High-Yield Terms
Triglyceride: also called triacylglyceride or triacylglycerol, major storage form of lipid in the body, composed of a glycerol backbone and 3 esterified fatty acids
Phospholipid: major lipid component of all cell membranes, composed of a glycerol backbone esterified to 2 fatty acids with phosphate esterified to the sn3 position, the phosphate group, with an esterified alcohol, is referred to as the polar head group of phospholipids
Lipin: a family of enzymes exhibiting both phosphatidic acid phosphatase activity involved in triglyceride synthesis and transcriptional coactivator activity regulating expression of genes involved in lipid metabolism and adipocyte differentiation
Plasmalogens: are glycerol ether phospholipids mainly of 2 types: alkyl ether (–O–CH2–) and alkenyl ether (–O–CH=CH–); the plasmalogen platelet-activating factor (PAF) is one of the most potent biological lipids
Synthesis of Triglycerides
Triglycerides (TGs) constitute molecules of glycerol to which 3 fatty acids have been esterified. The fatty acids present in TGs are predominantly saturated. The major building block for the synthesis of TGs, in tissues other than adipose tissue, is glycerol. Adipocytes lack glycerol kinase; therefore, dihydroxyacetone phosphate (DHAP), produced during glycolysis, is the precursor for TG synthesis in adipose tissue. This means that adipocytes must have glucose to oxidize in order to store fatty acids in the form of TG. DHAP can also serve as a backbone precursor for TG synthesis in tissues other than adipose tissue, but does so to a much lesser extent than glycerol.
The glycerol backbone of TG is activated by phosphorylation at the sn3 position by glycerol kinase. The utilization of DHAP for the backbone is carried out through either of 2 pathways depending on whether the synthesis of TG is carried out in the mitochondria and ER or the ER and the peroxisomes. In the first situation, the action of glycerol-3-phosphate dehydrogenase (the same reaction as that used in the glycerol-phosphate shuttle) reduces DHAP to glycerol 3-phosphate. Glycerol-3-phosphate acyltransferase (GPAT) then esterifies a fatty acid to glycerol 3-phosphate, generating the monoacylglycerol phosphate structure called lysophosphatidic acid. The expression of the GPAT gene is under the influence of the transcription factor ChREBP (see Chapter 19).
The second reaction pathway utilizes the peroxisomal enzyme DHAP acyltransferase to fatty acylated DHAP to acyl-DHAP, which is then reduced by the NADPH-requiring enzyme acyl-DHAP reductase. An interesting feature of the latter pathway is that DHAP acyltransferase is one of only a few enzymes that are targeted to the peroxisomes through the recognition of a peroxisome-targeting sequence 2 (PTS2) motif in the enzyme. Most peroxisomal enzymes contain a PTS1 motif.
The fatty acids incorporated into TGs are activated to acyl-CoAs through the action of acyl-CoA synthetases. Two molecules of acyl-CoA are esterified to glycerol-3-phosphate to yield 1,2-diacylglycerol phosphate (commonly identified as phosphatidic acid). The phosphate is then removed, by phosphatidic acid phosphatase (PAP1), to yield 1,2-diacylglycerol, the substrate for addition of the third fatty acid. Intestinal monoacylglycerols, derived from the hydrolysis of dietary fats, can also serve as substrates for the synthesis of 1,2-diacylglycerols (Figure 20-1).
FIGURE 20-1: Biosynthesis of triacylglycerol and phospholipids. (, Monoacylglycerol pathway;
, glycerol phosphate pathway.) Phosphatidylethanolamine may be formed from ethanolamine by a pathway similar to that shown for the formation of phosphatidylcholine from choline. Murray RK, Bender DA, Botham KM, Kennelly PJ, Rodwell VW, Weil PA. Harper’s Illustrated Biochemistry, 29th ed. New York, NY: McGraw-Hill; 2012.
Lipin Genes: Triglyceride Synthesis and Transcriptional Regulation
Recent studies have identified a critical role for the enzyme PAP1 in overall TG and phospholipid homeostasis. The lipin-1 gene (LPIN1) was originally identified in a mutant mouse called the fatty liver dystrophy (fld) mouse. The mutation causing this disorder was found to reside in the LPIN1 gene. There are 3 lipin genes (LPIN1, LPIN2, and LPIN3) with the LPIN1 gene encoding 3 isoforms derived through alternative splicing. These 3 lipin-1 isoforms are identified as lipin-1α, lipin-1β, and lipin-1γ. All 5 lipin proteins possess phosphatidic acid phosphatase activity that is dependent on Mg2+ or Mn2+ and phosphatidic acid as the substrate.
Lipin-1 interacts with the transcription factors PGC-1α (peroxisome proliferator-activated receptor-γ [PPARγ] coactivator 1α) and PPARα, leading to enhanced gene expression. Lipin-1 also induces the expression of the adipogenic transcription factors PPARγ and CCAAT-enhancer–binding protein α (C/EBPα). Lipin-1α induces genes that promote adipocyte differentiation while lipin-1β induces the expression of lipid-synthesizing genes such as fatty acid synthase (FAS) and diacylglycerol acyltransferase (DGAT). The interactions of lipin-1 with PPARα and PGC-1α lead to increased expression of fatty acid–oxidizing genes such as carnitine palmitoyltransferase-1, acyl-CoA oxidase, and medium-chain acyl-CoA dehydrogenase (MCAD).
Phospholipid Synthesis
Phospholipids can be synthesized by 2 mechanisms. One utilizes a CDP-activated polar head group for attachment to the phosphate of phosphatidic acid. The other utilizes CDP-activated 1,2-diacylglycerol and an inactivated polar head group (see Figure 20-1). Phospholipids are synthesized by esterification of an alcohol to the phosphate of phosphatidic acid (1,2-diacylglycerol phosphate). Most phospholipids have a saturated fatty acid on C–1 and an unsaturated fatty acid on C–2 of the glycerol backbone. The most commonly added alcohols (serine, ethanolamine, and choline) also contain nitrogen that may be positively charged, whereas glycerol and inositol do not. There are 6 major classifications of phospholipid.
1. Phosphatidylcholine, PC: This class of phospholipids is also called the lecithins. At physiological pH, phosphatidylcholines are neutral zwitterions. They contain primarily palmitic or stearic acid at carbon-1 and primarily oleic, linoleic, or linolenic acid at carbon-2. The lecithin dipalmitoyl lecithin is a component of lung or pulmonary surfactant. It contains palmitate at both carbon-1 and -2 of glycerol and is the major (80%) phospholipid found in the extracellular lipid layer lining the pulmonary alveoli. Choline is activated first by phosphorylation and then by coupling to CDP prior to attachment to phosphatidic acid. PC is also synthesized by the addition of choline to CDP-activated 1,2-diacylglycerol. A third pathway to PC synthesis involves the conversion of either PS or PE to PC. The conversion of PS to PC first requires decarboxylation of PS to yield PE; this then undergoes a series of 3 methylation reactions utilizing S-adenosylmethionine (SAM) as methyl group donor.
In addition to the obvious role of lipin-1 in TG synthesis, evidence indicates that the protein is also required for the development of mature adipocytes, coordination of peripheral tissue glucose, and fatty acid storage and utilization, and serves as a transcriptional coactivator. The latter function has significance to diabetes as it has been shown that some of the effects of the thiazolidinedione (TZD) class of drugs used to treat the hyperglycemia associated with Type 2 diabetes are exerted via the effects of lipin-1.
2. Phosphatidylethanolamine, PE: These molecules are neutral zwitterions at physiological pH. They contain primarily palmitic or stearic acid on carbon-1 and a long-chain unsaturated fatty acid (eg, 18:2, 20:4, and 22:6) on carbon-2. Synthesis of PE can occur by 2 pathways. The first requires that ethanolamine be activated by phosphorylation and then by coupling to CDP. The ethanolamine is then transferred from CDP-ethanolamine to phosphatidic acid to yield PE. The second involves the decarboxylation of PS.
3. Phosphatidylserine PS: Phosphatidylserines will carry a net charge of −1 at physiological pH and are composed of fatty acids similar to the phosphatidylethanolamine. The pathway for PS synthesis involves an exchange reaction of serine for ethanolamine in PE. This exchange occurs when PE is in the lipid bilayer of a membrane. PS can serve as a source of PE through a decarboxylation reaction.
4. Phosphatidylinositol, PI: These molecules contain almost exclusively stearic acid at carbon-1 and arachidonic acid at carbon-2. Phosphatidylinositols composed exclusively of nonphosphorylated inositol exhibit a net charge of −1 at physiological pH. These molecules exist in membranes with various levels of phosphate esterified to the hydroxyls of the inositol. Molecules with phosphorylated inositol are termed polyphosphoinositides. The polyphosphoinositides are important intracellular transducers of signals emanating from the plasma membrane. The synthesis of PI involves CDP-activated 1,2-diacylglycerol condensation with myo-inositol. PI subsequently undergoes a series of phosphorylations of the hydroxyls of inositol, leading to the production of polyphosphoinositides. One polyphosphoinositide (phosphatidylinositol 4,5-bisphosphate, PIP2) is a critically important membrane phospholipid involved in the transmission of signals for cell growth and differentiation from outside the cell to inside.
5. Phosphatidylglycerol, PG: Phosphatidylglycerols exhibit a net charge of −1 at physiological pH. These molecules are found in high concentration in mitochondrial membranes and as components of pulmonary surfactant. Phosphatidylglycerol also is a precursor for the synthesis of cardiolipin. PG is synthesized from CDP-diacylglycerol and glycerol 3-phosphate. The vital role of PG is to serve as the precursor for the synthesis of diphosphatidylglycerols (DPGs).
6. Cardiolipins (diphosphatidylglycerols, DPG): These molecules are very acidic, exhibiting a net charge of −2 at physiological pH (Figure 20-2). They are found primarily in the inner mitochondrial membrane and also as components of pulmonary surfactant.
FIGURE 20-2: Biosynthesis of cardiolipin. Murray RK, Bender DA, Botham KM, Kennelly PJ, Rodwell VW, Weil PA. Harper’s Illustrated Biochemistry, 29th ed. New York, NY: McGraw-Hill; 2012.
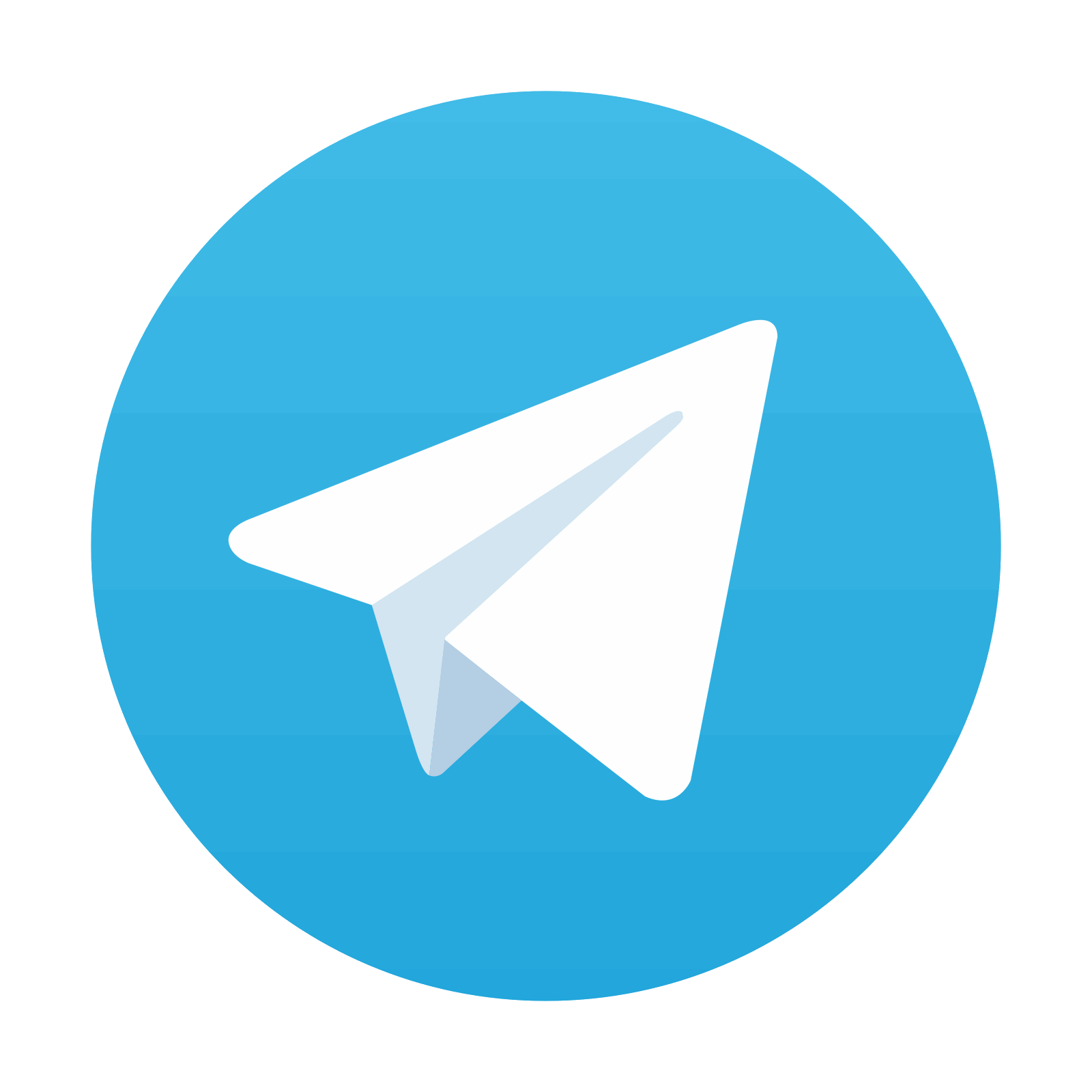
Stay updated, free articles. Join our Telegram channel

Full access? Get Clinical Tree
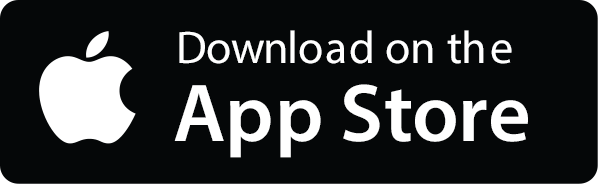
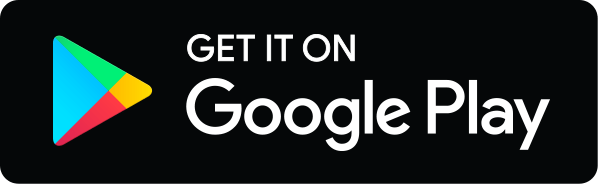