Chapter 25 Kartik Venkatachalam1,*; Kirill Kiselyov2,* 1 Department of Integrative Biology and Pharmacology, University of Texas School of Medicine, Houston, TX, USA TRPML1 is a cation channel belonging to the transient receptor potential (TRP) superfamily whose localization is commonly assigned to late endosomes and lysosomes due to the presence of the lysosomal localization signals on its C- and N-termini. Because mutations in the TRPML1-coding gene MCOLN1 are responsible for the lysosomal storage disease mucolipidosis type IV (MLIV), the majority of research into this ion channel has been focused on its role in preventing the buildup of undigested material in the endolysosomal pathway. Recent data suggest that TRPML1 contributes to processes beyond the degradative, recycling, and sorting functions of late-endosomes and lysosomes. Indeed, modulation of endolysosomal function using TRPML1 activators and inhibitors promises new approaches to such conditions as cancer, immune disorders, and neurodegenerative diseases. This chapter is focused on processes shown or inferred to depend on TRPML1 and predicting new uses for modulating activity of this interesting channel. This work was supported by the National Institutes of Health grant RO1HD058577 to KK, and R01NS081301 to KV. Although MLIV is considered a developmental brain disorder with a slowly progressing degenerative component [1–10], the fact that TRPML1 is ubiquitously expressed suggests a role outside the central nervous system (CNS), which is further supported by the findings of gastric and pancreatic involvement in human MLIV patients and mouse models [11–14]. Beyond its wide tissue distribution, TRPML1 appears to regulate an array of cellular processes pertaining to the function of late endosomes and lysosomes. The latter notion reflects our growing realization that far from being just a cellular digestion and absorption center, lysosomes are important cellular signaling and cell-fate hubs. Recent evidence linked lysosomes to regulation of transition metal toxicity and revealed their role in key physiological processes such as aging, antigen processing, and mitophagy, as well as autophagy in general [15–19]. Furthermore, the findings that lysosomal gene network responds to stress and amino acid scarcity via mechanistic target of rapamycin (MTOR), and transcription factors such as transcription factor EB (TFEB) suggest that lysosome-dependent processes are actively regulated depending on the physiological state of cells [20–24]. Previously published results suggest that TRPML1 regulates endocytic membrane traffic, lysosomal exocytosis, metal distribution, phagocytosis, and autophagy [25–29]. Drugs that affect TRPML1 function may provide new possibilities for treating conditions in which lysosomal function is a factor. With this in mind, here we will focus on TRPML1-dependent processes outside neurodevelopment and neurodegeneration. Such processes have been inferred based on the different aspects of TRPML1 function. We aim to delineate TRPML1 activators and inhibitors as potential treatments for various pathophysiological conditions. TRPML1 (Mucolipin 1) is a six-transmembrane-domain containing ion channel, a member of the TRPML family of the TRP channels [25,27]. It is coded by the MCOLN1 gene, which in chordates has two additional isoforms encoding TRPML2 and TRPML3 [30,31]. Although both TRPML2 and TRPML3 appear to function in the endocytic pathway, there are no known human diseases related to mutations in genes encoding either TRPML2 or TRPML3. Invertebrates have a single trpml gene, with closest homology to MCOLN1 [28,30]. The trpml gene family evolution has been recently discussed in a series of excellent reviews [28,30]. Although the different TRPMLs form heteromultimers [32], the range of tissues expressing TRPML2 and TRPML3 is reported to be very narrow [33,34], and thus the functional significance of such multimerization remains elusive. It should be noted that our knowledge of signals regulating TRPML2 and TRPML3 expression is very limited, and it is indeed possible that heteromultimers exist under certain conditions of cell stimulation or developmental stages. In humans, TRPML1 is a 540-amino-acid protein, with a putative pore region between the fifth and sixth transmembrane domains [25,27]. Additional splice variants of TRPML1 have not been described. Some lysosomal proteins that conduct ions appear to combine the functions of channels and transporters (e.g., Cl− permeation coupled with H+ transport by the ClC channels/transporters) [35]. Transporter function of TRPML1 has not been described and it is treated a channel. Mammalian TRPML1 has lysosomal localization signals in both its C- and N-termini that have been shown to be critical for its lysosomal localization via an AP-1-dependent mechanism [36,37]. These domains are poorly conserved, as they appear to be absent in the invertebrate or even fish TRPMLs, albeit the evidence pinning invertebrate TRPML1 to the endolysosomes is overwhelming. These differences likely reflect the evolutionary constraints driving the functional/spatial specialization within this family. The N-terminus of TRPML1 contains a domain that binds phosphoinositides (PI) [38,39], and this domain is evolutionarily extremely well conserved (Figure 25.1). The PI-binding domain was shown to bind the lysosomal lipid marker PI(3,5)P2 leading to TRPML1 activation, whereas its binding to the plasma membrane lipid PI(4,5)P2 was shown to inhibit the channel activity [38]. This provides a nearly perfect structural reflection of the TRPML1 functional context as TRPML1 travels to the lysosomes via the plasma membrane; its premature activation is inhibited by the PI(4,5)P2 binding until it is delivered to the PI(3,5)P2-rich lysosomes. Conversely, the inhibition by PI(4,5)P2 serves as a mechanism to abrogate channel activity following lysosomal exocytosis—a process driven by TRPML1 channel activity. The PI-dependence of TRPML1 raises some interesting questions. First, if PI(3,5)P2 is the TRPML1 activator and its lysosomal levels are fairly stable, then is TRPML1 a lysosomal leak channel activated on its delivery to the lysosomes? Or do lysosomal PI(3,5)P2 levels and/or spatial distribution change, and if so, what are the signals and machinery actuating these changes? Finally, the PI-binding domains are very similar within the TRPML family, yet both localization and function of TRPML3 was shown to be different from that of TRPML1 due to wider distribution in the endocytic pathway, including early endosomes [40,41]. Whether or not TRPMLs respond to PI species other than PI(3,5)P2 is currently unknown. Another unique feature of TRPML1 is a large extracytosolic loop between its first and second transmembrane domains. The loop is cleaved by a protease that is sensitive to cathepsin B inhibitors, but neither the identity of the cleavage site nor its functional significance has been unequivocally established [42]. The loop contains large numbers of histidine residues: 75% of the TRPML1 histidines are in that loop, whereas the loop is only 25% of the molecule’s length. In TRPML3 these histidines were shown to participate in this channel’s inhibition by low pH but their functional significance for TRPML1 is unknown. It is worth noting that the Zn transporters, ZnT (Slc30) and Zip (Slc39), also contain similar structures, which appear to be essential for Zn transport [43,44]. It is tempting to speculate that the TRPML1 histidine stretch is important in the context of Zn regulation by TRPML1 (or perhaps TRPML1 regulation by Zn), which will be discussed later. Electrophysiological inquiry into TRPML1 permeability properties began with analysis of human recombinant proteins in planar lipid bilayers and in whole cell patch clamp, but the field only matured after the whole-lysosome recording technique was established [45]. The latter approach revealed inwardly rectifying (from lysosomal lumen into the cytoplasm) ion channel permeable to monovalent and divalent cations including K+, Na+, Ca2 +, Fe2 + and Zn2 + [45]. The wild type channel is about equally selective to Na+ and Ca2 +, which means that at the presumed lysosomal Na+ and Ca2 + concentrations (about 50:1 ratio), TRPML1 efficacy of releasing lysosomal Ca2 + is about the same as the efficacy of a plasma membrane TRPC channel maintaining receptor-mediated Ca2 + influx. Genetically engineered Ca2 + probes report increased cytoplasmic Ca2 + in the immediate proximity of TRPML1 [39]. In one report TRPML1 overexpression and loss failed to appreciably change total lysosomal Ca2 +, suggesting that TRPML1 deals with a small amount of lysosomal Ca2 +, probably effecting changes in its immediate proximity [46]. Indeed, localized Ca2 + signaling appears to be the theme of this aspect of its proposed function. That being said, the loss of Drosophila TRPML does result in elevated levels of lysosomal Ca2 + [47]. These differences could reflect the fact that Drosophila expresses a single TRPML homolog in contrast to the three genes in mammalian cells. Electrophysiological recordings of the sole TRPML gene in Drosophila reveal a channel reproducing most physiological features of human TRPML1, including its regulation by pH and Ca2 + permeability [48]. At present, the functional overlap between Drosophila TRPML and the three mammalian TRPMLs remains unknown. TRPML1 activity is increased by low pH, in agreement with its function in the lysosomes [45]. The development of TRPML1 patch clamp enabled screening for additional modulators of its activity, leading to identification of SF-22 and ML-SA1 as its activators and sphingomyelin as an inhibitor [49,50]. It is clear that delineating the wider range of TRPML1-dependent cellular functions will stimulate the search for additional compounds modulating TRPML1 activity as such compounds could serve as drug candidates for a range of conditions. Identification of TRPMLs as members of TRP superfamily, the majority of which are Ca2 +-permeable channels, narrowed the focus of the search for TRPML1 function to Ca2 +-dependent events driving the SNARE-mediated fusion of the endocytic membranes. The current paradigm of the TRPML1-dependent aspects of membrane traffic suggests that Ca2 + release from the lysosomes via TRPML1 actuates conformational change in SNARE driving the homotypic and heterotypic membrane fusion events. Membrane traffic delays at the prelysosomal stage and suppressed fusion of the lysosomal and autophagosomal/amphisomal compartments have been shown in human MLIV fibroblasts, murine macrophages, and Drosophila cells lacking trpml [49,51–53]. The membrane traffic model has recently been developed into the idea that TRPML1 participates in lysosomal exocytosis by promoting the fusion of lysosomes with the plasma membrane [54–56]. This process emerged as an important mechanism for plasma membrane repair [57]. Lysosomal exocytosis has also been implicated in transition metal detoxification including the excretion of Zn2 + and Cu2 + from cells [18,19]. Up-regulation of lysosomal exocytosis via TFEB overexpression has been used to clear storage material and suppress cell death in several lysosomal storage disease models [16,58,59]. From all these findings, it is safe to conclude that lysosomal exocytosis is an important cytoprotective mechanism. The proposed role of TRPML1 in the lysosomal membrane fusion events raises several questions including the mechanism triggering Ca2 + release from the lysosomes, lysosomal maturation before fusion/exocytosis, and indispensability of specifically TRPML1-mediated Ca2 + release for this process. At the same time, a definitive role of TRPML1 in this process means that TRPML1 activators and inhibitors may be used to modulate cell repair and detoxification. In the C. elegans MLIV model, deletion of TRPML1 ortholog CUP-5 delayed reformation of the lysosomes from hybrid organelles [60–62], which is somewhat similar to postlysosomal lipid traffic deficits reported in an siRNA-driven acute human TRPML1 knockdown model [63]. A role of Ca2 + channels in membrane fission has not been shown, and thus it remains unclear what role TRPML1 would play in this process. Postlysosomal lipid traffic delays can be explained by incomplete processing of the endocytosed material resulting in unsuitability of the degradation products in TRPML1-deficient cells with the lysosomal transporters. Although diminished enzymatic activity was shown in MLIV fibroblasts [46,64], biochemical characterization of storage material in MLIV cells has not been consistent. The lack of a comprehensive biochemical account of storage material and lysosomal enzymatic profiling of TRPML1-deficient cells is a serious impediment to understanding the function of this ion channel in lysosomes. As TRPML1 regulates the endolysosomal membrane fusion, it may modulate the degradative function of lysosomes by driving the delivery of enzymes and transporters to lysosomes (Figure 25.2). Some initial experiments suggested lysosomal enzyme mislocalization in MLIV cells, but this model was not developed further [64], and to this day no comprehensive analysis of the lysosomal enzymatic and transporter profile as a function of TRPML1 status has been published. Macroautophagy deficits were shown in MLIV patients’ fibroblasts and Drosophila models [47,53,65–67]; because macroautophagy relies on membrane fusion events its suppression has been explained by delayed fusion of autophagosomes with lysosomes in TRPML1-deficient cells. Interestingly, chaperone-mediated autophagy appears to be defective in human MLIV patients’ fibroblasts as well [68]. In contrast to macroautophagy, chaperone-mediated autophagy involves chaperone-dependent translocation of proteins from cytoplasm into the lysosomes [69]. In addition to chaperones, it requires a translocator complex comprising several proteins including lysosomal associated membrane protein-2 (LAMP-2A). It will be interesting to answer whether or not localization of the translocator complex depends on TRPML1 and whether it is abnormal in cells affected by MLIV. Whereas the role of ion channels in membrane traffic is widely discussed, direct evidence linking ion channel activity with intracellular localization of a specific transporter or another transmembrane protein is lacking. A finding that TRPML1-deficient cells have abnormal distribution of lysosomal enzymes or transporters would set a precedent for a novel function of ion channels. An alternative mechanism involving TRPML1 in lysosomal degradation is via regulation of the activities of lysosomal enzymes and transporters. The initial findings that MLIV is associated with suppressed lysosomal enzymatic activity and the subsequent findings that lysosomal acid lipase activity is suppressed in MLIV fibroblasts [46,64] were not followed up, and thus the extent of the lysosomal malfunction in MLIV is not really known. TRPML1 may regulate the lysosomal enzymatic activity by regulating different aspects of the lysosomal ion homeostasis such as pH or metal content. TRPML1 was suggested to prevent lysosomal acidification [46,63], a notion that is indirectly supported by the recent evidence that proper maintenance of the lysosomal pH requires H+ leak or a monovalent cation channel [70,71]. Lysosomes are critical for Fe absorption in the majority of tissues as lysosomes are involved in processing the protein-bound Fe, and its subsequent extrusion into the cytoplasm [72]. Furthermore, recent evidence shows that lysosomes are also indispensable for sequestration and exocytosis of cytoplasmic Zn2 + and Cu2 + [18,19]. Indeed, loss of lysosomal metal uptake and exocytosis significantly increases metal toxicity. The idea that TRPML1 regulates lysosomal metal content emerged with the evidence of TRPML1 permeability to Fe and, therefore, Fe buildup in MLIV fibroblasts [45]. In subsequent studies, it was shown that TRPML1-deficient cells exposed to Fe have higher levels of oxidative stress and mitochondrial damage than normal cells treated similarly [73]. This too can be explained by the lysosomal Fe buildup in TRPML1-deficient cells. In addition to damaging lysosomal membrane, which is a likely contributor to the leak of the lysosomal digestive enzymes into the cytoplasm in TRPML1-deficient cells, reactive oxygen species generated by the excess lysosomal Fe may damage lysosomal digestive enzymes or render endocytosed material and products of its digestion unsuitable for degradation and absorption. Zn is another transition metal whose handling appears to depend on TRPML1, as two groups demonstrated its buildup in TRPML1-deficient cells [74,75]. Although Zn may enter lysosomes via endocytosis or by autophagy of Zn-rich organelles such as mitochondria and secretory granules, the evidence that TRPML1-deficient cells in which Zn transporter ZnT4 was knocked down do not accumulate lysosomal Zn suggest that lysosomes take up Zn from the cytoplasm and TRPML1 is involved in dissipation of the lysosomal Zn via exocytosis or its release into the cytoplasm [74]. The mechanisms underlying the regulation of lysosomal metals by TRPML1 are unclear. On one hand, TRPML1 has been shown to be permeable to Fe and Zn [45]. Although brain Fe was not consistently surveyed in MLIV patients or mouse model, a deficient brain Fe delivery would be compatible with the hypomyelination suggested in MLIV [10]. Fe is required for oligodendrocyte maturation and for myelin production by these cells [76,77]. Although hypomyelination is not a defining feature of MLIV and, indeed, it has been shown in several lysosomal storage diseases, the recent evidence points that TRPML1 is inhibited by sphingomyelin [49]. This lipid is a common secondary storage component in lysosomal storage diseases; therefore, it is possible that the loss or suppression of TRPML1 activity followed by drop in brain Fe uptake is a factor in other storage diseases. Accordingly, TRPML1 activation by chemical means alleviated the key aspects of Niemann-Pick C1 phenotype, a disease not directly caused by the TRPML1 loss [49]. A detailed survey of brain Fe dynamics in MLIV human patients as well as human patients and mouse models of other storage diseases would be very helpful in elucidating the role if TRPML1 in handling this vital ion at the organism level. Regarding the mechanism of TRPML1-dependent brain Fe uptake, TRPML1 Fe permeability could be particularly important in cells that appear to lack the divalent metal transporter 1 (DMT1) transporter—another component of Fe-handling machinery [72]. Among such cells are brain capillary endothelial cells, which are essential for brain Fe uptake. On the other hand, brain capillary endothelial cells were proposed to move Fe via transcytosis: capture of the extracellular material at the apical surface of the cell, followed by its transport across the cell and by the release of endocytic content via endo/lysosomal fusion with the basal membrane [78,79]. This is compatible with the TRPML1 role in the lysosomal secretion. It could be argued that intracellular and lysosomal-free Zn levels are too low (estimated to be in the picomolar range) for these cations to pass through an ion channel. However, the presence of the histidine-rich loop between the first and second transmembrane domains on TRPML1 could play a role in conferring Zn permeability to these channels. Interestingly, these regions exhibit similarity with similar domains in ZnT and Zip transporters, in which such loops were suggested to assist in Zn permeation by assisting in the transfer of Zn from other Zn-binding proteins to the transporters [43,44]. However, a role of TRPML1 in localization of other Zn transporters, such as a lysosomal Zip (which would export Zn from the lysosomes into the cytoplasm) or DMT1 cannot be ruled out at this moment. This, and an analysis of brain Zn as a function of TRPML1 status awaits further investigation. It should be noted that some of the most fascinating aspects of the lysosomal function lie beyond degradation and absorption of the endocytosed material, and thus modulation of TRPML1 activity using pharmacological means could be key to a range of processes and conditions. Next we outline some of the processes in which TRPML1 involvements has not been demonstrated, but can be predicted using the present models of the TRPML1 function and the current knowledge of the endocytic pathway. In addition to nutrient uptake and plasma membrane repair/remodeling, the endocytic pathway modulates the processing of growth factor receptors via its endocytosis followed by ligand dissociation and receptor degradation or recycling [80,81]. Beyond a possible role of TRPML1 in the membrane fusion in the latter endocytic pathway, the recent evidence that TRPML1 regulates lysosomal exocytosis and endo/phagocytosis [54–56] suggests that it may be involved in growth factor receptor handling. Whether or not TRPML1 activators and inhibitors affect cell growth and proliferation is an open question. Similarly, antigen processing and presentation depends on both degradative and sorting/trafficking functions of the lysosomes [82]. Based on the predicted function of TRPML1 in the endocytic membrane traffic, this suggests immune system involvement in MLIV. No consistent information on such an involvement in MLIV patients is available, and thus it would be important to establish whether or not it is a factor in MLIV patients and model mice. Interestingly, genome-wide RNAi screen in S2 cells for microbial clearance revealed fly trpml as one of the few hits for diminished degradation of bacteria [83]. ESCRT family members (proteins responsible for formation of intraluminal vesicles in multivesicular bodies [84]) were also involved, suggesting an interaction between TRPML function and the formation of multivesicular bodies. Directly showing that TRPML1 is involved in antigen processing and presentation would suggest that its activators and inhibitors could be novel candidates for treating immune and autoimmune diseases. Exosomes are extracellular vesicles secreted by some cell types. They have been implicated in establishment of the metastatic loci and in cell death in neurodegeneration [85–88]. Evidence suggests that exosomes are luminal vesicles of the multivesicular bodies secreted due to their fusion with the plasma membrane [89–91]. If TRPML1 is involved in formation of multivesicular bodies and/or their exocytosis, then it is possible that TRPML1 regulates exosome secretion. Keeping in mind their significance in cancer and neurodegenerative diseases, it would be important to establish whether not there’s a role of TRPML1 in exosomal secretion. Beyond that, a suggestion of non-cell-autonomous component of cell loss in storage diseases is very appealing because it would justify completely novel approaches to them. Beyond the digestive and sorting function of the endocytic pathway, TRPML1 appears to be an important component of the energy-sensing signaling circuit actuated by MTOR (mechanistic target or rapamycin). MTOR is a kinase responsible for translating the cellular energy status into activation of cellular circuits regulating metabolism, growth, and motility [24,92–94]. The recently demonstrated requirement for TRPML1 function for MTOR [47] suggests that the aspects of the endocytic function regulated by TRPML1 are read by MTOR suggesting that both molecules are involved in the same signaling pathway regulating key aspect of cellular function. It should be noted that MTOR dysregulation has been linked to several forms of cancer, potentially linking TRPML1 to this condition [95–97]. Further highlighting the potential role of TRPML1 in cancer is its role in regulation of lipid traffic and metabolism. Several types of lipids have been implicated in regulating cell proliferation, and thus regulation of lipid handling by TRPML1 is likely an important aspect of cell cycle regulation and, potentially, cancer research. The processing of several toxins such as shiga, cholera, botulinum, and diphtheria toxins depends on trafficking along the endocytic pathway [98–103]. Parasite invasion itself depends on membrane traffic as well. Indeed a role of TRPML1 activator PI(3,5)P2 in Plasmodium berghei invasion has been recently shown [104]. Although we still need to establish whether or not TRPML1 function affects parasite invasion or microbial toxicity, a role of TRPML1 in membrane traffic suggests that modulators of its activity could serve as antiparasitic drugs. As discussed earlier, roles of TRPML1 in both transition metal permeability and membrane traffic may underlie its utility in metal handling. Fe uptake depends on its dissociation from transferrin in late-endosomes/lysosomes, which in turn is a function of membrane traffic and organellar pH [72]. If TRPML1 regulates either of them, it may play key role in the Fe absorption process. The transfer of Fe across the lysosomal membrane depends on the conversion of ferric iron to divalent ferrous Fe. Interestingly, TRPML1 is permeable to Fe2 + but not to Fe3 + [45]. Although in the majority of tissues DMT1 is presumed to be the lysosomal Fe exporter, some tissues lack DMT1 [78,79]. Furthermore, DMT1 and TRPML1 appear to be regulated by different signaling circuits, as DMT1 does not appear to belong to the lysosomal gene network. It is possible that TRPML1 substitutes DMT1 in some tissues or under certain conditions such as during oxidative stress or during phases of development requiring Fe, such as myelination in the CNS. Whether TRPML1 regulates Fe by conducting it or by ensuring its delivery via membrane traffic, modulating its activity could be an important tool for modulating developmental processes. Fe excess is toxic due to the role of Fe in catalysis of ROS production via Fenton-type reactions. Indeed, Fe toxicity is a factor in neurodegenerative diseases, cell death during stroke, and the aging-related suppression of the lysosomal/autophagic function [105–110]. It was shown that, in aged cells, suppression of autophagy due to Fe-dependent accumulation of lipofuscin is associated with the toxic buildup of aged, dysfunctional mitochondria [111]. Accordingly, autophagy and mitophagy deficits were shown in MLIV, including human fibroblasts and mouse and Drosophila models [47,53,65–67], and a link between autophagic deficits and cell death has been proposed [112]. Beyond autophagic deficits, Fe buildup in TRPML1-deficient cells appears to be associated with oxidative stress and mitochondrial damage [73]. It is, therefore tempting to suggest that TRPML1 activators or increased expression may alleviate some of the effects of aging and oxidative stress. Zn2 + is a key element in numerous aspects of cellular and systems processes including gene expression, enzymatic activity, and neurotransmission [113,114]. The importance of this ion is emphasized by the fact that about twice as many genes code for proteins dedicated to regulation of Zn2 + than Ca2 +. The previously published and recent evidence link lysosomes to the regulation of cellular Zn, and indeed key biological processes such as cell death during mammary gland involution appear to depend on Zn uptake by the lysosomes. The role of Zn in the mammary gland involution has been recently reviewed [114]; it will be interesting to establish whether or not TRPML1 is involved in regulation of Zn in other involuting tissues such as ovaries and uterus. Whether TRPML1 is involved in the dissipation of the lysosomal Zn, its exocytosis, or localization of the lysosomal transporters, it is important to note that involution was suggested to clear premalignant cells, and thus TRPML1 activators and inhibitors may play a role in defense against cancer. Since its discovery, the search for TRPML1 function has been focused on the CNS. However, the examples outlined earlier are just a glance at the entire range of processes predicted to be regulated by TRPML1. Although TRPML1 has been shown to play a role in neuronal, gastric, and muscle function, it is clear that its repertoire is much wider. Its central role in the lysosomal biogenesis, traffic, or ion homeostasis makes it an almost inescapable conclusion that those processes that depend on lysosomal membrane traffic or ion homeostasis are regulated by TRPML1. Such processes include growth factor processing, transition metal uptake, and recycling of aged organelles. These processes are solidly established to drive neurodevelopment, aging, and cancer, and thus, based on the aspects of the cellular function in which TRPML1 has been previously implicated, we propose that TRPML1 activators and inhibitors promise new approaches to a range of conditions likely including such key processes as development, cancer, and aging.
TRPML1-Dependent Processes as Therapeutic Targets
2 Department of Biological Sciences, University of Pittsburgh, Pittsburgh, PA, USA
* Corresponding authors: kartik.venkatachalam@uth.tmc.edu, kiselyov@pitt.edu
Abstract
Acknowledgments
Introduction
TRPML1 Features and Properties
TRPML1-Dependent Processes, Shown and Discussed
TRPML1-Dependent Processes Inferred from its Functional Context
Conclusions
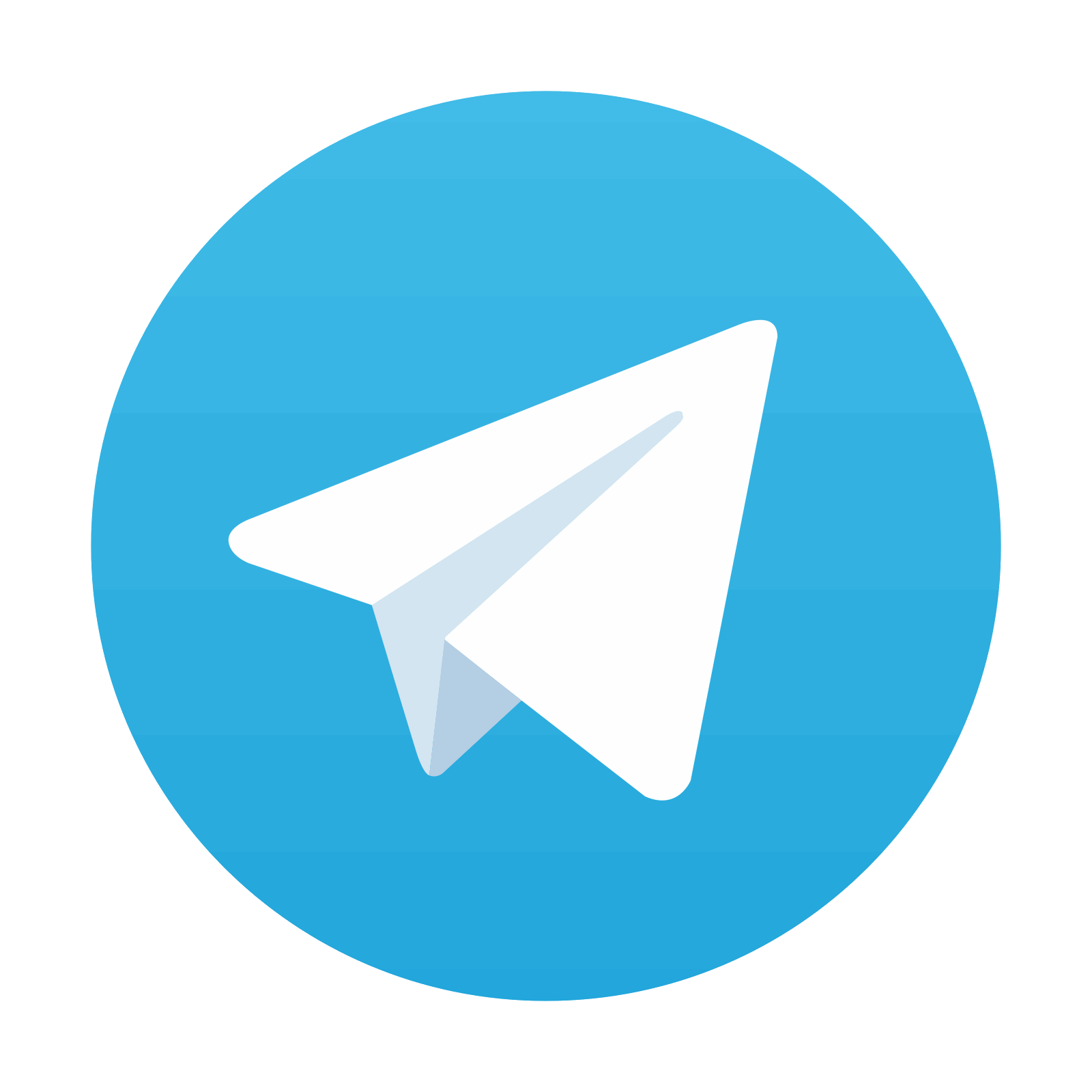
Stay updated, free articles. Join our Telegram channel

Full access? Get Clinical Tree
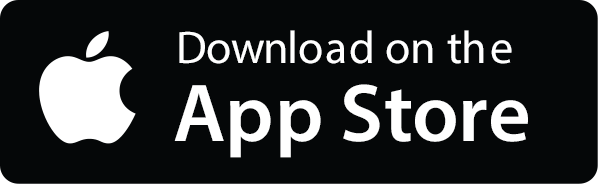
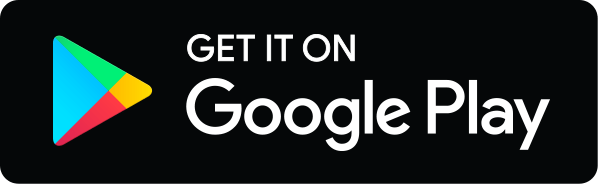