth is rapid
Enterobacteriaceae grow readily on simple media, often with only a single carbon energy source. Growth is rapid under both aerobic and anaerobic conditions, producing 2 to 5 mm colonies on agar media and diffuse turbidity in broth after 12 to 18 hours of incubation. All Enterobacteriaceae ferment glucose, reduce nitrates to nitrites, and are oxidase negative.
CLASSIFICATION
Genus and species designations are based on phenotypic characteristics, such as patterns of carbohydrate fermentation, and amino acid breakdown. The O, K, and H antigens are used to further divide some species into multiple serotypes. These types are expressed with letter and number of the specific antigen, such as Escherichia coli O157:H7, the cause of numerous foodborne outbreaks. These antigenic designations have been established only for the most important species and are limited to known antigenic structures. For example, many species lack capsules and/or flagella. In recent years, DNA and rRNA homology comparisons have been used to validate these relationships and establish new ones. The genera containing the species most virulent for humans are Escherichia, Shigella, Salmonella, Klebsiella, and Yersinia. Other less common but medically important genera are Enterobacter, Serratia, Proteus, Morganella, and Providencia.
Biochemical characteristics establish species
Antigenic characters define serotypes within species
TOXINS
In addition to the LPS endotoxin common to all Gram-negative bacteria, some Enterobacteriaceae also produce protein exotoxins, which act on host cells by damaging membranes, inhibiting protein synthesis, or altering metabolic pathways. The end result of these actions may be cell death (cytotoxin) or a physiologic alteration, the net effect of which depends on the function of the affected cell. For example, enterotoxins act on intestinal entero-cytes, causing the net secretion of water and electrolytes into the gut to produce diarrhea. Although these toxins are most strongly associated with E coli, Shigella, and Yersinia, others with the same or very similar actions have now been discovered in other species. Toxins found in another species may differ slightly in protein structure and genetic regulation but still have the same biologic action on host cells. Details of these toxins are discussed later in this chapter in relation to their prototype species.
All have LPS
Cytotoxins kill cells
Enterotoxins cause secretion and diarrhea
DISEASES CAUSED BY ENTEROBACTERIACEAE
EPIDEMIOLOGY
Most Enterobacteriaceae are primarily colonizers of the lower gastrointestinal tract of humans and animals. Many species survive readily in nature and live freely anywhere that water and minimal energy sources are available. In humans, they are the major facultative components of the colonic bacterial flora and are also found in the female genital tract and as transient colonizers of the skin. Enterobacteriaceae are scant in the respiratory tract of healthy individuals; however, their numbers may increase in hospitalized patients with chronic debilitating diseases. Escherichia coli is the most common species of Enterobacteriaceae found among the indigenous flora, followed by Klebsiella, Proteus, and Enterobacter species. Salmonella and Shi-gella species are not considered members of the resident microbiota, although carrier states can exist. Shigella and Salmonella serovar Typhi are strict human pathogens with no animal reservoir. An overview of these infections is illustrated in Figure 33–1.
FIGURE 33–1. Enterobacteriaceae disease overview. The external sources of infection are frequently from animal sources, but some pathogens are strictly human (Shigella, Salmonella ser. Typhi). Endogenous flora is the source of opportunistic infection particularly urinary tract infection (UTI). Bacteria from any source entering the blood may cause endotoxic shock.
Present in nature and the intestinal tract
Shigella and STyphi are found only in humans
PATHOGENESIS
Opportunistic Infections
Enterobacteriaceae are often poised to take advantage of their common presence in the environment and human microbiota to produce disease when they gain access to normally sterile body sites. Surface structures such as pili are known to aid this process for some species and surely do for many others. Once in deeper tissues, their ability to persist and cause injury is little understood except for the action of LPS endotoxin and the species known to produce exotoxins or capsules. The prototype opportunistic infection is the UTI, in which Enterobacteriaceae gain access to the urinary bladder due to minor trauma or instrumentation. Strains able to adhere to uroepithelial cells can persist and multiply in the nutrient-rich urine, sometimes spreading through the ureters to the renal pelvis and kidney (pyelonephritis). Likewise, mucosal or skin trauma can allow access to soft tissues and aspiration to the lung when the relevant site is colonized with Enterobacteriaceae.
Colonization presents opportunity when defense barriers open
UTI follows access and adherence to bladder mucosa
Intestinal Infections
Salmonella, Shigella, Yersinia enterocolitica, and certain strains of E coli are able to produce disease in the intestinal tract. These intestinal pathogens have invasive properties or virulence factors such as cytotoxins and enterotoxins, which correlate with the type of diarrhea they produce. In general, the invasive and cytotoxic strains produce an inflammatory diarrhea called dysentery with white blood cells (WBCs) and/or blood in the stool. The enterotoxin-producing strains cause a watery diarrhea in which fluid loss is the primary pathophysiologic feature. For a few species, the intestinal tract is the portal of entry, but the disease is systemic as a result of spread of bacteria to multiple organs. Enteric (typhoid) fever caused by Salmonella enterica ser. Typhi is the prototype of this form of infection.
Cell destruction causes dysentery
Enterotoxins cause watery diarrhea
Enteric fever is a systemic illness
Regulation of Virulence
In addition to adherence pili, LPS, and exotoxins, the Enterobacteriaceae produce a myriad of other virulence factors to cause disease. Many of them are deployed in a complex and sequential fashion in response to environmental signals (temperature, iron, calcium) or as yet unknown factors. Some members have injection (type III or IV) secretion systems that target human cells by delivering a syringe-like injection of multiple virulence factors into the cytoplasm of host cells.
Virulence factors respond to signals
Secretion systems inject factors
The genes for these factors, located in the chromosome, plasmids, or both, are controlled by interactive regulators that seem to produce each virulence factor exactly when it is needed. The genes themselves are often organized into clusters, which include the genes for the effector molecules as well as their regulatory proteins. This is particularly true for complex characteristics such as invasiveness, which involve multiple sequential steps. Some of these gene clusters are pathogenicity islands (PAIs) acquired from another bacterium in the genetically distant past. In particular, PAIs are associated with injection secretion systems, where they contain the structural genes for the injection apparatus, as well as the virulence factors injected.
Virulence genes are organized into gene clusters
Expression may be stimulated by environmental cues
PAIs contain multiple genes
IMMUNITY
Little is understood about immunity to the broad range of opportunistic infections caused by Enterobacteriaceae. Antibody directed against an LPS core antigen has been shown to provide a degree of protection against Gram-negative endotoxemia, but the diversity of antigens and virulence factors among the Enterobacteriaceae is too great to expect broad immunity. Immunity to intestinal infection is generally short-lived and is discussed where it is relevant to specific intestinal pathogens.
Immunity is short-lived
ENTEROBACTERIACEAE: CLINICAL ASPECTS
MANIFESTATIONS
The Enterobacteriaceae produce the widest variety of infections of any group of microbial agents, including two of the most common infectious states, UTI and acute diarrhea. Urinary tract infections are manifested by dysuria and urinary frequency when infection is limited to the bladder, with the addition of fever and flank pain when the infection spreads to the kidney. Enterobacteriaceae are by far the most common cause of UTIs, and the most common species involved is E coli.
UTI and acute diarrhea are most common
DIAGNOSIS
Culture is the primary method of diagnosis; all Enterobacteriaceae are readily isolated on routine media under almost any incubation conditions. Special indicator media such as MacConkey agar are commonly used in primary isolation to speed separation of the many species. For example, the common pathogens E coli and Klebsiella typically ferment lactose rapidly, producing acid (pink) colonies on MacConkey agar, whereas the intestinal pathogens Salmonella and Shigella do not. Separation of the intestinal pathogens from all the other Enterobacteriaceae in stool requires highly selective media designed solely for this purpose. These are discussed as they relate to individual pathogens. Improved understanding of the genetic and molecular basis for virulence has led to the development of direct nucleic acid and immunodiagnostic techniques for direct detection of toxin, adhesin, and invasin proteins or their genes in clinical material (eg, stool). These methods once too expensive for use in clinical laboratories are beginning to emerge as primary diagnostic tools.
MacConkey agar demonstrates lactose fermentation
Selective media required for Salmonella and Shigella in stools
Gene probes allow direct detection
TREATMENT
Antimicrobial therapy is crucial to the outcome of infections with members of the Entero-bacteriaceae. Unfortunately, combinations of chromosomal and plasmid-determined resistance render them the most variable of all bacteria in susceptibility to antimicrobial agents. They are usually resistant to high concentrations of penicillin G, erythromycin, and clindamycin, but may be susceptible to the broader spectrum β-lactams, aminoglycosides, tetracycline, chloramphenicol, sulfonamides, quinolones, nitrofurantoin, and the polypeptide antibiotics. Because the probability of resistance varies among genera and in different epidemiologic settings, the susceptibility of any individual strain must be determined by antimicrobial susceptibility tests. Typical patterns of resistance for some of the more common Enterobacteriaceae appear in Appendix 23–1.
Susceptibility to antimicrobials is highly variable
ESCHERICHIA COLI
BACTERIOLOGY
Most strains of E coli ferment lactose rapidly and produce indole. These and other biochemical reactions are sufficient to separate it from the other species. There are over 150 distinct O antigens and a large number of K and H antigens, all of which are designated by number. The antigenic formula for serotypes is described by linking the letter (O, K, or H) and the assigned number of the antigen(s) present (eg, O111:K76:H7).
Serotypes use O, H, K antigens
PILI
Pili play a role in virulence as mediators of attachment to human epithelial surfaces. They show marked tropism for different epithelial cell types, which is determined by the availability of their specific receptor on the host cell surface. Most E coli express type 1 or common pili. Type 1 pili bind to the D-mannose residues commonly present on epithelial cell surfaces and thus mediate binding to a wide variety of cell types. More specialized pili are found in subpopulations of E coli. P pili bind to digalactoside (Gal–Gal) moieties on kidney cells and erythrocytes of the P blood group. Pili that mediate binding to enterocytes are found among the diarrhea-causing E coli and are specific to the pathogenic type as shown in Figure 33–2 and listed in Table 33–1. Escherichia coli also causes diarrhea in animals, and different sets of pili exist with host-specific tropism for their enterocytes. The receptor(s) for the enteric pili are not known in detail but include glycolipids and glycoproteins on the enterocyte surface.
FIGURE 33–2. Antigenic structure of Escherichia coli. The O antigen is contained in the repeating polysaccharide units of the lipopolysaccharide (LPS) in the outer membrane of the cell wall. The Η antigen is the flagellar protein. The K antigen is the polysaccharide capsule present in some strains. Most E coli have type 1 (common) hair-like pili extending from the surface. Some E coli have specialized P colonization factor antigens (CFAs), or bundle-forming pili (Bfp), as well as type 1 pili.
TABLE 33–1 Characteristics of Pathogenic Enterobacteriaceae
Type 1 pili bind mannose
P pili bind kidney cells
Pili of diarrhea strains bind enterocytes
The genetics of pilin expression is complex. The genes are organized into multicistronic clusters that encode structural pilin subunits and regulatory functions. Pili of different types may coexist on the same bacterium, and their expression may vary under different environmental conditions. Type 1 pilin expression can be turned “on” or “off” by inversion of a chromosomal DNA sequence containing the promoter responsible for initiating transcription of the pilin gene. Other genes control the orientation of this switch.
Type 1 has on-off switch
TOXINS
Escherichia coli can produce every kind of protein exotoxin found among the Enterobac-teriaceae. These include a pore-forming cytotoxin, inhibitors of protein synthesis, and a number of toxins that alter messenger pathways in host cells. The α-hemolysin is a pore-forming cytotoxin that inserts into the plasma membrane of a wide range of host cells in a manner similar to streptolysin O (Chapter 25) and Staphylococcus aureus α-toxin (Chapter 24). The toxin causes leakage of cytoplasmic contents and eventually cell death. The more recently discovered cytotoxic necrotizing factor (CNF) is often produced in concert with α-hemolysin. CNF is an A-B toxin that disrupts G proteins regulating signaling pathways in the cell cytoplasm with multiple effects including cytoskeleton rearrangement and apoptosis.
α-Hemolysin is pore-forming cytotoxin
CNF disrupts intracellular signaling
Shiga toxin (Stx) is named for the microbiologist who discovered Shigella dysenteriae, and this toxin was once believed to be limited to that species. It is now recognized to exist in at least two molecular forms released by multiple E coli and Shigella strains on lysis of the bacteria. In the years after the discovery of this toxin, the term Shiga toxin was reserved for the original toxin, and others were called Shiga-like. In this book, the term Stx is used for all molecular variants that have the same mode of action regardless of the species under consideration. Stx is an A–B type toxin. The B unit directs binding to a specific glycolipid receptor (Gb3) present on eukaryotic cells and is internalized in an endocytotic vacuole. Inside the cell, the A subunit crosses the vacuolar membrane in the trans-Golgi network, exits to the cytoplasm, and enzymatically modifies the ribosome site (28S-RNA of 60S subunit) where amino acyl tRNA binds. This alteration blocks protein synthesis, leading to cell death (Figure 33–3). This action is very similar to the plant toxin ricin.
FIGURE 33–3. Stx (Shiga) toxin. The A–B toxin binds to the cytoplasmic membrane, enters in an endocytotic vacuole, and enters the Golgi network. Exiting to the cytoplasm, it combines at ribosome sites involved with tRNA binding. The result is interference with protein synthesis.
Shiga toxin is produced by Shigella and E coli
Inhibits protein synthesis by ribosomal modification
Labile toxin (LT) is also an A-B toxin. Its name relates to the physical property of heat lability, which was important in its discovery, and contrasts with the heat-stable toxin (ST) also produced by E coli. The B subunit binds to the cell membrane, and the A subunit catalyzes the ADP-ribosylation of a regulatory G protein located in the membrane of the intestinal epithelial cell. This inactivation of part of the G protein complex causes permanent activation of the membrane-associated adenylate cyclase system and a cascade of events, the net effect of which depends on the biologic function of the stimulated cell. If the cell is an enterocyte, the result is the stimulation of chloride secretion out of the cell and the blockage of NaCl absorption. The net effect is the secretion of water and electrolytes into the bowel lumen. The structure and action of LT are nearly identical with that already described for cholera toxin (CT), but LT is less potent than CT.
LT ADP-ribosylates G protein
Adenylate cyclase stimulation similar to cholera
Stable toxin is a small peptide that binds to a glycoprotein receptor, resulting in the activation of a membrane-bound guanylate cyclase. The subsequent increase in cyclic GMP concentration causes an LT-like net secretion of fluid and electrolytes into the bowel lumen.
ST stimulates guanylate cyclase
E COLI OPPORTUNISTIC INFECTIONS
URINARY TRACT INFECTION
Epidemiology
Escherichia coli accounts for more than 90% of the more than 7 million cases of cystitis and 250 000 of pyelonephritis estimated to occur in otherwise healthy individuals every year in the United States. Urinary tract infections are much more common in women, 40% of whom have an episode in their lifetime, usually when they are sexually active. The reservoir for these infections is the patient’s own intestinal E coli flora, which contaminate the peri-neal and urethral area. In individuals with urinary tract obstruction or instrumentation, environment sources assume some importance.
Perineal flora is reservoir of common cystitis
Pathogenesis
Relatively minor trauma or mechanical disruptions can allow bacteria colonizing the periurethral area brief access to the urinary bladder. These bacteria originally derived from the fecal flora are frequently present in the bladder of women immediately after sexual intercourse. In most instances, they are purged by the flushing action of voiding, but may persist to cause a UTI, depending on host and bacterial factors. Host situations that violate bladder integrity (urinary catheters) or that obstruct urine outflow (enlarged prostate) allow the bacteria more time to attach, multiply, and cause injury. However, most UTIs are in otherwise healthy women. Here, bacterial virulence factors are important, and E coli is the prototype UTI pathogen. Fewer than 10 E coli serotypes account for the majority of UTI cases, and these UTI serotypes are not the most common ones in the fecal flora. These E coli with enhanced potential to produce UTI are called uropathogenic E coli (UPEC).
Minor trauma admits E coli to the bladder
UPEC cause most UTIs
The ability of UPEC to produce UTI begins with type 1 pili which are the most important for both periurethral and bladder colonization. The tip of these pili attaches to mannose moieties presented by membrane proteins (uroplakins) in the transitional epithelium of the bladder. Other pili such as P pili may add to the strength of this attachment although P pili are more important for upper urinary tract disease. Their Gal–Gal receptor is most abundant in the renal pelvis and kidney where P pili facilitate pyelonephritis. Escherichia coli possessing P pili are a minor percentage of the fecal flora (<20%), but the proportion of P+ strains progressively rises with the level of UTI up to 70% in pyelonephritis isolates. Motility driven by flagellar motors also plays a role both in access to the bladder and swimming up the ureter to the kidney. Obviously, adherence and motility are at cross purposes, but UPEC are able to reciprocally regulate these features. Using the on/off switching of type 1 pili, subpopulations of UPEC can alternate between swimming and adherent phases. Another feature is the ability of UPEC to invade superficial epithelial cells. The raft-like clusters formed by this maneuver are felt to aid persistence against the periodic flushing of the bladder. Once established, LPS and the production of other virulence factors such as α-hemolysin and CNF cause injury. Spread to the bloodstream leads to LPS-induced septic shock. The adherence aspects of UPEC are illustrated in Figure 33–4.
FIGURE 33–4. Urinary tract infection due to Escherichia coli. The urinary bladder, perineal mucosa, and short female urethra are shown. E coli from the nearby rectal flora have colonized the perineum, utilizing binding by type 1 (common) pili. E coli with P pili are also present but are of no use at this site. A. A few E coli have gained access to the bladder owing to mechanical disruptions such as sexual intercourse or instrumentation (catheters). Note that receptors for the P pili not present on the perineal mucosa are found on the surface of bladder mucosal cells. B. During voiding, the bladder has expelled the E coli, which have only type 1 pili. The P pili-containing bacteria remain behind due to the strong binding to the P (Gal-Gal) receptor C. The remaining E coli have multiplied and are causing a UTI (cystitis) with inflammation and hemorrhage. In some cases, the bacteria ascend the ureter to cause pyelonephritis in the kidney where the P (Gal–Gal) receptor is most abundant. WBCs, white blood cells.
Type 1 pili adhere to periurethral and bladder cells
P pili prominent in pyelonephritis
OTHER OPPORTUNISTIC INFECTIONS
Meningitis
Escherichia coli is one of the most common causes of neonatal meningitis; many features of which are similar to group B streptococcal disease. The pathogenesis involves vaginal E coli colonization of the infant via ruptured amniotic membranes or during childbirth. Failure of protective maternal IgM antibodies to cross the placenta and the special susceptibility of newborns surely play a role. Fully 75% of cases are caused by strains possessing the K1 capsular polysaccharide that contains sialic acid and is structurally identical to the group B polysaccharide of Neisseria meningitidis, another cause of meningitis.
Infection from vaginal flora such as group B streptococcus
K1 capsule identical to meningococcus
With the exception of UTIs, extraintestinal E coli infections are uncommon unless there is a significant breach in host defenses. Opportunistic infection may follow mechanical damage such as a ruptured intestinal diverticulum, trauma, or involve a generalized impairment of immune function. The virulence factors involved are likely the same as with UTI (eg, pili, α-hemolysin), but have been less specifically studied. Failure of local control of infection can lead to spread and eventually Gram-negative septic shock. A significant proportion of blood isolates have the K1 surface polysaccharide. The particular diseases that result depend on the sites involved.
Non-UTI infections require some breach of defenses
E COLI INTESTINAL INFECTIONS
Diarrhea-causing E coli are conveniently classified according to their virulence properties as enterotoxigenic (ETEC), enteropathogenic (EPEC), enteroinvasive (EIEC), enterohemorrhagic (EHEC), or enteroaggregative (EAEC). Each group causes disease by a different mechanism, and the resulting syndromes usually differ clinically and epidemiologically. For example, ETEC and EIEC strains infect only humans. Food and water contaminated with human waste and person-to-person contact are the principal means of infection. A summary of the pathogenesis of infection, clinical syndromes, and epidemiology of infection for each enteropathogen is shown in Table 33–1.
Multiple pathogenic mechanisms have their own epidemiologic and clinical features
ENTEROTOXIGENIC E COLI
Epidemiology
Enterotoxigenic E coli (ETEC) is the most important cause of traveler’s diarrhea in visitors to developing countries. ETEC also produce diarrhea in infants native to these countries, where they are a leading cause of morbidity and mortality during the first 2 years of life. Repeated bouts of diarrhea caused by ETEC and other infectious agents are an important cause of growth retardation, malnutrition, and developmental delay in third-world countries where ETEC are endemic. ETEC disease is rare in industrialized nations, although recent outbreaks suggest that it may be underestimated.
Traveler’s diarrhea affects children of developing countries
Transmission is by consumption of food and water contaminated by infected human or convalescent carriers. Uncooked foods such as salads or marinated meats and vegetables are associated with the greatest risk. Direct person-to-person transmission is unusual, because the infecting dose is high. Animals are not involved in ETEC disease.
High dose in uncooked foods required
Pathogenesis
ETEC diarrhea is caused by strains of E coli that produce LT and/or ST enterotoxins in the proximal small intestine. ST seems to be more potent than LT and strains that elaborate both cause the most severe illness. Adherence to surface microvilli mediated by multiple variants of colonizing factor (CF) pili is essential for the efficient delivery of toxin to the target enterocytes. The genes encoding the ST, LT, and the CF pili are borne in plasmids. A single plasmid can carry all three sets of genes. The bacteria remain on the surface, where the adenylate cyclase-stimulating action of the toxin(s) creates the flow of water and electrolytes from the enterocyte into the intestinal lumen. The mucosa becomes hyperemic but is not injured in the process. There is no invasion or inflammation.
LT and/or ST cause fluid outpouring in small intestine
CF pili are required
Immunity
Although there can be more than one episode of diarrhea, infections with ETEC can stimulate immunity. Travelers from industrialized nations have a much higher attack rate than adults living in the endemic area. This natural immunity is presumably mediated by sIgA specific for LT and CFs. The small ST peptides are nonimmunogenic. The disease is of very low incidence in breastfed infants, underscoring the protective effect of maternal antibody and the importance of transmission by contaminated food and water.
sIgA to LT and CFs may provide some protection
ENTEROPATHOGENIC E COLI
Epidemiology
Enteropathogenic E coli (EPEC) strains were first identified as the cause of explosive outbreaks of diarrhea in hospital nurseries in the United States and Great Britain during the 1950s. The link to E coli was established on epidemiologic grounds alone using serotyping of stool isolates, no small task. The World Health Organization still recognizes a group of 12 EPEC serotypes. The disease seems to have disappeared in industrialized nations, although it may be underestimated because of the difficulty of diagnosis. In developing countries throughout the world, EPEC account for up to 20% of diarrhea in bottlefed infants younger than 1 year of age. The reservoir is infant cases and adult carriers with transmission by the fecal–oral route. Nursery outbreaks demonstrate the importance of spread by fomites, which suggests that the infecting dose for infants is low. Adult cases are felt to require a very high infecting dose (108 to 1010 bacteria).
Nursery outbreaks and endemic diarrheas occur in developing world
Pathogenesis
Enteropathogenic E coli initially attach to small intestine enterocytes using bundle-forming (Bfp) pili to form clustered microcolonies on the enterocyte cell surface. The lesion then progresses with localized degeneration brush border, loss of the microvilli, and changes in the cell morphology including the production of dramatic “pedestals” with the EPEC bacterium at their apex. The combination of these actions is called the attachment and effacing (A/E) lesion (Figure 33–5). The many steps involved in the formation of the A/E lesion are genetically controlled in a PAI, which includes the genes for the major EPEC attachment protein, intimin, and an injection (type III) secretion system. The secretion system injects over 30 E coli secretion proteins (Esps) into the host cell cytoplasm including—remarkably—the surface receptor (Tir) for intimin which migrates to the surface after its injection. The other E coli secretion proteins perturb intracellular signal transduction pathways, one effect of which is the induction of modifications in enterocyte cytoskeleton proteins (actin, talin). The cytoskeleton accumulates beneath the attached bacteria to form the pedestals and complete the actin-rich A/E lesion (Figure 33–6). The Esps cause a host of other intracellular disruptions, including mitochondrial injury and induction of apoptosis. The link between these morphologic changes of the A/E and diarrhea is not known, but the injected Esps have also been shown to change electrolyte transport across the luminal membrane.
FIGURE 33–5. Enteropathogenic Escherichia coli (EPEC) attachment to epithelial cells. The EPEC are attaching to and effacing the microvilli on the epithelial cell surface. The cell’s filamentous actin is rearranged at the attachment point. Note the pedestal below the EPEC cell.
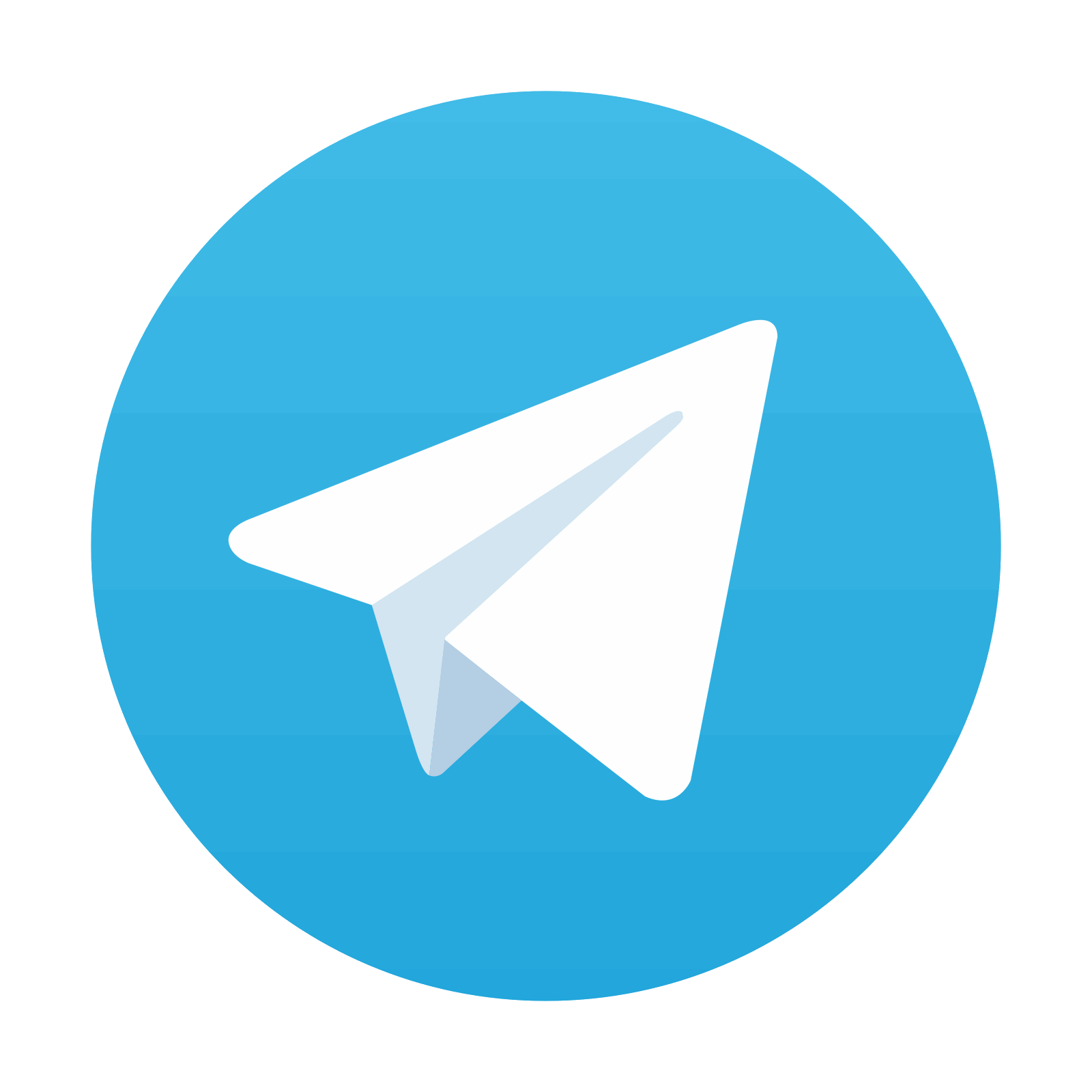
Stay updated, free articles. Join our Telegram channel

Full access? Get Clinical Tree
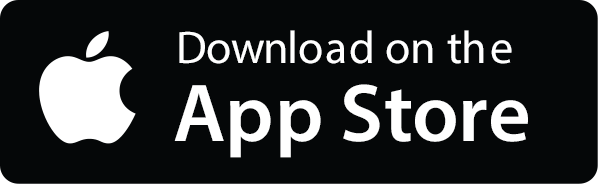
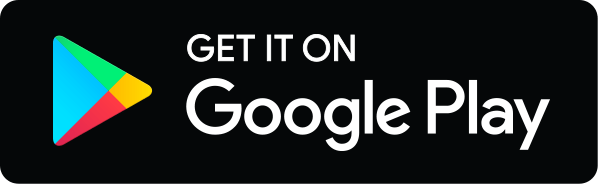