Chapter 3 Mahendra Bishnoi1; Pragyanshu Khare1; Kanthi K. Kondepudi2; Louis S. Premkumar3,* 1 Department of Nutritional Sciences and Technology, National Agri-Food Biotechnology Institute (NABI), SAS Nagar, India The transient receptor potential vanilloid 1 (TRPV1) is a nonselective cation channel with high calcium permeability that has been studied extensively since its identification and characterization in 1997. Its involvement in different modalities of pain has been well documented, which has resulted in the development of novel strategies for the treatment of pain. However, TRPV1 is also involved in a plethora of other physiological and pathophysiological functions related to urinary, cardiovascular, gastrointestinal, respiratory, and central nervous systems. Here, we summarize the recent developments of the role of TRPV1 in acquired diseases and review the current perspective of TRPV1 agonist and antagonist as potential drugs for therapeutic intervention. This work is supported by the grant from NIDA (DA028017). TRPV1, the first member of the transient receptor potential vanilloid (TRPV) family of ion channels was cloned in 1997 by David Julius and colleagues [1]. TRPV1 is a 95-kDa, 838 amino acid protein consisting of six transmembrane (TM) segments with a pore-forming loop between the fifth and sixth TM segments and intracellular N- and C-terminals. The stoichiometry of TRPV1 is considered to be a homo- or heterotetramer. Recently, using electron cryomicroscopy, the detailed structure and possible allosteric modulation during activation of mammalian TRPV1 channel have been determined. TRPV1 exhibits fourfold symmetry around a central ion pore formed by TM segments 5-6 (S5-S6) with an open extracellular “mouth” with a short selectivity filter. Subunit organization is influenced by interactions among amino-terminal ankyrin repeats present in cytoplasmic domains. The opening on activation is associated with major structural changes in the outer pore and a pronounced dilation of a hydrophobic constriction at the lower gate, suggesting a dual-gating mechanism. This allosteric coupling between upper and lower gates may account for the rich physiological modulations exhibited by TRPV1 [2,3]. TRPV1 is expressed in sensory and nonsensory neurons and in nonneuronal cells. Neurons expressing TRPV1 are small to medium diameter and peptidergic, which gives rise to unmyelinated C-fibers and thinly myelinated A-δ fibers. These are mainly expressed in a subset of dorsal root ganglion (DRG), trigeminal ganglion (TG), and nodose ganglion neurons [1,4]. TRPV1 is expressed on both the peripheral and central terminals of the sensory neurons [1,5,6]. TRPV1 is expressed in various nonneuronal cells [7] including mast cells [8], epithelial cells of urinary bladder [9], stomach [10], palate [11], and airway [12–14], skin epidermal keratinocytes [15], hematopoietic cells [16] and preadipocytes [17]. On the basis of its widespread tissue distribution, loss- or gain-of-function can be associated with a variety of acquired diseases. Such effects can be brought about by changes in transcriptional, translational, and posttranslational modifications. The channels can be robustly sensitized by phosphorylation and undergo desensitization on overactivation by exogenous and endogenous ligands. This chapter focuses on role of TRPV1 ion channel in acquired disorders and development of TRPV1 modulators as therapeutic agents. Preclinical research has identified TRPV1 as an important target for developing novel analgesics. TRPV1 gene knockout mice are partially devoid of the thermal inflammatory hyperalgesia [18,19]. TRPV1 plays a central role in mediating peripheral [20] and central sensitization [21]. TRPV1 is involved in triggering central sensitization during repetitive C-fiber firing by releasing neuropeptides and neurotransmitters, which results in activation of glia [22–24]. One of the mechanisms involved in the peripheral sensitization is due to phosphorylation of the receptor, which robustly potentiates the channel [25–27]. Both TRPV1 antagonists and agonists are capable of inducing pain relief. As expected, TRPV1 antagonists prevent the generation of receptor potential in response to temperature or endogenous and exogenous ligands. However, while using potent exogenous agonists, the generation of receptor potential and subsequent generation of the action potential are prevented either by desensitization of the receptor or by the depolarization block of the nerve terminals [28–30]. Desensitization of TRPV1 receptor is dependent on the concentration of the agonist and the extent of calcium influx through the receptor [28,31–33]. Persistent activation of TRPV1 by low concentrations of agonists can cause nerve terminal depolarization by maintaining the sodium channels in an inactivated state, thereby preventing nociceptive transmission in the short term and enhancing calcium influx into the nerve terminal causing nerve terminal ablation in the long term [28,32,34,35]. Therefore, both peripheral and central nerve terminals at the spinal cord can be targeted to induce pain relief by TRPV1 agonists/antagonists [28,32,34]. Intrathecal administration of resiniferatoxin (RTX) reduces inflammatory thermal hypersensitivity without altering acute thermal sensitivity [28,34]. Rats treated with systemic RTX (200 μg/kg, i.p.) were devoid of both nocifensive behavior (manifested as the guarding behavior of the injured paw) and the evoked pain (quantified as thermal hyperalgesia in the hot plate test) [28,34]. Furthermore, it has been found in immunostaining studies that following intrathecal administration of RTX, TRPV1 staining in the central nerve terminals was completely abolished, whereas the staining of the cell bodies in the DRG and the peripheral terminals in the skin were intact [32,36]. Based on these findings, it is proposed that sensory efferent functions that are dependent on CGRP and SP release from the peripheral nerve terminals would not be affected following intrathecal administration of RTX [34–36]. To test the effectiveness of intrathecal administration of RTX in treating cancer pain, a clinical trial has been initiated by National Institute of Dental and Craniofacial Research (NCT008041). TRPV1 agonists and antagonists have been demonstrated to be beneficial in different preclinical models of pain. These include pain associated with multiple forms of arthritis, cancer, chemotherapy, diabetic peripheral neuropathy, Herpes zoster infection, inflammatory bowel disease (IBD), visceral pain, dental pain, and migraine [25,37–48]. Several newer TRPV1 antagonists have been synthesized, characterized, and validated, and some of them have entered clinical trials for these conditions [20,49–51]. Unfortunately, hyperthermia was a major side effect encountered following administration of TRPV1 antagonists in humans [52–54]. This unexpected side effect has halted the clinical trials and has steered away the efforts of the major pharmaceutical companies from pursuing TRPV1 antagonists as analgesics. TRPV1-like immunoreactivity has been observed in myenteric ganglia, intraganglionic fiber tracts, blood vessels supplying GI tract, muscle layers, and mucosa. Eighty percent of sensory fibers that project into the visceral mucosa and 40-60% of vagal afferents arising from nodose ganglia are TRPV1 positive and play a major role in the sensation of bloating/discomfort, pain, nausea, and satiety [55]. TRPV1 labeling has also been found in the parietal cells. Higher density and number of TRPV1-immunoreactive axons have been found in the distal colon and rectum, whereas they are barely expressed in the transverse and proximal colon [56]. Using retrograde labeling techniques, it has been shown that a substantial proportion of spinal and vagal jejuna and colonic afferents express TRPV1 and/or in association with neuronal nitric oxide synthase (NOS), CGRP, and SP [57,58]. TRPV1 mRNA has been detected in cells of the rat gastric wall and rat gastric epithelial cell lines (RGM-1) [59,60]. Presence of TRPV1 on afferents innervating the mucosa of the human esophagus, its increased expression in disease conditions, and activation by acidic pH renders it as a potential target for the treatment of gastroesophageal reflux disease (GERD) [61,62]. TRPV1 knockout mice develop a lesser extent of esophagitis after acid exposure as compared to wild-type controls [63]. Surprisingly, in clinical trials TRPV1 antagonists showed a limited efficacy profile in GERD patients [64] (ClinicalTrials.Gov identifier: D9127C00002). TRPV1-positive neurons mediate visceral pain in response to inflammation and noxious rectal distension [65–67]. TRPV1 inhibition using antagonists and silencing by RNA interference has been reported to ameliorate visceral pain in rats [66,68,69]. Increased TRPV1-immunoreactivity has been observed in colonic sensory afferents in patients with IBD, both Crohn’s disease and ulcerative colitis [70], and in rectal sensory fibers with rectal hypersensitivity and fecal urgency [71]. TRPV1 also appears to be involved in the inflammation and hyperalgesia associated with dextran sodium sulfate-induced experimental colitis [72]. A correlation has been described between the number of TRPV1-immunoreactive fibers in the rectosigmoid colon and the abdominal pain score in patients with irritable bowel syndrome [73]. TRPV1 antagonists prevent the development of visceral hypersensitivity initiated by acetic acid treatment during the neonatal period in a rat model of irritable bowel syndrome [74,75]. TRPV1 modulation by both agonist (capsaicin) and antagonist (YL1421) has shown beneficial effects against trinitrobenzene sulfonic acid-induced rat model of IBD [76,77]. The pancreas is innervated by sensory neurons that play a central role in pancreatitis-associated inflammatory pain [78]. Recent studies suggest that efferent function of primary sensory neurons of the pancreas contributes to inflammation by releasing pro-inflammatory agents that can activate/sensitize TRPV1 [79]. Blockade of TRPV1 by capsazepine significantly attenuated pain associated with experimental pancreatitis, suggesting that the antagonists of TRPV1 could be useful to reduce the inflammatory response and ameliorate the excruciating pain associated with pancreatitis [80]. Activation of TRPV1 mediates neurogenic inflammation in cerulein-induced pancreatitis via activation of the neurokinin receptor 1 [81]. Enhanced TRPV1 immunoreactivity is also observed in the colons of patients with Hirschsprung’s disease, characterized by bowel obstruction [82]. Multiple reports have suggested the TRPV1 expression in neuronal and nonneuronal cells of the urinary system, including bladder, renal pelvis, ureter, and urethra [34,83]. Activation of TRPV1 by agonists and physiological stimuli (heat and low pH) cause calcium influx and ATP release in rat and human urothelial cells [83], suggesting the presence of functional TRPV1. In TRPV1 knockout animals and following chemical/surgical denervation of rat or human bladder leads to loss of TRPV1 function [9,76,84–87]. Recently, Birder and colleagues have shown enhanced TRPV1 expression and TRPV1-dependent ATP release in overactive bladder patients [88]. Intravesical administration of capsaicin or RTX increases bladder capacity and partially restores continence [89,90] in the patients with neurogenic detrusor overactivity disorder. Intravesical administration of RTX in patients with detrusor overactivity has been found to be effective and safe [91–94]. The role of TRPV1 in micturition reflex dysfunction is well established [95–98]. Intravesical administration of TRPV1 agonist (capsaicin and RTX)-induced desensitization or denervation therapy results in controlled micturition in both neurogenic and nonneurogenic cases of overactive bladder [95,99]. TRPV1 antagonists have beneficial effects against pain and inflammation associated with interstitial cystitis [97,100]. GRC-6211, an orally active TRPV1 antagonist, counteracts the bladder hyperactivity and pain induced by cystitis [97]. Intravesicular RTX has been found to be safe, but less tolerable in a prospective, double-blind and randomized clinical trial for the treatment of interstitial cystitis [101]. In mice, genetic manipulation of the TRPV1 gene has been shown to be effective in bladder reflex hyperactivity and the spinal c-fos overexpression in response to cystitis [67]. Interestingly, recent reports have pointed out that severity of cystitis can be positively correlated with expression levels of TRPM2 and TRPV2 but not with TRPV1 [102]. There are several studies using RT-PCR, radioligand binding, in situ hybridization, and autoradiography techniques, suggesting the presence of TRPV1 mRNA and protein in hippocampus (CA1 and CA3 regions); dentate gyrus; thalamic and hypothalamic nuclei; cortical structures such as prefrontal cortex (PFC), somatosensory cortex, anterior cingulate cortex, and insular cortex; limbic structures including central amygdala, caudate putamen, and substantia nigra; locus coeruleus; periaqueductal gray (PAG); ventral tagmental area; cerebellum; nucleus of solitary tract; ventral medulla; and olfactory bulb [103–106]. Interestingly, TRPV1 channels have also been reported to be present on glial cells of the CNS [104,107]. Although, studies have documented the presence of TRPV1 by various methods, there is limited functional evidence by the way of recording TRPV1-mediated membrane currents from CNS neurons. A recent work relying on a powerful combination of reporter mice, in situ hybridization, electrophysiological recordings, and calcium imaging suggests that TRPV1 expression is restricted to very few brain regions, most notably the caudate nucleus of the hypothalamus [108]. Furthermore, a recent study shows convincingly TRPV1 expression in the second-order inhibitory neurons in the spinal dorsal horn [109]. At present, it is unclear how one can reconcile with these strikingly different findings. Clearly, additional research has to be carried out to explain the differences that have been noted between wild-type and TRPV1 knockout mice. TRPV1-mediated modulation in miniature excitatory postsynaptic currents have been observed in the spinal cord, striatum, hippocampus, substantia gelatinosa, PAG, medial preoptic nucleus, substantia nigra, and locus coeruleus. In the CA1 region of the hippocampus, anandamide, an endogenous cannabinoid and a TRPV1 receptor agonist, has been shown to increase paired-pulse depression and inhibit evoked excitatory synaptic transmission that could be reversed by the TRPV1 antagonist, capsazepine but not by cannabinoid receptor 1 (C131) antagonist, AM281 [110,111]. TRPV1 activation facilitates long-term potentiation (LTP) and suppresses long-term depression (LTD) in the hippocampus. Acute stress-induced suppression of LTP and augmentation of LTD are reversed by capsaicin application [112]. Application of capsaicin or 12-(S)-HPETE induces a form of LTD that could be blocked by capsazepine, which is absent in TRPV1 knockout mice [112,113]. TRPV1-mediated calcium influx leads to mitochondrial damage, apoptosis, and cell death in neurons as well as in glial cells via different molecular mechanisms [114–118]. Using a gerbil model of global transient ischemia and ouabain-induced excitotoxicity, researchers have shown that TPRV1 has a neuroprotective effect [106,119–121]. An anandamide uptake inhibitor, AM404, was able to significantly reverse the hyperkinetic movements associated with Huntington’s disease in a 3-nitropropionic acid-induced model with Huntington’s disease. Hyperkinetic movements were reversed by TRPV1 antagonist, capsazepine, suggesting the involvement of TRPV1 [121]. TRPV1 has been implicated in the process of epileptogenesis due to a higher level of expression in the cortex and hippocampus [122,123]. Reduced inhibition within neuronal networks causes hyperexcitability and can enhance seizure susceptibility [124]. During epilepsy, anandamide levels increase due to enhanced neuronal activity [125], and both exogenous and endogenous anandamide display a proconvulsant activity [126]. In contrast, recent reports implicate an anticonvulsant action of anandamide in kainic-acid-induced seizures [123,127]. TRPV1 has been associated with neuropsychiatric disorders and addiction. Higher expression of TRPV1 in PFC, dorsolateral columns of PAG, and ventral hippocampal regions suggests a link between TRPVI and anxiety/aversive (fear) behavior. Ablation of TRPV1 expressing neurons or blockade of TRPV1 receptors can induce anxiolytic behavior. Systemic administration of capsazepine induces anxiolytic-like effects in rats [128]. In addition, TRPV1 knockout mice exhibit reduced anxiety-like behavior and impaired fear conditioning [129]. It has been recently shown that capsazepine induces anxiolytic-like effects when injected into the dorsolateral PAG [130,131], ventral hippocampus [132], and ventral medial PFC [133]. These studies suggest a role of TRPV1 in anxiety and fear conditioning. Studies in dopamine receptor 3 (D3R) knockout mice have suggested that altered endocannabinoid and endovanilloid systems in these animals are responsible for the excitotoxic and anxiogenic effects [134]. Intraperitoneal injections of a TRPV1 agonist, olvanil, decreased the time spent in the open arms of the elevated plus maze [135]. Further, TRPV1 has a role in cocaine-, nicotine-, and ethanol-induced addictive behavior and related psychiatric changes [136,137]. Methamphetamine and morphine administration is associated with increased TRPV1 expression [138,139]. SB366791, a TRPV1 antagonist, prevented morphine-induced tolerance/withdrawal symptoms as well as thermal hyperalgesia [139]. Systemic administration of capsaicin causes a significant reduction in movement in both the horizontal (locomotion) and vertical (rearing) planes, which is reversed by the TRPV1 antagonist, capsazepine [116]. l-Dihydroxyphenylalanine (l-DOPA) treatment in reserpine-treated rats elicits high levels of motor activity in both the horizontal and vertical planes, which is reversed by capsaicin [116]. Furthermore, intranigral administration of 12-(S)-HPETE in rats triggers dopaminergic neuronal death, whereas coadministration of capsazepine prevents this effect [140]. Anandamide and 12-(S)-HPETE have been implicated in l-DOPA-induced dyskinesias and cell death in cultured dopamine neurons by a TRPV1-mediated mechanism [116,140–142]. All these studies suggest a link between TRPV1 and dopaminergic circuits, with a potential role in Parkinson’s disease and dyskinesias. TRPV1 has a role in dopamine-mediated hyperactivity and schizophrenia [119,143]. TRPV1 is involved in the activation of glia (astroglia and microglia). In TRPV1 knockout animals, capsaicin and Complete Freund’s Adjuvant (CFA) administration or sciatic nerve ligation exhibit reduced glial activation, as suggested by reduced ionized calcium-binding adapter molecule 1 (Iba1) and astrocytes glial fibrillary acidic protein immunostaining [39]. The TRPV1 antagonism is associated with a reduction in microglial activation, decrease in the production of proinflammatory cytokines (TNFα, IL-1β, and IL-6), and increase in the production of the anti-inflammatory cytokine IL1-R in different brain regions, including spinal cord and hippocampus [144–146]. Capsazepine and Iodo-RTX can significantly reduce the generation of Reactive Oxygen Species (ROS) in microglia [147]. TRPV1 immunoreactivity is present in the lungs (in sensory nerve fibers), extra and intrapulmonary airways (within and beneath the epithelium, around blood vessels, and within airway smooth muscles and alveoli), tracheal epithelium, mast cells, pleura, and nasal mucosa [148–152]. Capsaicin induces calcium influx, causes membrane depolarization, and generates action potentials, mucus secretion and cough reflex through activation of TRPV1 [153,154]. Asthma, obstructive sleep apnea [155], chronic cough [156,157], cystic fibrosis [158], airway hyper-responsiveness [159], and acute lung injury [160] are all associated with acidic pH of the lower airway. Impaired CO2 clearance from the lungs in chronic obstructive pulmonary disease or excessive lactic acid production caused by tissue ischemia or hypoxia can lead to the acidification of pulmonary tissues. TRPV1 is activated by low pH, which suggests its involvement in airway-related diseases. Activation of TRPV1 induces the release of neuropeptides [161–163]. Stimulation of vagal pulmonary sensory neurons by bradykinin (BK) can initiate cough reflex [164]. TRPV1 is expressed in sensory airway nerve fibers and plays an important role in the cough reflex [153,165–167]. BK- and proton-induced activation or sensitization of TRPV1 underlies this response. One of the common side effects of angiotensin-converting enzyme inhibitors during the treatment of hypertension is cough, which is due to accumulation of BK because of the inhibition of its degradation. TRPV1 antagonists (capsazepine, iodo-RTX. BCTC, and JNJ17203212) have been shown to block capsaicin, citric acid-, and antigen-induced cough responses [168–171]. Capsaicin-induced desensitization of TRPV1 inhibits allergenic bronchoconstriction in sensitized guinea pigs [172]. Capsaicin-induced bronchoconstriction is not significant in humans, possibly due to lack of specific innervation [173–175]. Activation of TRPV1 may be associated with airway inflammation in chronic respiratory diseases [176,177]. Expression of TRPV1 in rat DRG neurons projecting to the lung and pleura has been investigated [178]. TRPV1 is coexpressed with CGRP and SP in the secretory cells of the airway and lungs. TRPV1-induced calcium influx can also trigger the release of SP and CGRP from both peripheral and central nerve terminals of sensory neurons resulting in the development of “neurogenic inflammatory reaction” in the airways [179,180]. Airway neurogenic inflammation is associated with the pathogenesis of rhinosinusitis [181,182]. Intranasal administration of capsaicin induces desensitization of TRPV1 and provides relief in patients with vasomotor rhinitis [183]. TRPV1 is also reported to be involved in endoplasmic reticulum-mediated stress and cell death in human bronchial epithelial and alveolar cells [184]. Endogenous 13-S-hydroxyoctadecadienoic acid (13-S-HODE) produced in high concentrations during mitochondrial degradation in reticulocytes plays a role in asthma. Inhibition of TRPV1 attenuates 13-S-HODE produced in both a mouse model and human bronchial epithelial cells [185]. Mucus hypersecretion occurs in chronic obstructive pulmonary disease, and TRPV1 plays a role in mucus secretion [177,186,187]. Tumorigenesis is associated with altered expression of TRP channels (TRPV1, TRPV6, TRPC1, TRPC6, TRPM1, TRPM4, TRPM5, and TRPM8) [188]. There are two strategies of targeting TRPV1 channels for the development of drugs to treat cancer. First, activation of TRPV1 can lead to sustained increase in cytoplasmic concentrations of calcium resulting in cancerous cell death either by apoptosis or necrosis [189,190]. Second, in TRPV1 expressing cancerous cells, TRPV1 ligands can act as carriers for the toxic payload (in radiotherapy or chemotherapy), thereby enhancing the efficacy and localization of drugs [191]. Several types of cancers such as prostate, bladder, brain, mammary gland, pancreas, tongue, skin, liver, and colon show altered expression of TRPV1 in the affected tissue. Overexpression of TRPV1 and TRPV1-mediated CGRP release have been demonstrated in colon adenocarcinoma by immunohistochemical studies. TRPV1 expression has also been reported in Glioblastoma multiforme, a deadly form of brain cancer [192]. TRPV1 receptor activation mediates cell death, thereby inhibiting the development of brain carcinoma [193]. Some studies have suggested that TRPV1 receptor is expressed and functional in human prostate cells as well as in prostate cancer cell lines [194]. Capsaicin, methanandamide, and RTX induce a dose-dependent increase in the intracellular calcium concentration in prostate cells, which is reversed by capsazepine [195]. There is a significant decrease in TRPV1 expression in human urothelial cancer cells [118,196]. Intrinsic and extrinsic apoptotic pathways are triggered in epithelial cancer cells by the application of capsaicin [118]. Expression of TRPV1 is significantly upregulated in human pancreatic cancer cells [189]. A recent study has indicated that TRPV1 receptor expression has a role in the prediction of hepatocellular carcinoma [197]. Capsaicin-stimulated calcium influx and cell migration are enhanced by hepatocyte growth factor/scatter factor (HGF/SF). Thus, TRPV1 may mediate, in part, the action of HGF/SF in increasing intracellular calcium and promoting tumor invasion in hepatoblastoma. TRPV1 gene expression is also identified in uveal melanoma cells [198] and oral squamous cell carcinoma [198–200]. Recently published studies demonstrate the presence of TRPV1 in human and canine mammary cancer cells [201]. TRPV1-immunoreactivity has been shown to be increased in all layers of the tongue epithelium in patients with squamous cell carcinoma of the tongue [199]. The incidence of skin cancer is increased significantly in mice lacking TRPV1 [202]. TRPV1 has been proven to be important in modulating cardiovascular functions. TRPV1 is expressed in sensory neurons innervating cardiac tissue, especially spinal sympathetic afferent fibers with nerve endings in the heart [203]. These afferent nerve endings may transduce tissue hypoxia- and inflammation-induced cardiac pain. Multiple studies have demonstrated the importance of the activation of TRPV1 and subsequent release of neuropeptides (CGRP, SP, and BK) and neurotransmitters (NE and ACh) in cardioprotection [204–208]. Blood vessels are densely innervated by TRPV1 expressing C-fiber terminals [209,210]. TRPV1-mediated CGRP (a potent vasodilator) release plays an important role in maintaining the microvascular circulation. CGRP is colocalized with SP in the sensory ganglia and perivascular nerve fibers of the heart [180,211]. CGRP is released by TRPV1 activation and reduces vascular resistance and coronary perfusion pressure [212,213]. The endogenous cannabinoid, anandamide, induces profound vasodilation by direct activation of TRPV1 and subsequent release of CGRP [214]. Involvement of neurogenic release of CGRP in ischemia/reperfusion is altered in TRPV1 knockout and capsazepine-treated animals suggesting a role of TRPV1 [215]. Capsaicin-sensitive nerve terminals also express NOS and produce NO, which can affect vascular tone [49,216]. Increased spontaneous firing activity of sensory neurons innervating the heart following coronary artery occlusion is significantly decreased by the treatment with iodo-RTX [217]. TRPV1-mediated sympathetic responsiveness is enhanced in rats with the femoral artery occlusion as compared with sham-operated animals [218]. Ischemia-induced firing of nerve fibers carrying cardiac ischemic pain may be because of the activation of TRPV1 by acidosis. Loss of TRPV1 expressing nerve fibers in diabetic neuropathy may be responsible for silent myocardial ischemia in patients with long-standing diabetes. One of the areas where TRPV1 is expressed other than its predominant expression in sensory neurons is vascular smooth muscle cells [219]. Altered TRPV1 activity contributes to the development of hypertension and plays a counterbalancing role in preventing salt-induced increases in blood pressure. Data collected from genetic and experimental models of hypertension suggest that the destruction of capsaicin-sensitive sensory neurons renders a rat salt sensitive and hampers blood pressure regulation [220]. TRPV1 dysfunction is implicated in renal hypertension [221]. Ablation of the TRPV1 gene exacerbates renal damage induced by deoxycorticosterone (DOCA)-mediated salt hypertension, indicating that TRPV1 may play a protective role against end-organ damage induced by hypertension [222]. These renoprotective effects seem to be closely related to the inhibition of inflammatory response mediated via TRPV1. Involvement of protein kinase C pathway has been suggested [222]. Renal inflammation (monocyte/macrophage and lymphocyte recruitment, proinflammatory cytokine and chemokine production, nuclear factor-kappa B (NF-κB) activity, and adhesion molecule expression) is aggravated in DOCA-salt hypertensive TRPV1 knockout mice, suggesting that TRPV1 antagonists may lessen renal injury in DOCA-salt hypertensive animals [223]. TRPV1 expression and function are upregulated during high salt intake in Dahl salt-resistant (DR) rats, which prevent salt-induced increases in blood pressure, whereas the expression and function of TRPV1 are impaired in Dahl salt-sensitive (DS) rats [123]. TRPV1 function is impaired in the kidneys of DS rats, altering renal hemodynamics, and contributes to hypertension [123]. Activation of TRPV1 expressed in sensory nerves innervating the renal pelvis enhances afferent renal nerve activity and diuresis [224]. TRPV1 agonists capsaicin, RTX, and an orally active agonist, SA13353, prevent the ischemia/reperfusion-induced acute kidney injury, and the effect is proposed to be associated with the inhibition of inflammatory response mediated by TRPV1 [225,226]. Furthermore, TRPV1 knockout mice exhibit reduced serum arginine-vasopression response to increased serum osmolarity following high salt intake [227]. TRPV1 has a potential role to play in diabetes- and obesity-related cardiac complications. TRPV1 activation by capsaicin protects against diabetes-induced endothelial dysfunction through a mechanism involving the PKA/UCP2 pathway [228]. Obesity-related release of endothelium-derived contracting factors is also mediated by TRPV1 activation [229]. 17β-Estradiol potentiates mesenteric relaxation in response to anandamide through a TRPV1-dependent mechanism [230]. Reports confirm the expression of TRPV1 in duramater, which is associated with migraine headaches [231,232]. Coexpression of CGRP with TRPV1-expressing neurons and the requirement of TRPV1 activation for its release suggests that TRPV1 plays an important role in migraine [49,233–236]. Migraine-associated symptoms like phonophobia, photophobia, and facial allodynia may be associated with sensitization of TRPV1 expressed in TG neurons [237]. Multiple TRP channels including TRPV1 have shown to be expressed in pancreatic β-cells and play a significant role in insulin secretion and glucose homeostasis [238,239]. TRPV1 is reported to be expressed in nerve fibers supplying the pancreas, pancreatic islet endocrine cells, and pancreatic beta cell lines (RIN and INS1) [79,240,241]. Activation of TRPV1 expressed in islet beta cells increases intracellular calcium and facilitates insulin secretion [240], and the effect is inhibited by capsazepine or by removal of extracellular calcium [240]. TRPV1-mediated release of insulin can sensitize TRPV1 [242,243]. Activation of TRPV1 at nerve terminals can release neuropeptides such as CGRP and SP. SP promotes neurogenic inflammation, thereby creating an autocrine feedback loop involving TRPV1 [79]. Involvement of TRPV1 expressed at sensory neurons in diabetes, glucose homeostasis, and insulin sensitivity has been investigated by using different animal models [79,244–248]. Role of TRPV1 has been studied in obesity and other metabolic disorders. Consumption of chili peppers and related dietary ingredients generate heat sensation and increase the energy expenditure [249–251]. There are multiple clinical trials, suggesting the beneficial antiobesity effects of active ingredients in chili peppers, capsaicin and capsinoids (nonpungent capsaicin analogues), by virtue of their action on fat oxidation, energy expenditure, and induction thermogenesis [45,250–253]. In addition, experimental evidences through animal studies suggest that capsaicinoids (capsaicin, dihydrocapsaicin) possess antiobesity properties [45,250,254]. In a clinical trial, a Japanese group has found that capsinoid ingestion increases energy expenditure through brown adipose tissue activation [255]. Although it is controversial, multiple groups have shown the presence of TRPV1 channels in 3T3-L1 preadipocytes and adipose tissue of mice (wild type and ob/ob) and in fat tissue of obese humans [33,256]. Capsaicin dose-dependently induced calcium influx and prevented adipogenesis in 3T3-L1 preadipocytes [33]. Long-term capsaicin consumption along with the high-fat diet prevented diet-induced obesity in WT mice, but not in TRPV1-null mice [33]. Capsaicin (0.014% in the diet) did not influence the caloric intake but significantly decreased the weight of the visceral fat-pad in rodents [257]. Researchers have used genomic and proteomic tools to understand the molecular mechanisms of the antiobesity effect of capsaicin by analyzing the gene and protein expression levels in adipose tissue of rats in response to capsaicin treatment [33,36,258]. Using TRPV1 knockout animals, the role of TRPV1 in adipose tissue inflammation and sensory neuronal dysfunction has been studied [259]. TRPV1 activation by capsaicin or its nonpungent analogs capsiates has been reported to have modulatory effects on the cellular signaling pathways involved in energy metabolism, preadipocyte differentiation and obesity-induced inflammation. Capsaicin can cause activation of the serine/threonine kinase, AMP-activated kinase and peroxisome proliferator-activated receptor gamma inactivation of NF-κB, up-regulation of uncoupling protein (UCP) 1, and UCP2 expression in brown and white adipose tissues. Further, capsaicin also causes activation of caspase-3, pro-apoptotic genes Bax and Bak, cleavage of poly (ADP-ribose) polymerase (PARP), and down-regulation of antiapoptotic factor Bcl-2, hence resultant promotion of apoptosis [33,242,243,258,260]. CGRP, which has a role in antagonizing insulin release, is primarily secreted by TRPV1-expressing nerve terminals. Capsaicin administration to young rats destroyed capsaicin-sensitive afferent autonomic nerves following prolonged sensitization and activation, which resulted in persistently high-circulating CGRP levels. High CGRP levels cause insulin resistance and result in obesity in the long term [213,261,262]. TRPV1 channels are functionally expressed in the coronary vessels of lean and obese male Ossabaw miniature swines and mediate endothelium-dependent vasodilatation [263]. Endogenous TRPV1 ligand, N-oleoylethanolamide reduces food intake in wild-type, but not in TRPV1 knockout mice, which suggests a novel role for TRPV1 in appetite regulation [264,265]. Although several reports suggest that capsaicin has antiobesity effects, because of its pungency, it has limited use. The nonpungent CH-19 sweet pepper (the major source of natural capsinoids), therefore, might be an attractive alternative. Capsiate, dihydrocapsiate, and nordihydrocapsiate present in capsinoids in CH-19 sweet pepper are relatively less pungent than capsaicin or dihydrocapsaicin [266]. Increased body temperature and oxygen consumption was noticed with a single dose of CH-19 sweet pepper, whereas regular consumption reduced body weight and promoted fat oxidation [267,268]. Capsiate, similar to capsaicin, enhances the energy expenditure and raises the core body temperature [269,270]. Capsinoids increased fat oxidation and thermogenesis in wild-type mice, but not in TRPV1 knockout mice, suggesting the involvement of TRPV1 in this action [271]. Capsinoids also increase the levels of UCP in adipose tissue [260]. Capsiate is available as a weight loss product in the market in Japan and the United States [266]. There are reports contradicting the role of TRPV1 in obesity. In several studies, TRPV1 knockout mice showed decreased body fat [262]; therefore, the exact role of TRPV1 in the regulation of body weight remains to be explored. Immune cells express TRPV1, and capsaicin modulates various immune responses, but the mechanism is still unclear. TRPV1 is shown to have beneficial effects on several immune diseases, including autoimmune encephalomyelitis, chronic fatigue syndrome, autoimmune diabetes, fibromyalgia syndrome, liver injury [272–275], and dermatitis [276]. TRPV1 has been shown to be involved in the progression of allergic contact dermatitis (ACD), as shown by its protective effect against oxazolone or 2,4-dinitrofluorobenzene-induced murine ACD [276,277]. Recent studies have shown that TRPV1 has a significant role in atopic dermatitis [278]. In an animal model of atopic dermatitis, increased coexpression of gastrin releasing peptide with TRPV1 was detected [279]. Capsaicin-induced desensitization of TRPV1 relieves scratching behavior in different animal models of atopic dermatitis [280]. TRPV1 antagonist, PAC-14028 ameliorates the atopic dermatitis-like symptoms in the NC/nga mice [281]. Interestingly, in another model of atopic dermatitis (NC/Tnd mice), stimulation of TRPV1 was found to be beneficial [282]. TRPV1 agonists have been suggested as novel adjuvants in contact hypersensitivity, as suggested by the favorable outcomes in fluorescein isothiocyanate-induced mouse contact hypersensitivity model [283]. TRPV1 neurons respond to different itch-producing agents and can be classified as a central integrator of the itch mechanism, although itch sensitivity is carried by a specific population of neurons at the level of the spinal cord [128,284]. TRPV1 is considered as a candidate to explain the amiloride-insensitive salty taste because TRPV1 knockout mice showed diminished gustatory nerve responses [285]. Immunohistochemical studies reveal the expression of TRPV1 in the hair cells, cells of the organ of Corti, and spiral ganglion cells of the cochlea [286]. TRPV1 may be involved in the regulation of human hair growth and hearing. It has been shown that TRPV1 agonists can inhibit hair growth. TRPV1 knockout mice exhibit a significant delay in hair follicle cycling as compared to wild-type counterparts [287]. Capsaicin markedly suppresses hair shaft elongation, induces apoptosis and catagen regression, and promotes the intrafollicular cytokine production in organ-cultured human hair follicles [288]. In addition, after noise exposure TRPV1 expression levels increase in all cochlear regions [289]. A recent report has suggested that a TRPV1-mediated inflammatory process is central to temporary hearing loss [290]. Also, cisplatin-induced hearing loss could be reduced by short interfering RNA against TRPV1 [291]. TRPV1 is also expressed in human sebaceous glands and in the immortalized SZ95 sebocyte cell line [292]. In summary, TRPV1 plays a significant role in many disease processes. The preclinical research from academia and industries has provided proof of concept that targeting TRPV1 by agonists or antagonist may be a useful strategy in treating some of the disease conditions. Contradictory data from clinical studies are discouraging, given the side effects associated with agonists (pungency) and antagonists (hyperthermia). However, localized effect by topical application or slow intrathecal administration can be useful to target peripheral and central terminals for pain relief. Further, oral administration of these agents could produce local effects in the GI tract by acting on the nerve terminals or nonneuronal cells (enteroendocrine cells) by releasing peptide hormones in a paracrine and endocrine fashion. The controversy over the extent of TRPV1 expression and distribution in different tissues stands in the way of making significant progress. Although the expression of TRPV1 is confirmed in certain tissues, the required concentrations of exogenous and endogenous ligands to activate the receptor leads to speculation about the role of TRPV1 in several physiological and pathophysiological conditions.
The Role of TRPV1 in Acquired Diseases
Therapeutic Potential of TRPV1 Modulators
2 Department of Food Sciences and Technology, National Agri-Food Biotechnology Institute (NABI), SAS Nagar, India
3 Department of Pharmacology, Southern Illinois University-School of Medicine, Springfield, Illinois, USA
* Corresponding author: lpremkumar@siumed.edu
Abstract
Acknowledgment
Introduction
TRPV1 and Pain
TRPV1 and Gastrointestinal System-Related Disease
TRPV1 and Urinary System-Related Diseases
TRPV1 and Central Nervous System-Related Diseases
TRPV1 and Respiratory System-Related Diseases
TRPV1 and Cancer
TRPV1 and Cardiovascular System-Related Diseases
TRPV1 and Metabolic Diseases
TRPV1 and Other Disease Conditions
Conclusions
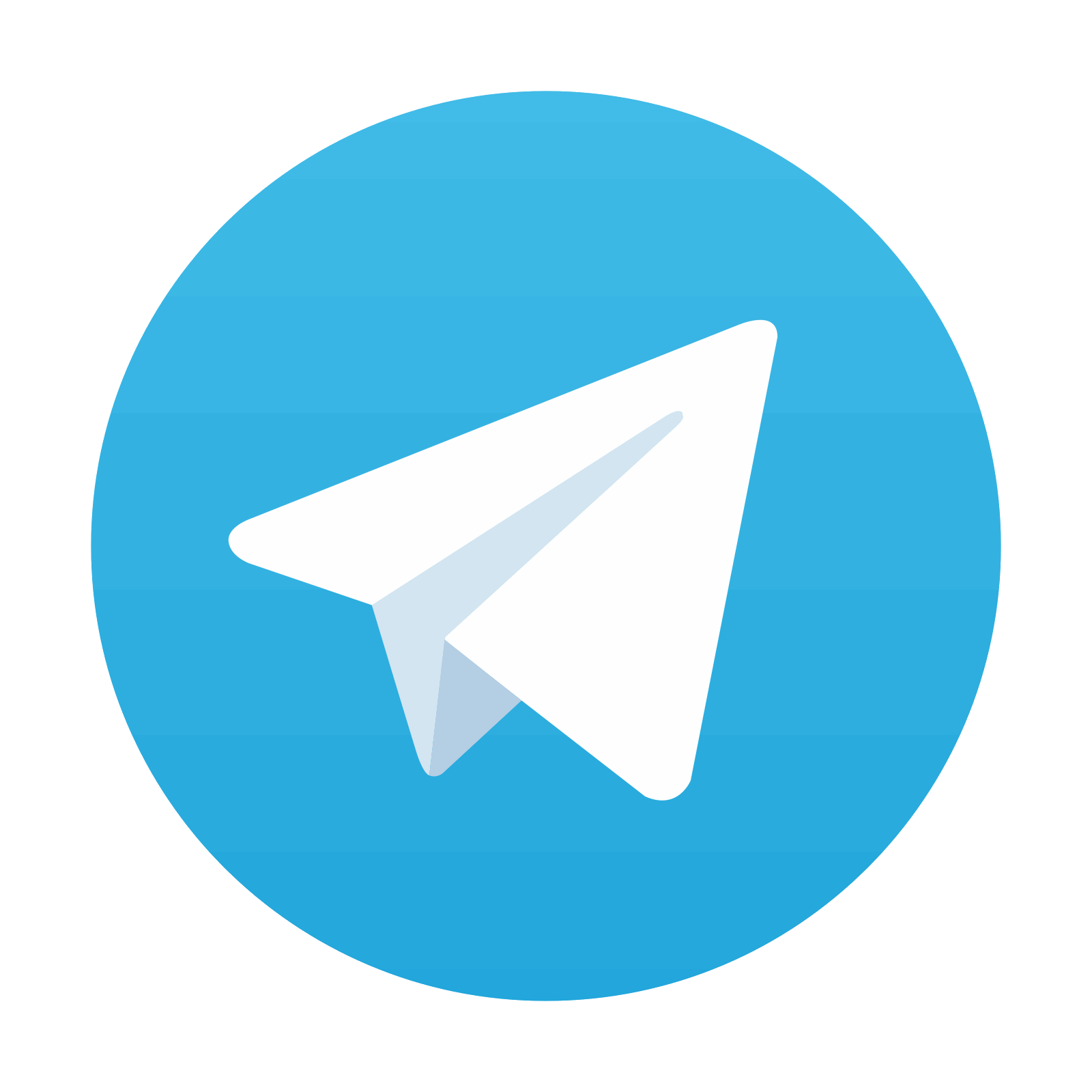
Stay updated, free articles. Join our Telegram channel

Full access? Get Clinical Tree
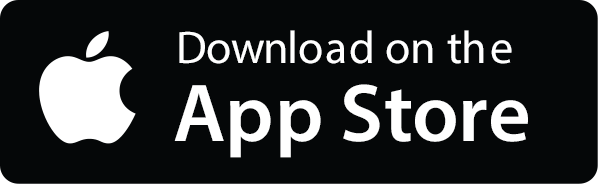
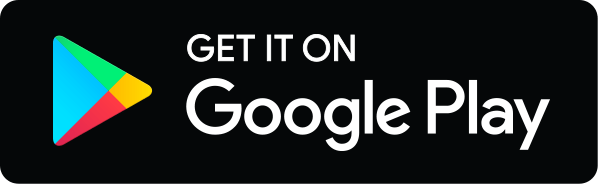