Uropathogenic Escherichia coli
Rachel R. Spurbeck and Harry L.T. Mobley, University of Michigan Medical School, Ann Arbor, MI, USA
Background
Classification and evolution of uropathogenic E. coli
Uropathogenic Escherichia coli (UPEC) are a heterogeneous group of strains within the broader classification of extraintestinal pathogenic E. coli. There is no core set of virulence factors shared by all UPEC. Most UPEC belong to B2 and D phylogroups (Chapter 1) and can be classified based on the O (lipopolysaccharide) serotype into uropathogenic clones, with 58% of UPEC isolates belonging to one of eight serogroups (O1, O2, O4, O6, O8, O9, O18, and O83) (Blanco et al., 1994, 1996). UPEC can be classified by the type or severity of infection from which the bacteria are isolated. Pyelonephritis or urosepsis isolates cause the most severe UTI, infecting the kidneys and allowing access to the bloodstream. Cystitis isolates cause bladder infections, and asymptomatic bacteriuria (ABU) strains stably colonize the urinary tract without causing disease symptoms in an almost commensal state. Comparative genomic hybridization comparing the genomic content of ten UPEC strains and four fecal/commensal strains revealed 10 pathogen-specific genomic islands comprising 13% of the genome of pyelonephritis isolate E. coli CFT073 (Lloyd et al., 2007). Three islands were known pathogenicity islands containing P fimbriae and other characterized virulence factors. Furthermore, 52% of the genome is shared among UPEC and commensal isolates, and only 131 genes of the 5379 genes present in E. coli CFT073 were UPEC-specific (Lloyd et al., 2007).
ABU isolates, like UPEC isolates that cause symptomatic disease, have expanded genomes when compared to fecal commensal E. coli. However, a study of 112 ABU isolates, in which bacteriuria lasted from 1–74 days with at least one urine culture of ≥105 CFU/ml, suggests that ABU isolates have undergone reductive evolution within human hosts. Point mutations and deletions in genes encoding virulence factors reduce the virulence of the ABU isolates over time (Salvador et al., 2012). Therefore, UPEC acquired virulence through horizontal gene transfer events, such as acquisition of PAIs, but reductive evolution attenuates ABU strains into a more commensal-like state.
Epidemiology and global impact
Urinary tract infections (UTIs) are the second most common infection of humans, causing 8.1 million physician visits in the United States in 2007 (Schappert and Rechtsteiner, 2008), and an estimated 10 million cases in western Europe. UTIs are usually caused by bacteria from the intestinal tract contaminating the periurethral opening, followed by ascension of the urinary tract. Women are more susceptible to UTI due to the relative proximity of the periurethral space to the anus and to the fact that the urethra is much shorter than that of men, thus allowing easier access to the bladder by fecal bacteria. It is estimated that over 50% of all women will have a UTI in their lifetime, 25% will then experience a second UTI, and 3% will have a third UTI within 6 months of the initial infection (Foxman, 2003). E. coli is the primary cause of UTIs, responsible for an estimated 80% of all uncomplicated UTIs (Stamm and Hooton, 1993; Ronald, 2002)and 18–35% of long-term indwelling catheter-associated UTIs (Nicolle, 2005).
Molecular pathogenesis
Entry and ascension of the urinary tract
UTIs caused by E. coli are ascending infections in which bacterial contamination of the periurethral space allows UPEC access to the otherwise sterile urinary tract. Ascension of the urinary tract by UPEC is mediated by the action of flagella, which propel bacteria up the urethra to the bladder, where the bacteria utilize a multitude of fimbriae and non-fimbrial adhesins to colonize, iron acquisition systems to facilitate growth, and toxins to avoid the innate immune response (Table 9.1 and Figure 9.1). A subpopulation of E. coli can detach from the bladder and move up the ureters to the kidneys (mediated by flagella) (Lane et al., 2007a; Walters et al., 2012). Flagellar motility is essential for uropathogenesis, as non-motile mutants of UPEC that cannot express FliC, the main component of the flagellum, are attenuated in the mouse model of ascending UTI (Wright et al., 2005; Lane et al., 2007a). E. coli reciprocally regulates fimbrial and flagellar biosynthesis so that when fimbriae are expressed, flagella are down-regulated causing a shift between motile and sessile lifestyles (Lane et al., 2007b). Several transcription factors, including H-NS (Korea et al., 2010), Lrp (Simms and Mobley, 2008a), cAMP-CRP (Yokota and Gots, 1970; Muller et al., 2009), and LeuX (Ritter et al., 1995), as well as non-specific regulators, such as DNA topology (Dorman and Corcoran, 2009), can affect the switch between motility and sessility. Most of these transcription factors regulate the expression of the recombinases FimB and FimE, which affect the orientation of the promoter of the type 1 fimbrial operon as described below (Ritter et al., 1995; Simms and Mobley, 2008b; Dorman and Corcoran, 2009; Muller et al., 2009). Simultaneously, the same regulators affect the transcription of flhDC, which encode the master regulator of flagellar biosynthesis, in an opposite manner, so that when flagella are expressed, fimbrial biosynthesis is repressed.
TABLE 9.1
1Gene or expression more frequent in UPEC than control strains.
2Mutant in gene less able to cause UTI in animal model.
3Ability to cause UTI restored to mutant by re-introduction of gene.
4Evidence of role in UTI from experiments in humans.
5Attenuation and complementation confirmed in murine model of chronic pyelonephritis.
6Attenuated mutant also was hemolysin and serum resistance negative.
Fimbrial operons can encode regulatory elements themselves that affect motility and flagellar expression. For example, a regulatory gene associated with the SfaII fimbrial operon, sfaXII, when overproduced, reduces the amount of FliC, corresponding with lower motility. This same protein also negatively affects type 1 fimbrial expression (Sjostrom et al., 2009). PapX, a protein encoded by the P fimbrial operon that has 96% amino acid sequence identity to SfaX, also inhibits flagellar gene expression in UPEC (Simms and Mobley, 2008b). Although the mechanisms are unknown, the expression of type 1 and Yad fimbriae also inhibit flagellar expression (Lane et al., 2007b; Simms and Mobley, 2008a; Spurbeck et al., 2011), demonstrating that production of fimbriae can have indirect effects on motility.
Adherence
Colonization of the urinary tract is an essential step in the infection process. Without binding to the host epithelium, bacteria would be washed out of the urinary tract by the force of urination. UPEC have several different mechanisms for adherence to the uroepithelium, including: fimbriae, rod-like appendages that protrude from the bacterial cell surface culminating in a tip adhesin; fibrillae, flexible, extended conformations with adhesins present throughout the structure (i.e. not only at the tip); and afimbrial adhesins such as autotransporters. Extensive regulatory systems coordinate expression of these different adhesins (Snyder et al., 2005). Study of the regulatory networks coordinating expression of fimbrial adhesins is an active area of research, which could eventually lead to the development of novel therapeutic targets.
Fimbriae
The prototypical pyelonephritis strain E. coli CFT073 encodes 12 fimbrial operons in its genome (Welch et al., 2002) including 10 chaperone-usher fimbriae (Chapter 12) and two putative type IV pili (Chapter 13). Eight of the chaperone-usher fimbriae have been studied for effects on uropathogenesis: type 1, F1C, Auf, Ygi, Yad, F9, and two P fimbriae. The remaining four, Yeh; Yfc; and two type IV pili, remain to be studied. Pix fimbriae have been associated with uropathogenic E. coli and have been studied in the pyelonephitis isolate E. coli 536, however, molecular epidemiologic data demonstrate that this fimbria is rare (Spurbeck et al., 2011). Dr fimbriae are fibrillar structures that have been associated with uropathogenic strains of E. coli as well as diffuse adhering E. coli (DAEC), and will be discussed below.
Type 1 fimbriae
Type 1 fimbriae, encoded in the genomes of most (99%) E. coli strains (Vigil et al., 2011a, b), are necessary for colonization of the oropharynx as a precursor to intestinal colonization (Orndorff and Bloch, 1990). Type 1 fimbriae primarily consist of FimA, the main structural subunit, several minor subunits, and the adhesin, FimH, which is found at the tip of the fimbriae as well as intermittently throughout the shaft (Klemm et al., 1990; Krogfelt et al., 1990). These fimbriae enhance pathogenicity in the urinary tract by mediating adherence to and invasion of bladder epithelial cells by binding mannose moieties (Brinton, 1959) and uroplakin 1a with the tip adhesin FimH (Zhou et al., 2001), as well as by binding to muscle cells and vascular walls (Virkola et al., 1988). Type 1 fimbriae cause damage to the urinary tract by increasing inflammation during infection (Connell et al., 1996). The promoter for type 1 fimbriae resides within an invertible element (designated the fim switch or fimS), and thus type 1 fimbriae are subject to phase variation at the level of transcription. This phase variation is regulated by the activity of two recombinases, FimB and FimE, on the fim switch (see below in the section on the regulation hierarchy of fimbriae). Type 1 fimbriae are a virulence factor of UPEC in experimental models, as shown in the studies by Connell et al. (1996) that demonstrated deletion of fimH reduced colonization and inflammation in the mouse model of ascending UTI. Furthermore, colonization of the murine urinary tract and inflammatogenicity were restored in the fimH mutant by expression of fimH from a plasmid, fulfilling Koch’s postulates (Connell et al., 1996).
P fimbriae
P fimbria was the first UPEC virulence factor characterized (Eden et al., 1976). The pap (pyelonephritis associated pili) operon encoding P fimbriae consists of 11 genes, with the main fimbrial subunit encoded by papA and the tip adhesin encoded by papG (Hull et al., 1981). The adherence mediated by P fimbriae is mannose-resistant, unlike that of type 1 fimbriae, and is specific to P blood group antigen, a glycosphingolipid, in which the key epitope is a digalactoside, α-D-Gal-(1-4)-β-D-Gal (Källenius and Möllby, 1979). There are three alleles of papG, each with substrate specificity to a different region of the Gal-Gal disaccharide-containing glycosphingolipids (Stromberg et al., 1991). P fimbriae are encoded in the genomes of 54–70% of UPEC isolates as compared to 20–25% of fecal E. coli strains, with the papG allelic distribution being papG1 (0–1% UPEC, 0–1% fecal), papG2 (36–46% UPEC, 16–18% fecal), papG3 (17–23% UPEC, 5–9% fecal) (Johanson et al., 1993; Johnson et al., 1998; Spurbeck et al., 2011). P fimbriae enhance the severity of UTI by promoting adherence strongly to vascular endothelium, the muscular layer, and weakly to bladder epithelial surfaces (Virkola et al., 1988), as well as increasing mucosal inflammation. Although there is a positive correlation between severity of infection and presence of P fimbriae (Spurbeck et al., 2011; Vigil et al., 2011b), and antibodies to P fimbriae are present in the serum of infected individuals (de Ree and van den Bosch, 1987), there is no defect in colonization of the mouse model of ascending UTI in the P fimbria isogenic mutant (Mobley et al., 1993). However, in a pyelonephritis model in cynomolgus monkeys wild-type E. coli DS17 colonized the urinary tract longer and caused a loss of kidney function, whereas E. coli DS17-8 (ΔpapG) was cleared earlier and did not cause as extensive kidney damage (Roberts et al., 1994). While P fimbriae are thought to play only a subtle role in uropathogenesis, the presence of the pap operon is clearly a marker of highly virulent strains.
F1C fimbriae
In a recent study, the prevalence of F1C fimbriae was not significantly different between UPEC (16%) and fecal E. coli strains (10%) (Spurbeck et al., 2011), however, other studies have shown that the presence of F1C fimbriae correlates with pyelonephritis isolates (Johnson et al., 2005b). An operon of seven genes, focAICDFGH, encodes F1C fimbria (Riegman et al., 1990), with FocA representing the major subunit of the pilus and FocH the tip adhesin. F1C fimbriae bind receptors present in both the bladder and kidneys (Virkola et al., 1988). F1C fimbriae mediate adhesion to the endothelium and the muscular layer of the bladder, but not to the epithelium (Virkola et al., 1988). In the kidneys, F1C mediates adherence primarily to the distal tubules and collecting ducts, however, these fimbriae also bind in the glomeruli and to the vascular endothelium (Virkola et al., 1988). The receptor structure of F1C fimbriae was determined to be the GalNAcβ1-4Galβ sequence of glycolipids (Khan et al., 2000). Therefore, F1C fimbriae have the potential to be a virulence factor involved in colonization of the urinary tract. However, there has been no experimental infection conducted to demonstrate the importance of F1C fimbriae during a urinary tract infection.
S fimbriae
S fimbriae are encoded by 15% of UPEC isolates as compared to 5% fecal E. coli strains, and are highly associated with cystitis and ABU isolates (Spurbeck et al., 2011). Genetically identical to F1C fimbriae, with exception of the tip adhesin, S fimbriae are often grouped with F1C fimbriae in epidemiological studies (Ott et al., 1988). However, the difference in the tip adhesin between F1C and S fimbriae confers different binding specificities; thus, these similar fimbriae should be considered different virulence factors for UPEC (Marre et al., 1990). SfaS, the tip adhesin, binds sialic acid present on epithelial cells in the human kidney (Morschhauser et al., 1990), and can be inhibited by addition of the receptor analog sialyl(α2-3)lactose (Korhonen et al., 1986). Specifically, S fimbriae bind to vascular endothelium in the large vessels of kidney tissue and the capillary endothelium in the interstitium and glomerulus. S fimbriae also bind to the epithelium in the lumen of the proximal and distal tubules, the collecting ducts, and the glomerular epithelium (Korhonen et al., 1986). In the bladder, S fimbriae bind to the epithelial and muscular layers as well as connective tissue (Virkola et al., 1988). In a rat model of pyelonephritis, deletion of a region of the chromosome that encodes hemolysin, S fimbriae, and serum resistance from the pyelonephritis isolate E. coli 536 attenuated the mutant. When the mutant was complemented with a plasmid containing the sfa operon, the number of bacteria recovered from the kidneys of infected rats was increased 20-fold (Marre et al., 1986). Thus, S fimbriae fulfill the criteria of a virulence determinant in UPEC.
F9 fimbriae
F9 fimbriae (encoded by the f9 operon) are encoded by 78% of UPEC isolates as compared to 56% fecal E. coli strains (Spurbeck et al., 2011). Although F9 fimbriae do not agglutinate red blood cells from human, dog, horse, or sheep, nor promote adherence to HeLa epithelial cells, this fimbria mediates biofilm formation in M9 minimal salts medium when expressed in a non-fimbriated strain of E. coli (Ulett et al., 2007a). However, when the f9 operon was deleted from E. coli CFT073, no significant difference in biofilm formation was observed, even when type 1 fimbriae and F1C fimbriae, which have been implicated in biofilm formation, were also deleted from the chromosome (Ulett et al., 2007a). Thus, F9 fimbriae play, at most, a minor role in biofilm formation by UPEC on abiotic surfaces. F9 fimbriae are expressed when E. coli CFT073 is cultured in human urine, which may indicate that these fimbriae have some role in virulence (Spurbeck et al., 2011). However, the role of F9 fimbriae in vivo or on adherence to uroepithelial cells has not been assessed.
Ygi fimbriae
Ygi fimbriae are encoded by 61% of UPEC isolates as compared to 24% fecal E. coli strains, suggesting these fimbriae may be urovirulence factors (Spurbeck et al., 2011). Deletion of the ygi operon from the pyelonephritis strain E. coli CFT073 was sufficient to reduce adherence to the human kidney epithelial cell line, HEK 293, and reduce biofilm formation on abiotic surfaces (Spurbeck et al., 2011). These deficiencies were fully complemented by introduction of the ygi operon on a plasmid. Ygi fimbriae are expressed both in LB medium and in human urine. In the mouse model of ascending UTI, the ygi deletion mutant was outcompeted by wild-type E. coli CFT073 in the kidneys of mice and this phenotype was fully complementable, fulfilling molecular Koch’s postulates, demonstrating that these fimbriae are involved in the pathogenesis of UTI (Spurbeck et al., 2011).
Yad fimbriae
Yad fimbriae are encoded by 46% of UPEC compared to 21% fecal E. coli strains (Spurbeck et al., 2011). Deletion of the yad operon from E. coli CFT073 resulted in diminished adherence to the human bladder epithelial cell line, UM-UC-3, biofilm formation on abiotic surfaces, and motility (Spurbeck et al., 2011). Furthermore, Yad fimbriae are expressed when the bacteria are cultured in human urine, and a double mutant, in which both the yad and ygi fimbrial operons are deleted from the chromosome, was outcompeted by wild-type E. coli CFT073 in bladder and kidneys of the mouse model of ascending UTI. Since the ygi deletion mutant alone is only outcompeted by wild-type E. coli CFT073 in the kidneys, this suggests that Yad fimbriae, along with Ygi fimbriae, are involved in colonization of the bladder (Spurbeck et al., 2011).
Auf fimbriae
Auf fimbriae (another upec fimbriae, encoded by aufABCDEFG) are encoded by 67% of UPEC compared to 27% fecal E. coli strains (Spurbeck et al., 2011). Auf fimbriae, when expressed in E. coli BL21-AI cells, did not agglutinate human, guinea pig, or sheep erythrocytes, nor mediate adherence to T24 bladder epithelial cells or HEp-2 laryngeal carcinoma cells (Buckles et al., 2004). Although the auf operon is transcribed by E. coli CFT073 and 10 other UPEC strains, no protein was detected by immunoblot in vitro. After mice were infected with either wild-type E. coli CFT073 or an aufC mutant, the mice infected with the wild-type strain elicited an immune response to AufA antigen as detected by ELISA, whereas only 1 out of 10 mice infected with the mutant strain had an immune response. This suggested that Auf fimbriae are synthesized in vivo (Buckles et al., 2004). However, results from the mouse model of ascending UTI demonstrate that Auf fimbriae do not play a significant role in colonization, as there was no significant difference in colonization between wild-type E. coli CFT073 and the auf mutant and the mutant was not outcompeted by wild-type in competition studies (Buckles et al., 2004).
Pix fimbriae
Pix fimbriae are a relatively rare fimbrial type found in 8% of UPEC compared to 4% of fecal E. coli strains (Spurbeck et al., 2011). Pix fimbriae were initially discovered on a pathogenicity island found in the uropathogenic E. coli strain X2194 (Lugering et al., 2003). Pix fimbriae are related to P fimbriae, but are differentially regulated due to a truncation in the regulatory region associated with P fimbriae. Expression of Pix was found to be temperature-dependent during stationary phase. Pix fimbriae promote adherence to HeLa cells (Lugering et al., 2003), demonstrating that these fimbriae, although rare, can be an important virulence factor for some UPEC. The importance of Pix fimbriae has yet to be tested in the mouse model of ascending UTI.
Dr/Afa fimbriae
Dr and Afa adhesins are a family of E. coli adhesins that recognize the Dr blood group antigen (a cell membrane protein in erythrocytes also called the decay-accelerating factor) as their receptor (Nowicki et al., 1990). While some Dr family adhesins are afimbrial adhesins, such as AFA-I and AFA-III, others are fimbrial adhesins like F1845 and Dr fimbriae (Nowicki et al., 1990). The best-studied member of the Dr family of adhesins, the Dr fimbriae (aka O75X), are found in 7% of UPEC compared to 2% of fecal E. coli strains (Spurbeck et al., 2011) and bind type IV collagen in vitro (Westerlund et al., 1989). In vivo, Dr fimbriae bind to peritubular connective tissue in the human kidney (Nowicki et al., 1986), the connective tissue between muscle cell layers in the bladder, human neutrophils (Johnson et al., 1995), and weakly to epithelial cells (Virkola et al., 1988). Dr fimbriae also facilitate cell invasion (Goluszko et al., 1997; Fang et al., 2004; Das et al., 2005), allowing bacteria to avoid the humoral immune response by hiding within the intracellular space. Vaccination with purified Dr fimbriae reduced mortality associated with UTI in a mouse model of infection (Goluszko et al., 2005), however, since the majority of UPEC do not encode these fimbriae, vaccination against Dr fimbriae would prevent only a minor subset of UPEC infections.
Putative fimbriae (Yeh, type IV pili 1 and 2, Yfc)
The presence of two putative fimbriae encoded by E. coli CFT073, Yfc fimbriae (encoded by yfcOPQRSUV, present in 73% UPEC isolates v. 33% fecal isolates) and type IV pilus 2 (encoded by c2394-c2395, present in 51% UPEC v. 12% fecal isolates), are correlated with uropathogenic isolates (Spurbeck et al., 2011). However, Yeh fimbriae (encoded by yehABCD, present in 94% of E. coli isolates) and type IV pilus 1 (encoded by ppdD, hofB, and hofC, present in 99% of E. coli isolates) are found ubiquitously in the E. coli population, and are therefore, not markers of pathogenic isolates.
Regulatory hierarchy of fimbrial expression
Regulation of type 1 fimbriae has been the focus of several studies, since this is one of the major factors required for virulence in the murine model of ascending UTI. The promoter of the type 1 fimbrial operon is located on an invertible sequence (designated the fim switch) and causes type 1 fimbriae to be phase-variable. Phase variation is dependent on the activity of two recombinases, FimB and FimE. FimB can reverse the orientation of the fim switch from either the on-to-off or off-to-on direction, whereas FimE primarily works only in the on-to-off direction. Therefore, any regulation that changes the ratio of these recombinases or enhances or suppresses their activity, will affect the proportion of E. coli expressing type 1 fimbriae. P and S fimbriae can affect the expression of type 1 fimbriae via inhibition of FimB activity, which then forces the fim switch into the off position from the action of FimE (Xia et al., 2000; Holden et al., 2001, 2006). The negative regulation of type 1 fimbrial expression by F1C, S and P fimbriae is due to regulatory proteins, FocB, SfaB, or PapB, associated with the respective fimbrial operons (Xia et al., 2000; Holden et al., 2001; Lindberg et al., 2008). Furthermore, P fimbriae positively regulate the expression of S fimbriae (Morschhauser et al., 1994), suggesting that both fimbriae are utilized by the bacteria to colonize the same niche. When taken together with the negative regulation of type 1 fimbriae, the hierarchy of regulation begins to appear such that while type 1 fimbriae are needed for adherence in an earlier niche, such as in the bladder, they may hinder adherence later, such as in the kidneys, where P and S fimbriae are utilized. This type of hierarchical regulation could be essential for a pathogen like UPEC to ensure that only fimbriae necessary for adherence to a particular substrate are expressed at any given time, as well as to limit exposure of each antigen to the immune system, allowing the pathogen to avoid clearance brought on by an antibody response. How the uncharacterized fimbriae feed into this regulatory system remains to be elucidated.
Non-fimbrial adhesins
Factor adherence E. coli: FdeC
FdeC is an ExPEC adhesin that is found in 99% of ExPEC, 82.6% of commensal, and 93–100% of intestinal pathogenic E. coli strains (Nesta et al., 2012). FdeC is only expressed upon contact with host cells and has structural similarity to intimin (from enteropathogenic E. coli) and invasin (from Yersinia pseudotuberculosis), two bacterial virulence factors that mediate host cell interactions. Recombinant FdeC binds epithelial cells and collagens V and VI (both present in the human urinary tract) with high affinity and, in vivo, E. coli 536 wild-type significantly outcompetes a fdeC mutant in colonization of the bladder and kidneys (Nesta et al., 2012). Thus, FdeC is a non-fimbrial adhesin necessary for full urovirulence.
RTX-like adhesin: TosA
TosA is a repeat-in-toxin (RTX) protein highly associated with UPEC (Vigil et al., 2011b). Originally thought to encode a toxin due to sequence similarity with other RTX proteins such as hemolysin, tosA was of interest to researchers studying the pathogenesis of UPEC for two reasons. First, tosA is encoded on a pathogenicity island that, when deleted, rendered E. coli CFT073 attenuated in the mouse model of ascending UTI (Lloyd et al., 2009a).Upon deletion of tosA itself, the deletion strain was outcompeted in vivo, demonstrating that the attenuation of the PAI deletion strain was due, at least in part, to the deletion of tosA (Lloyd et al., 2009a). Second, tosA was hit in an IVIAT screen, a method for identification of antigenic proteins that are expressed only in vivo (Vigil et al., 2011a). TosA is outer membrane-associated in E. coli, but does not have a cytotoxic effect on mammalian cells (Vigil et al., 2012). Rather, when over-expressed, TosA increases adherence of E. coli CFT073 to kidney epithelial cell lines, suggesting that TosA acts as an afimbrial adhesin mediating intimate attachment to the upper urinary tract (Vigil et al., 2012).
Autotransporters
Autotransporters are proteins that undergo type V secretion (Chapter 16), and can either be secreted into the extracellular mileu or remain attached to the bacterial cell surface. Eleven autotransporter-encoding genes have been identified in the uropathogenic E. coli strain CFT073 (Parham et al., 2004; Allsopp et al., 2010). Some, like Sat, are secreted toxins, but most autotransporters in UPEC remain membrane-associated. Here we discuss the autotransporter proteins that enhance adherence to uroepithelial cells or biofilm formation.
UpaG
UpaG is associated with E. coli phylogroups B2 and D, which include the uropathogens (Valle et al., 2008; Totsika et al., 2012). UpaG mediates adherence to human bladder epithelial cells in vitro (Valle et al., 2008; Totsika et al., 2012) and promotes cell aggregation, biofilm formation, and binding to the human extracellular matrix proteins fibronectin and laminin (Valle et al., 2008). However, when tested in the mouse model of ascending UTI, a upaG deletion mutant had no fitness defect when compared to wild-type E. coli CFT073 (Valle et al., 2008), suggesting that the role of this protein during infection is too subtle to be detected as a fitness defect.
UpaH
UpaH is highly prevalent in all E. coli strains tested, as it is found in 86% of A, 85% of B1, 84% of B2, and 70% of phylogenetic group D (Allsopp et al., 2010). However, in E. coli K-12 the pseudogene encoding UpaH, ydbA, is truncated by insertion sequences (Allsopp et al., 2010). In wild-type E. coli CFT073, UpaH promotes biofilm formation on abiotic surfaces in M9 minimal medium. UpaH is also expressed in vivo during infection and the upaH deletion strain was significantly outcompeted by wild-type E. coli CFT073 in the mouse bladder, demonstrating that this autotransporter adhesin is involved in uropathogenesis (Allsopp et al., 2010). However, in two other UPEC strains that express UpaH there was no significant defect in colonization when upaH was deleted. Thus, UpaH appears to be a strain-specific virulence factor (Allsopp et al., 2010).
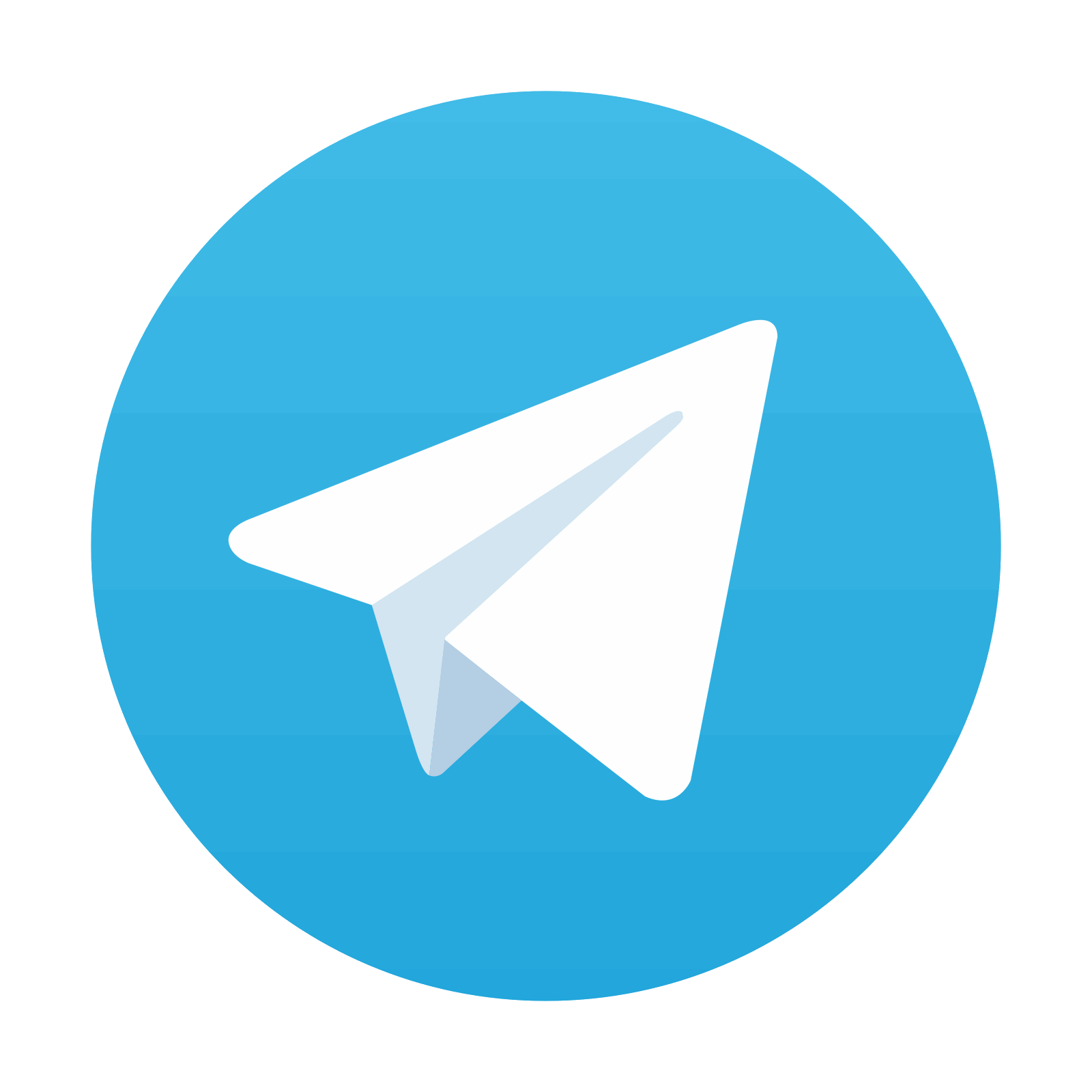
Stay updated, free articles. Join our Telegram channel

Full access? Get Clinical Tree
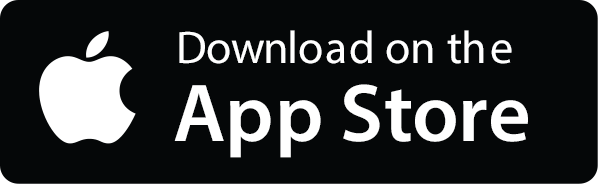
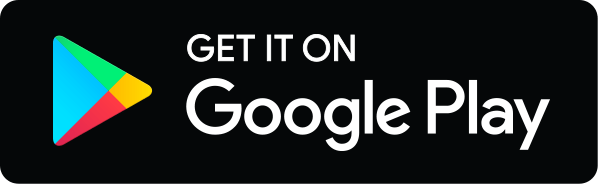