© Springer International Publishing Switzerland 2016
Ivan D. Montoya (ed.)Biologics to Treat Substance Use Disorders10.1007/978-3-319-23150-1_33. Vaccines for Treating Cocaine Use Disorders
(1)
Michael E DeBakey Veterans Affairs Medical Center, Houston, TX, USA
(2)
Menninger Department of Psychiatry and Behavioral Sciences, Baylor College of Medicine, Houston, TX, USA
Keywords
Substance use disordersImmunotherapiesVaccineCocaineTA-CDDr Kosten is the JH Waggoner Chair and Professor of Psychiatry, Pharmacology, Neuroscience, Immunology, and Pathology at Baylor College of Medicine.
3.1 Introduction
Worldwide, the United Nations Office on Drugs and Crime (UNODC) estimates the global annual prevalence of cocaine use to be between .3 and .4 %, affecting 13–20 million individuals between 15 and 64 years of age. North America remains among the top 3 markets for cocaine with more than 5 million past year users and an estimated 3000 deaths annually due to cocaine use (United Nations Office on Drugs and Crime (UNODC) 2012). The impact of cocaine use disorders on our healthcare system is significant, making it a major public health issue. A recent US National Survey on Drug Use and Health noted that cocaine use disorders accounted for 8 % of all patients who entered substance abuse treatment programs (SAMHSA 2012a, b). Further, of patients 21 years or older, cocaine was found to be the most common illicit drug involved in drug-related emergency department (ED) visits, accounting for almost half of the approximately one million illicit drug-related ED visits annually (NIH-NIDA 2011). These drug-related ED visits cover a wide range of brain and cardiovascular-related disorders (Kosten et al. 2011). Brain disorders include seizures, strokes, movement disorders, more general neuropsychiatric impairment, and psychoses. Movement disorders associated with stimulants include chorea, dystonia, akathisia, and choreoathetosis. While cocaine-induced psychosis is uncommon, users can experience delusions of persecution and hallucinations of virtually any type. Cocaine’s profound effects on the cardiovascular system can result in serious and often lethal complications including myocardial ischemia and infarction, cardiomyopathy, myocarditis, arrhythmias, endocarditis, and aortic dissection. Within the first hour after cocaine intake, the risk of myocardial infarction (MI) is significant and is 23.7 times greater compared to 3 h following intake in individuals who are otherwise at low risk for MI (Mittleman et al. 1991). Chest pain is commonly experienced by cocaine users that present to emergency departments and is associated with acute MI in upward of 6 % of these patients (Mukherjee 2008). Obstructive coronary artery disease has been found in 35–40 % in patients that experience cocaine-induced chest pain that underwent diagnostic angiography (Dressler et al. 1990). Thus, developing a medication that would not only reduce the reinforcing properties of cocaine but also its many medical complications is a critical need. Although research is advancing, there are presently no FDA-approved pharmacotherapies for cocaine dependence (Kosten et al. 2011; Haile et al. 2012).
In terms of optimal pharmacotherapeutic interventions for the treatment of cocaine use disorder, characteristics such as agent duration of action and frequency of administration are primary considerations. Immunotherapies present a relevant and timely intervention, since they can be long-acting agents to prevent relapse as well as blocking a broad range of cocaine’s effects, including those in the central nervous system (e.g., reward, reinforcement, neuropsychiatric sequelae) as well as cardiovascular system. Active vaccines against cocaine have a substantial history of development and can potentially reduce reinforcement and some acute toxicity from cocaine (Kosten and Owens 2005; Orson et al. 2008; Haney and Kosten 2004). These vaccine-induced antibodies are meant to bind to cocaine molecules in the circulation, preventing their ability to traverse the blood–brain barrier, and prevent activation of the brain’s reinforcement pathways (e.g., dopamine activation of the nucleus accumbens) and thus blunt the addictive liability of cocaine. An important component of this capture of cocaine by the antibody is that bound cocaine molecules are metabolized into inactive compounds which minimally bind to the antibody and are subsequently excreted (Fox et al. 1996). The cocaine is broken down by pseudocholinesterase in the circulation or by nonenzymatic hydrolysis into inactive metabolites such as benzoylecgonine, ecgonine methylester, and benzoic acid body (Stewart et al. 1979). In theory, these inactive metabolites do not bind to the antibody. Therefore, free antibody is available to bind more cocaine, rather than remaining attached to metabolites. This detachment from inactive metabolites likely prolongs and potentiates the antibodies’ capacity for blocking more cocaine from entering the brain (Kosten et al. 2012).
This simple “sponge” model of anti-cocaine antibodies probably describes a significant component of their actions (Shen and Kosten 2011). However, these antibodies cannot indefinitely soak up the abused drugs since the total number of antibody binding sites is necessarily limited. Furthermore, not every cocaine molecule of a bolus dose can be tightly bound. The additional actions of the anti-cocaine antibodies act as pharmacokinetic buffers against the rapid transit of cocaine into the brain. The rapid brain accumulation within seconds from a bolus cocaine dose delivered by either smoked or intravenous cocaine administration is prevented by the relatively slower equilibrium developed between free and antibody-bound cocaine in the blood. Smoked cocaine administration is highly reinforcing while cocaine powder for intranasal administration has much less reinforcement for an equivalent dose (Nelson et al. 2006; Cornish and O’Brien 1996; Walsh et al. 2009; Smith et al. 2001; Rush et al. 1999). Furthermore, oral cocaine requires considerably larger doses to be as reinforcing as the intravenous route of administration. This need for an increased dose in part reflects that oral cocaine reaches the brain relatively slowly, and although high blood and brain levels can be attained, much higher levels are needed to produce euphoria or other reinforcing effects. The kinetic effects of a pool of antibodies in circulation may act in a similar way by slowing drug entry into the brain and reducing the reinforcing effects of this drug. Thus, the specificity and ability of antibodies to slow cocaine entry from the blood vessels into the brain, heart, and other key organs can be a more effective blocker of reinforcement and perhaps toxicity than a transporter-based antagonist. However, any antibody strategy will be a competitive antagonism that can be overridden by sufficient doses of cocaine. Overall, these immunotherapies are aids to prevent relapse among patients motivated to become and remain abstinent and are less likely to be successful for inducing abstinence among current heavily cocaine-dependent individuals. Vaccines also will be most successful in conjunction with other behavioral and pharmacological therapies (Kosten et al. 2012).
3.2 Development of Cocaine Immunotherapy
The first report of an anti-cocaine vaccine was made by Bagasra in 1992 (Bagasra et al. 1992) and during that same time period other investigators tried to create cocaine catalytic antibodies (Chandrakumar et al. 1993; Landry et al. 1993; Basmadjian et al. 1995; Berkman et al. 1996). The simple anti-cocaine vaccine linked a cocaine derivative such as norcocaine to an antigenic carrier protein, since the cocaine alone was too small to elicit an immune response. The more complex catalytic antibodies attempted to imitate normal esterases in tissue such as butyrylcholinesterase (Lynch et al. 1997) that hydrolyze cocaine at its phenyl ester to give ecgonine methyl ester and benzoic acid. Because these metabolites have little if any pharmacological activity, the cocaine would be rendered inactive. The concept with catalytic antibodies was to create transition state analogs as haptens that would mimic the intermediate and unstable chemical structures that lead to the hydrolysis reaction. Thus, these antibodies would act as enzymes to catalyze the release of the benzoyl group from cocaine. One vaccination approach produced polyclonal antibodies that were reported to have this effect, although the potential superiority to the already existing normal cholinesterase in human blood was not clear (Chandrakumar et al. 1993). Landry (Landry et al. 1993) produced monoclonal antibodies that had the potential for selection of a high-activity enzyme-like antibody, but this never reached clinical testing. Berkman (Berkman et al. 1996) chemically prepared a hapten that induced antibodies when conjugated to keyhole limpet hemocyanin (KLH) and reported this hapten as being chemically stable and long lived rather than spontaneously breaking down from its seemingly unstable transition state conformation. Monoclonal antibodies were developed from this KLH vaccine in mice, but were not subjected to definitive behavioral testing for efficacy in reducing cocaine actions or reinforcement. Furthermore, although catalytic antibodies would allow a relatively small number of antibodies from a vaccine or monoclonal to destroy cocaine molecules and rapidly take on more cocaine, the quantity, affinity, and behavioral efficacy of these antibodies were not addressed.
At about the same time as these catalytic antibodies were being developed, a significant discovery advanced the development of cocaine vaccines for making antibodies against the cocaine itself rather than making antibodies as catalysts for metabolism of cocaine (Matsushita et al. 2001). This discovery involved the linker molecule between the carrier protein and the cocaine hapten. The structure of this linker had typically included a string of three to eight carbons between the attachment to the carrier protein and the cocaine molecule. Another important variation was in the position on the cocaine molecule for attaching this linker chain. Janda reported that having an amide group in the carbon chain of the linker and having that linker attached at the methyl ester position rather than at the tropane nitrogen of cocaine gave better results than other linkage structures and linking positions on the cocaine. Among the other linking positions, a photoactivation method inserted a linker between one of the carbon-carbon bonds of cocaine rather than the methyl ester or the tropane nitrogen of cocaine (Ettinger et al. 1997). However, this method did not allow control over which bond or how many of the carbon-carbon bonds of the cocaine would get these linker attachments. In spite of this limitation for commercial application of such a vaccine, the resulting vaccine appeared to produce functional antibodies based on both place preference conditioning assays and attenuation of the discriminative stimulus properties of cocaine (Johnson and Ettinger 2000).
Janda’s anti-cocaine vaccine used a hapten named GNC that had a six-carbon linker terminating in a carboxylic acid group and conjugated to KLH on its free lysines (Carrera et al. 1995). Vaccinated rats showed suppression of cocaine-induced locomotor activity and lower brain levels of cocaine. Using this same vaccine, he then showed prevention of cocaine reinstatement of self-administration in rats (Carrera et al. 2000). Jhanda and colleagues then developed a second hapten called GND that replaced the two ester groups of GNC with two amide groups. Similar to GNC, vaccinated rats displayed suppression of cocaine’s locomotor activity (Carrera et al. 2001).
A similar anti-cocaine vaccine was created consisting of succinyl norcocaine (SNC) conjugated to bovine serum albumin (BSA) (Fox et al. 1996). This vaccine reduced cocaine brain levels of vaccinated mice after a cocaine dose. Furthermore, rhesus monkeys vaccinated with SNC-BSA had suppressed operant responding to a food reward (Koetzner et al. 2001). A commercial version of this same vaccine conjugated SNC to cholera toxin B. This commercial vaccine called TA-CD elicited antibody levels sufficient to antagonize self-administration of cocaine in rats (Kantak et al. 2000, 2001). Cocaine-specific antibodies generated in response to TA-CD also reduced early cocaine distribution to the brain by 25–80 % compared to controls. Both TA-CD and the KLH-related vaccines were used subcutaneously in mice. However, one study vaccinated mice both intranasally and subcutaneously with SNC–KLH and measured serum and brain cocaine levels after a challenge with cocaine (Hrafnkelsdottir et al. 2005). The brain levels were less for all cocaine-immunized groups than for controls, but brain levels of cocaine were 2 times higher in the intranasally vaccinated mice than in the subcutaneously vaccinated mice. Because the serum level of antibodies was fivefold higher in the intranasally than in the subcutaneously vaccinated mice, the investigators suggested that mucosal antibodies could sequester the cocaine and that intranasal vaccination could be useful for smoked or snorted cocaine. This intranasal route of administration was never moved to clinical trials, however, and intramuscularly administered TA-CD, which conjugated SNC to inactivated cholera toxin B, is the only cocaine vaccine so far that has made it into clinical trials.
3.3 Clinical Studies of the Cocaine Vaccine TA-CD
Human dosing of the TA-CD cocaine vaccine initially tested three different ascending doses with a dosing schedule based on the rodent work. In the rodents, a dosing schedule of an initial vaccination followed by two additional boosts had produced cocaine-specific antibodies at relatively high titers (Kantak et al. 2000, 2001). The schedule of human dosing therefore was three times over a 12-week period, although one rather than two booster vaccinations are typically given in humans for cholera protection (Svennerholm et al. 1984). The dose of TA-CD in the first human cohort started at 10 ug, because this dose was the standard used for cholera vaccination and was therefore considered relatively safe (Jertborn et al. 1992). Subsequent cohorts of subjects had dosing escalated 10- and 100-fold to 100 ug and 1000 ug per dose.
The phase I trial of TA-CD enrolled formerly cocaine-dependent subjects who were residing in a drug-free residential program without access to cocaine (Kosten et al. 2002). The 34 subjects were allocated to placebo (n = 6), 10 ug (n = 8), 100 ug (n = 10), and 1000 ug (n = 10). The TA-CD-induced cocaine-specific antibodies in all vaccinated subjects, but the peak antibody levels were quite variable across subjects within each of the three dose groups. The average of these peak levels also showed no difference between the 10 ug and 100 ug doses. However, the 1000 ug dose showed a clear doubling in antibody levels compared to these other two doses. These antibody levels declined by fourfold within 3 months after the final vaccination and did not persist beyond 1 year. Overall, the safety profile for the vaccine was quite favorable. Almost all recipients (33 of 34) reported local pain and/or tenderness at the injection site, with no difference between experimental and placebo groups or across the three different vaccine doses. This side effect profile was consistent with what might be expected from the alum adjuvant (Grabenstein 1994). Treatment-related systemic adverse effects that occurred in all groups included mild tachycardia, elevated temperature, and hypertension (Kosten et al. 2002). However, no serious adverse effects occurred during vaccination or the 12 months of follow-up.
Further studies examined the effects of smoked cocaine administration in vaccinated humans (Haney et al. 2010). Ten cocaine-dependent men were assessed for 13 weeks for the effects of cocaine (0, 25, 50 mg) prior to vaccination and at weekly intervals thereafter. Two doses of TA-CD (82 μg, n = 4; 360 μg, n = 6) were administered at weeks 1, 3, 5, and 9. The peak antibody titers ranged from 200 to 2300 units with a mean of 800 units. This mean antibody titer of 800 units corresponded to a 20 μg/ml level of anti-cocaine IgG and based on this study was considered the minimal level needed in order for the TA-CD vaccine to substantially decrease the intoxicating effects of a single smoked cocaine dose. A split of subjects on the mean peak plasma antibody levels showed that those in the upper half had an immediate and robust (55–81 %) reduction in subjective ratings of cocaine effects. However, those in the lower half showed no significant reduction. Higher plasma antibody levels were associated with higher peak heart rate after smoking cocaine at 50 mg, but no other potential toxicities were reported including no allergic or inflammatory responses to the vaccinations. Since cocaine primarily increases heart rate by peripheral rather than central activation of the sympathetic nervous system, higher plasma cocaine levels in the peripheral circulation probably accounted for the enhanced heart rate in the high-antibody group. Plasma cocaine levels significantly correlated with heart rate after being fully vaccinated and taking cocaine. Thus, increased plasma cocaine probably contributed to the greater cocaine-induced tachycardia in the subjects with high antibody levels.
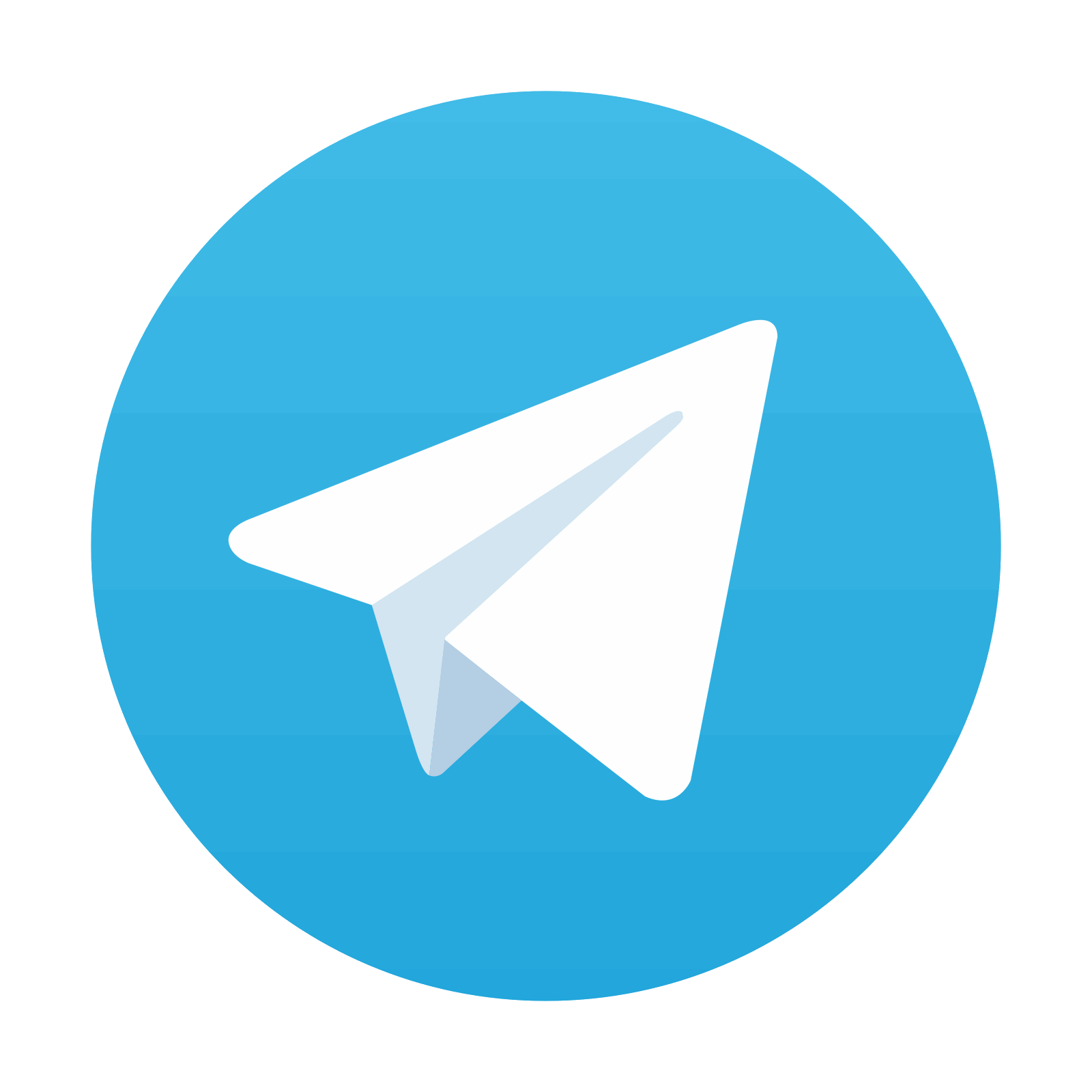
Stay updated, free articles. Join our Telegram channel

Full access? Get Clinical Tree
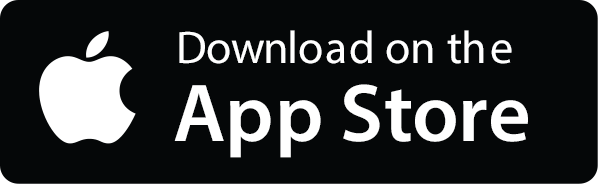
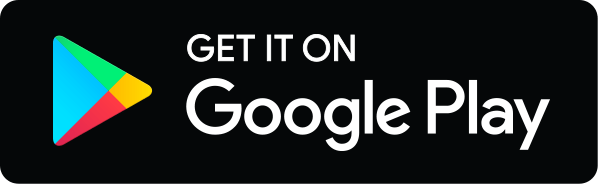