A. DIFFERENT TYPES OF MATERIALS
Materials science is the science of solids, a field that encompasses most aspects of modern life. This book provides an introductory, qualitative overview of the role of chemistry in several important and expanding areas of materials science, with an emphasis on the ways in which new materials are designed, synthesized, evaluated, and used. It starts from the recognition that there are six or seven different types of solid substances that are the basis of materials science. These are polymers, ceramics, metals, element and interelement semiconductors, superconductors, optical materials, and a range of species in which small molecules are packed into ordered solids (Figure 1.1). These fields were once separate disciplines with little or no exchange of ideas across the boundaries. This is no longer true, and the central area in Figure 1.1 symbolizes research that joins and crosses the different disciplines.
For example, many of the ideas that were developed originally for polymer chemistry are found to explain some of the more puzzling aspects of ceramic science, and vice versa. Concepts that were once thought to be specific to inorganic semiconductors may explain the behavior of some polymers and small molecules in solids. Synthetic diamonds, especially diamond coatings produced by chemical vapor deposition, have characteristics that are reminiscent of both ceramics and semiconductors. Zeolites, which are inorganic materials that contain cavities that accommodate guest molecules and catalyze their reactions, have ceramic network structures but behave like the clathrates formed from small organic molecules in ordered solids. Some ceramics are superconductors, a phenomenon hitherto known only for certain metals and alloys and one inorganic polymer. Thus, a number of the most interesting advanced developments in theory, design, and synthesis are taking place in the central area of hybrid materials shown in Figure 1.1.
Figure 1.1. Different types of materials. Ceramics, metals, semiconductors, and optical materials are traditionally derived from inorganic sources. Polymers and small molecules in solids are normally obtained from organic or organometallic starting materials. The central area represents new types of materials that combine ideas and structures from the traditional areas in order to generate new combinations of properties. It is in this central area that some of the most important future advances can be expected.

This is particularly important because each of the classical materials have advantages and disadvantages. These are summarized in Figure 1.2. For instance, classical ceramics are rigid, chemically inert, and withstand high temperatures, but they are heavy, often brittle, and are difficult to fabricate into complex shapes. Most common metals are strong, tough, and good electrical conductors, but nearly all are heavy and prone to corrosion. There are also serious environmental penalties to be paid for their extraction from minerals and their refining. Inorganic semiconductors play a vital role in communications technology and computing but are difficult to purify and fabricate and are thus expensive. Classical polymers are inexpensive because they are derived from plentiful petroleum. They are easily fabricated because of their low softening temperatures, corrosion resistance, and excellent electrical insulating property. However, most polymers melt at only moderate temperatures, decompose when heated in air, and are flammable. Materials derived from small molecules packed into solids may be semiconductors, but they are brittle and melt at relatively low temperatures. These advantages and disadvantages further illustrate the importance of the central area in Figure 1.1, where cross-disciplinary research aims to produce materials that retain the advantages but minimize the disadvantages of existing materials.
All but two of the main materials areas were once based exclusively on inorganic chemistry. The exceptions were polymer science and small molecules in solids, both of which leaned heavily for their traditions on organic and organometallic chemistry. However, here, too, the boundaries are disappearing, as easily fabricated organic polymeric semiconductors replace traditional silicon semiconductors for some applications, transparent organic glasses replace the heavier silicate-based glasses in lenses, prisms, and some optical waveguides and switches, and ultrastrong polymer–ceramic composites have grown in importance as replacements for metals. Polymers that are part organic and part inorganic have properties that cannot be duplicated by classical polymers, metals, or inorganic ceramics, and these hold considerable promise for the future.
Finally, it is essential for the reader to recognize the fundamental principle that materials science extends across all areas of chemistry, physics, engineering, biology, and medicine, and that breadth of knowledge across the physical and biological sciences is an essential requirement for understanding the materials field.
The uses of materials are extremely diverse, and a few of them are summarized in Table 1.1 and Figure 1.3. Some fairly obvious applications are as structural materials in automobiles, aircraft, ships, trains, buildings, computer housings, nuclear reactors, and other structures (polymers, metals, and ceramics) where strength, impact resistance, and heat or radiation stability are important.
Other uses are in medical and dental applications that range from instruments, cardiovascular components (Figure 1.3a), drug delivery materials, scaffolds for tissue regeneration, and membranes (metals, ceramics, polymers). Still other applications for materials are in the electrical, computing, and communications sectors [semiconductors, electroluminescent screens, plasma displays, lasers, wiring, insulation. optoelectronic switches, and satellites (Figure 1.3b)]. Finally, a wide range of fibers, films, coatings, membranes, and elastomers are essential components of clothing, automobiles, aircraft, furniture, and manufacturing machinery. Many of these applications cannot be improved unless new materials are discovered that, for example, are fire-resistant, are unaffected by solvents or aggressive chemicals, or remain flexible at very low temperatures.
TABLE 1.1. Example Uses of Advanced Materials in Devices and Machines

Figure 1.3. (a) A prosthetic heart valve with a stainless-steel cage, a silicone rubber ball valve, and a polyester collar for suturing into the damaged organ. (b) The International Space Station showing the silicon semiconductor solar panels and the metal main structure (courtesy of NASA).

Most technology is materials limited. In other words, most advances in technology that are deemed to be desirable or even essential are blocked by the lack of materials that have the right combination of properties. For example, a certain ceramic may be stable to 2000°C and would otherwise be used in gas turbines, but it is too brittle to withstand the shock waves that emanate from the jet engine. A promising polymer has the correct elasticity to be used in replacement heart valves, but its surface characteristics trigger a cascade of reactions that lead to the formation of a blood clot. A superconductor designed for use in levitated trains works well at –150°C but loses its superconducting properties when warmed to room temperature.
C. APPROACHES TO PRODUCING NEW MATERIALS, NEW PROPERTIES, AND USES
There are two different approaches to research and development in materials science. The first is a traditional method used by engineers and many biomedical materials scientists. In this approach, materials are chosen from long-existing solids with a well-known, fixed set of properties that can be changed somewhat by physical manipulation (heat annealing, stretching, incorporation into composites, etc.). Typically this manipulation changes the way in which the molecules or crystallites are organized in the solid state without altering the overall molecular structure. The overwhelming credo is to improve devices or machines by new engineering designs using well-known inexpensive materials rather than more costly new materials. New materials often mean a lot of trouble because engineering and medical protocols have been fixed by long and arduous trial-and-error procedures. Nevertheless, this approach usually must be discarded for critical advanced applications such as aerospace developments or advanced medical devices, where no amount of manipulation of existing materials can generate the crucial combination of required properties.
The second approach is to use chemistry to produce entirely new materials. Chemists are uniquely placed to design and synthesize new materials. Chemists visualize materials down to their structure at the molecular level. They seek to improve materials through molecular chemistry. They ask two related questions: (1) “What is it about the molecular structure of a material that is responsible for its properties?” and (2) “How can I change the molecular structure to improve the materials properties?” This approach is more likely to generate dramatic advances in properties and performance. However, the molecular chemistry approach is successful only if it leads to a marked and often dramatic improvement in properties, because the cost of new products is always high until they can be produced and used on a large scale.
In practice, both the materials manipulation and the molecular chemistry approaches are important, and the challenge is to bring the two together. This connection of chemistry to engineering and medicine is one of the key features of materials science and biomaterials research. It becomes manifest in the sequence of events that occur along the pathway to the discovery and development of new materials. This is illustrated in Figure 1.4. The sequence starts with synthetic and mechanistic discoveries in small-molecule chemistry of the type that all chemists are familiar with. These discoveries can be in organic, inorganic, or organometallic chemistry. In addition, a few of these advances may provide access to new polymers, ceramics, semiconductors, and other materials with entirely new combinations of properties by processes that are described in later chapters. The characterization of these new substances by physical and analytical techniques will provide clues to their possible utility.
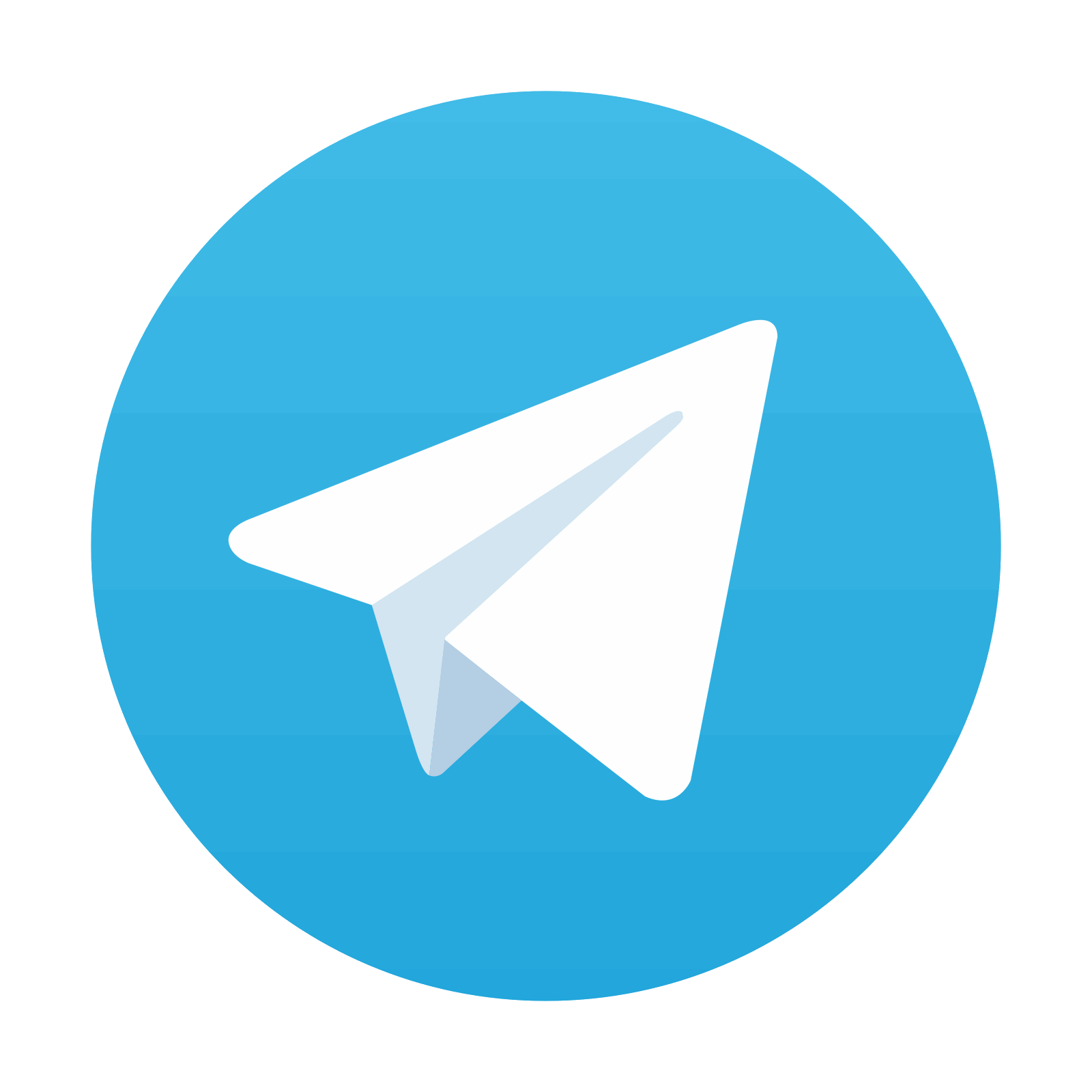
Stay updated, free articles. Join our Telegram channel

Full access? Get Clinical Tree
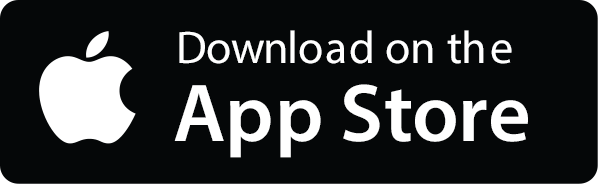
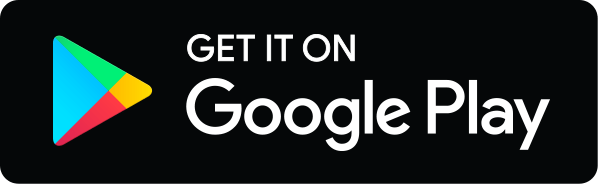