Chapter 22 Artem Kondratskyi1; Natalia Prevarskaya1,* 1 Inserm U-1003, Equipe labellisée par la Ligue Nationale contre le cancer, Laboratory of Excellence Ion Channels Science and Therapeutics, Université Lille 1, Villeneuve d’Ascq, France Transient receptor potential (TRP) channels play an important role in many fundamental cellular processes and are also implicated in initiation and progression of different cancers. To date, most reports suggest that malignant transformation is often associated with changes in TRP channels expression/function rather than mutations. Current evidence supports that TRP channels are important in the regulation of the cellular processes altered in cancer, including proliferation, differentiation, apoptosis, migration, and angiogenesis. However, the contribution of a specific TRP channel to these processes could vary depending on cancer type and stage. Therefore, further studies are needed to unravel specific roles of TRP channels in each type of cancer to determine their potential to be used as diagnostic markers and/or therapeutic targets in each particular case. Cancer is a group of diseases characterized by out-of-control growth and dissemination of abnormal cells. Cancer represents one of the leading causes of death worldwide, accounting for 8.2 million deaths in 2012 [1]. Both external (radiation, tobacco, car exhaust fumes, and other carcinogens) and internal factors (inherited mutations, hormones, immune conditions) could be the causes of cancer. Moreover, these factors may act together or in sequence to initiate or promote the development of cancer [2]. There are more than 100 different types of cancer, including breast cancer, skin cancer, lung cancer, colon cancer, prostate cancer, lymphoma, and others. Different cancers are characterized by the different set of mutated genes (either oncogenes or tumor suppressor genes) and thus behave differently. However, Hanahan and Weinberg have proposed several general hallmarks, characteristic to most forms of cancer [3]. These hallmarks include sustaining proliferative signaling, evading growth suppressors, resisting cell death, enabling replicative immortality, inducing angiogenesis, activating invasion and metastasis, avoiding immune destruction, deregulating cellular energetics, tumor-promoting inflammation as well as genome instability and mutation [3]. Interestingly, calcium signaling has been previously reported to regulate most if not all of these phenomena [4,5]. Changes in the cytosolic-free Ca2 + concentration play a central role in many fundamental cellular processes, including muscle contraction, transmitter release, cell proliferation, differentiation, gene transcription, and cell death [6]. Given that Ca2 + controls so many vital processes, disturbance of the Ca2 + homeostasis regulatory mechanisms leads to a vast variety of severe pathologies, including cancer. Indeed, the role of Ca2 + is well established in many cell signaling pathways involved in carcinogenesis [7–9]. The increase in cytosolic calcium can occur as a result of Ca2 + influx from the extracellular space and/or Ca2 + release from intracellular sources. Both Ca2 + influx and Ca2 + release are tightly controlled by numerous regulatory systems that provide the specific spatial and temporal characteristics of an intracellular calcium signal that are required for sustaining certain cellular functions [6]. Mitochondrial, endoplasmic reticulum (ER), lysosomal, and cytosolic calcium levels are regulated by calcium-permeable ion channels localized either on the membranes of the intracellular organelles or on the plasma membrane [10,11]. The calcium-permeable channels, including families of transient receptor potential (TRP) channels, store-operated channels, voltage-gated calcium channels, two-pore channels, mitochondrial permeability transition pore, mitochondrial calcium uniporter, inositol 1,4,5-trisphosphate receptor (IP3) and ryanodine receptors, and others contribute to changes in [Ca2 +]i by providing Ca2 + entry pathways, by modulating the driving force for the Ca2 + entry, and also by providing intracellular pathways for Ca2 + uptake/release into/from cellular organelles [10–13]. Thus, modulation of a calcium-permeable ion channel’s expression/function affects intracellular Ca2 + concentrations and consequently calcium-dependent processes, such as proliferation, apoptosis, and autophagy [14–16]. The growing number of studies suggests that malignant transformation is often accompanied by the changes in ion channel expression/function [17]. Among these channels, the members of the TRP channels superfamily have been shown to regulate a variety of calcium-dependent cellular processes altered in cancer [18]. In this chapter, we will summarize the information available on the role of TRP channels in different cancers and discuss the possible use of these channels as biomarkers and therapeutic targets to treat cancer. Among 28 members of the mammalian TRP superfamily grouped into six subfamilies (TRPC (canonical), TRPM (melastatin), TRPV (valinoid), TRPA (ankyrin-like), TRPP (polycystin), and TRPML (mucolipin), over 10 members were reported to have a role in cancer [18]. Further, we discuss specific roles of various TRP channels in different cancers, categorized by TRP subfamily. TRPM1 (melastatin), the founding member of TRPM subfamily, was originally identified as a melanoma metastasis suppressor gene based on its decreased expression in metastatic mouse melanoma cell lines [19]. TRPM1 is involved in the regulation of calcium homeostasis in melanocytes and mediate an endogenous current that can be suppressed by TRPM1 knockdown [20,21]. Moreover, it was shown that ultraviolet B radiation could regulate the expression of TRPM1 [20]. Also, TRPM1 expression has been linked to melanocyte proliferation and differentiation in vitro. TRPM1 expression is significantly lower in rapidly proliferating melanocytes compared to the differentiated melanocytes [20]. TRPM1 is alternatively spliced, resulting in the production of long and short isoforms [21–23]. Full-length TRPM1 (1533 amino acids) localizes to the plasma membrane and mediates Ca2 + entry when expressed in human embryonic kidney cells, whereas a cytosolic N-terminal truncation variant (500 amino acids) interacts directly with and suppresses the activity of full-length TRPM1. This suppression seems to result from the inhibition of translocation of TRPM1 to the plasma membrane [24]. Thus, alternative splicing of TRPM1 can potentially affect melanoma progression. It was reported that TRPM1 is expressed at high levels in poorly metastatic variants of B16-F1 melanoma cell line, and its expression was much less in highly metastatic B16-F10 melanoma cell line [19]. Moreover, Duncan and colleagues demonstrated high TRPM1 levels in normal human epidermal melanocytes and benign melanocytic nevi, whereas in primary melanomas TRPM1 expression was decreased with virtually no expression detected in metastatic melanoma [19]. Further, TRPM1 mRNA expression appears to inversely correlate with melanocytic tumor progression, melanoma tumor thickness, and the potential for melanoma metastasis [22,25,26]. These patterns of TRPM1 expression have led to the suggestion that TRPM1 could serve as a valuable diagnostic and prognostic marker for the development and progression of melanomas [27]. TRPM2 is a chanzyme, that is, an ion channel with enzyme activity. Its structure contains an enzymatic region with ADP-ribose (ADPR)-hydrolase activity [28]. TRPM2 is widely expressed in mammalian cell types, including neurons [29], immune cells [30], insulin-secreting cells [31], and neutrophil granulocytes [32]. It is thought that the principal physiological role of TRPM2 is to control cytokine release in human monocytes, including tumor necrosis factor-alpha (TNFα) [33], insulin secretion [31], and others. TRPM2 is activated by a number of signals, such as intracellular ADPR, hydrogen peroxide, and TNFα [34–36]. One of the most remarkable features of TRPM2 is its modulation by oxidative stress [34]. Recently it has been shown that TRPM2 could play a role in different cancers. It has been reported that insertion of TRPM2 into human glioblastoma cells facilitates hydrogen peroxide-induced cell death with no effect on proliferation, migration, and invasion [37]. In melanoma cell lines, TRPM2 locus has been found to transcribe TRPM2-AS (antisense) and TRPM2-TE (tumor-enriched dominant negative) transcripts, in addition to full-length TRPM2 [38]. Both TRPM2-AS and TRPM2-TE transcripts are up-regulated in melanoma and several other cancers, and thus, they down-regulate full-length TRPM2. Functional knockout of TRPM2-TE or overexpression of wild-type TRPM2 increases melanoma susceptibility to apoptosis and necrosis. Thus, restoration of full-length TRPM2 activity in cancer could be an attractive therapeutic opportunity. Interestingly, a somewhat different role of TRPM2 was demonstrated in prostate cancer [39]. siRNA-mediated knockdown of TRPM2 inhibits the growth of prostate cancer cells but not of noncancerous cells. This is due to the difference in its subcellular localization. In noncancerous cells TRPM2 is homogenously located near the plasma membrane and in the cytoplasm, whereas in the cancerous cells, a significant amount of the TRPM2 protein is located in the nuclei. Thus, it was suggested that TRPM2 is essential for prostate cancer cell proliferation and may be a potential target for the selective treatment of prostate cancer [39]. TRPM4 and TRPM5 are closely related channels, which are impermeable for calcium, in contrast to all other TRPs [40,41]. TRPM4 is mostly expressed in the pancreas, heart, and placenta, whereas TRPM5 is detected in the lungs, testis, tongue, digestive system, and brain [42]. It was reported that TRPM4 levels are elevated in prostate cancer, diffuse large B-cell non-Hodgkin lymphoma, and cervical cancer [43–45]. Further, it was demonstrated that TRPM4 enhances cell proliferation of HeLa cells, a cervical cancer-derived cell line, through up-regulation of the β-catenin signaling pathway [46]. In a recent study on the role of genetic polymorphisms in immune response genes in the development of childhood leukemia, Han and coauthors suggested that some genetic variants of TRPM5 are associated with the risk of childhood leukemia [47]. It is worthwhile to note that the Trpm5 gene is located on chromosome 11, aberrations of which have been linked to a variety of malignances including leukemia, ovarian cancer, and rhabdoid tumors [48–50]. Moreover, TRPM5 mRNA was found to be expressed in a large proportion of Wilms’ tumors and rhabdomyosarcomas [49]. TRPM7 represents a Ca2 +-permeable nonselective cation channel with enzyme activity. It contains an atypical serine/threonine protein kinase within the C-terminal domain [51]. It is ubiquitously expressed and involved in the regulation of cellular magnesium homeostasis [52,53]. TRPM7 has been shown to regulate lymphocyte survival [54], anoxic neuronal cell death [55], cell volume in human epithelial cells [56], cell adhesion in neuroblastoma [57], as well as osteoblast proliferation and migration [58]. In cancer, TRPM7 has been reported to be overexpressed in high-grade breast cancer samples. Moreover, its expression positively correlates with tumor size [59], which makes it the putative diagnostic/prognostic marker for the development and progression of breast cancer. Silencing of TRPM7 was shown to inhibit growth and proliferation of human head and neck squamous carcinoma cell lines [60], human retinoblastoma cells [61], as well as breast cancer cells MCF-7 [59]. Recently it was reported that high levels of TRPM7 expression independently predict poor outcome in breast cancer patients and that it is functionally required for metastasis formation in a mouse xenograft model of human breast cancer [62]. Mechanistic studies revealed that TRPM7 regulates myosin II-based cellular tension, thereby modifying focal adhesion number, cell-cell adhesion, and polarized cell movement [62]. Another group of scientists suggested that TRPM7 mediates breast cancer cell migration and invasion through the mitogen-activated protein kinase (MAPK) pathway [63]. The importance of TRPM7 for human nasopharyngeal carcinoma cells migration [64], as well as for proliferation, migration, and invasion of pancreatic adenocarcinoma cells has also been reported [65]. TRPM8, another member of TRPM subfamily, was first cloned from the human prostate as a prostate-specific gene up-regulated in cancer [66]. Later, it was shown that TRPM8 has a major role in the cold sensation in sensory neurons [67,68]. In normal tissues, the expression of the channel could be detected in a subpopulation of primary sensory neurons [67,68], as well as in the male reproductive system [66,69–71]. In situ hybridization analysis showed that TRPM8 mRNA expression was at moderate levels in normal prostate tissue and appears to be elevated in prostate cancer [66]. Other than prostate, TRPM8 mRNA was expressed in a number of primary tumors of breast, colon, lung, and skin origin, whereas transcripts encoding TRPM8 were hardly detected or not detected in the corresponding normal human tissues [66]. Moreover, a significant difference in the expression level of TRPM8 mRNA between malignant and nonmalignant tissue specimens has been detected in prostate cancer tumors [70]. It was proposed that in prostate cancer cells TRPM8 could form a calcium-permeable channel at both plasma membrane and ER [72–75]. TRPM8 is subjected to alternative splicing, which generates several splice variants of the full-length and truncated forms. The full-length isoform is expressed at the plasma membrane, whereas the short isoform is localized to ER, where it forms a Ca2 +-release channel [72,74,75]. TRPM8 localization and activity was reported to be regulated depending on the differentiation and oncogenic status of prostate cancer cells [72,75]. Thus, only highly differentiated prostate cancer cells expressed functional plasmalemmal TRPM8 channels. This was further confirmed by the finding that the TRPM8-mediated current density was significantly higher in prostate cancer cells obtained from in situ prostate cancer biopsies than in normal prostate or benign prostate hyperplasia cells. However, following dedifferentiation and loss of the luminal secretory phenotype, activity of plasmalemmal TRPM8 was abolished. In contrast, the ER-TRPM8 remained functional regardless of the differentiation status of prostate cells [72]. This differential regulation of TRPM8 activity has been explained by the complex regulation of the TRPM8 isoforms by the androgen receptor [73,75]. Indeed, it has been suggested that TRPM8 is directly regulated by androgen receptor in both normal and cancer prostate cells [73,75]. It appears that Trpm8 gene expression requires a functional androgen receptor and is down-regulated in cells losing androgen sensitivity [73,76]. Moreover, overexpression of androgen receptor in PNT1A cells (human postpubertal prostate normal, immortalized with SV40), that normally lack it, induced TRPM8 expression [73]. TRPM8 overexpression and overactivity in androgen-dependent prostate cancer was reported to correlate with the increased cell growth [77]. On the other hand, antiandrogen therapy as well as transition to androgen independence is associated with the loss of TRPM8 in prostate cancer cells, suggesting that the loss of TRPM8 could be a potential marker of the transition to a more advanced form of the prostate cancer [76]. Furthermore, it has been shown that pharmacological activation of TRPM8 as well as silencing of the channel with siRNA in human prostate carcinoma LNCaP cells negatively influences cell survival, likely by perturbing Ca2 + homeostasis [75]. However, plasma membrane TRPM8 might have a protective effect because stimulation of TRPM8 activity by PSA (prostate-specific antigen) reduced prostate cancer cell motility [78]. Therefore, the gradual loss of the plasmalemmal TRPM8 during tumor progression to late, invasive stages may be an adaptive mechanism of prostate cancer epithelial cells to reduce constant stimulation of the PSA/TRPM8 pathway [78]. Along with prostate cancer, the role of TRPM8 has been also demonstrated in other cancers. Activation of TRPM8 with menthol was reported to decrease the viability of melanoma cells [79]. In breast cancer, TRPM8 is functional at the plasma membrane and expressed in early primitive breast cancers presenting a well-differentiated status [80]. Further, TRPM8 was shown to be overexpressed and required for proliferation of pancreatic adenocarcinoma cells [65]. In human bladder cancer cells, menthol induces cell death via the TRPM8 channel [81]. Thus, TRPM8 seems to have a great potential to be used as a diagnostic marker and/or therapeutic target in the treatment of different cancers. The TRPV1 channel, also known as capsaicin receptor, was the first discovered TRPV channel. TRPV1 was originally identified in sensory neurons as a heat-activated ion channel, which functions as a transducer of painful thermal stimuli in vivo [82]. Increased expression of TRPV1 has been found in different cancers, including cancers of prostate, colon, pancreas, and bladder [83–86]. High expression of TRPV1 was reported to be associated with a better prognosis of patients with hepatocellular carcinoma [87]. A progressive loss of TRPV1 expression has been found in the urothelium, as transitional cell carcinoma stage increased and cell differentiation was lower [85]. In glioma, TRPV1 expression was reported to inversely correlate with tumor grade with a complete loss observed in 93% of high grade (IV) glioblastomas [88]. Also, it was reported that TRPV1 suppresses skin carcinogenesis [89]. These results suggest that TRPV1 might function as a tumor suppressor, and down-regulation of TRPV1 mRNA expression may represent an independent negative prognostic factor for cancer patients. Moreover, TRPV1 has been proposed to be involved in cancer pain transduction [84,90]. TRPV2 exhibits 50% sequence identity to TRPV1 and is activated by noxious heat (> 53 °C) [91]. TRPV2 is also expressed in sensory neurons, as well as in other tissues, including smooth muscle cells and GI tract [12]. Overexpression of TRPV2 has been reported in several cancers and cancer cell lines [92]. In prostate cancer, TRPV2 expression levels are higher in metastatic cancers (stage M1) compared with primary solid tumors (stages T2a and T2b). This property makes TRPV2 a potential marker for aggressive advanced prostate cancer [93]. TRPV2 was also demonstrated to promote prostate cancer cell migration by regulating the expression of invasive enzymes MMP2, MMP9, and cathepsin B [93]. In liver cancers, TRPV2 demonstrates high expression levels in human hepatocarcinoma cells (HepG2) [94]. Expression of TRPV2 at both the mRNA and protein levels was also shown to be increased in cirrhotic livers compared with chronic hepatitis, suggesting that it might be a prognostic marker of patients with hepatocellular carcinoma [95]. Moderately and well-differentiated liver tumors were also characterized by the increased TRPV2 expression compared with that of poorly differentiated tumors [95]. In bladder cancer, enhanced TRPV2 mRNA and protein expression was found in high-grade urothelial carcinoma specimens and cell lines. Both the full-length TRPV2 and a short splice-variant were detected in normal human urothelial cells and normal bladder specimens, whereas a progressive loss of short-TRPV2 accompanied by a marked increase of full-length TRPV2 expression was found in high-grade urothelial carcinoma tissues [96]. TRPV2 mRNA was reported to be abundantly expressed in a poorly differentiated urothelial carcinoma T24 cells. Moreover, the TRPV2 agonist cannabidiol induced urothelial carcinoma cell death via apoptosis, suggesting that TRPV2 could serve as a potential therapeutic target for the treatment of bladder cancer [97]. In contrast, TRPV2 mRNA and protein expression progressively decline in high-grade glioma tissues as histological grade increases compared to benign astrocyte tissues [98]. Furthermore, TRPV2 channel negatively controls glioma cell survival and proliferation [98]. TRPV6 channel was first identified as a channel-like transporter mediating intestinal calcium absorption in the duodenum [99]. TRPV6 is expressed in a variety of tissues with predominant expression in the duodenum, jejunum, colon, and kidneys. The expression of TRPV6 is substantially increased in prostate cancer tissue as well as human carcinomas of the colon, breast, thyroid, and ovary (see Ref. [100] for review). In prostate cancer, TRPV6 expression correlates with tumor grade. TRPV6 mRNA levels are elevated in prostate cancer specimens in comparison to benign prostatic hyperplasia (BPH) specimens and positively correlate with the Gleason grade [101,102]. Thus, TRPV6 has been suggested as a prognostic marker and a promising target for new therapeutic strategies to treat advanced prostate cancer [101,102]. TRPV6 was also shown to be directly involved in the control of LNCaP cell proliferation, as siRNA-mediated TRPV6 silencing slowed down the proliferation rate, decreased the accumulation of LNCaP cells in the S phase of the cell cycle, and lowered the expression of the proliferating cell nuclear antigen [103]. An elevated expression of TRPV6 was also detected in colon carcinoma cells, where siRNA-mediated TRPV6 knockdown inhibited proliferation and induced apoptosis [104]. TRPV6 is mainly overexpressed in the invasive breast cancer cells and the selective silencing of TRPV6-inhibited human prostate carcinoma MDA-MB-231 cell migration and invasion, as well as MCF-7 migration [105]. Breast cancer cell proliferation was also reported to be regulated by TRPV6 [106]. Thus, it seems that in many cancers TRPV6 represents an oncogene; however, this is not true for all cancers. Indeed, no TRPV6 up-regulation was observed in pancreatic cancer. Furthermore, TRPV6 has been demonstrated to mediate capsaicin-induced apoptosis in gastric cancer cells [107]. TRPC1 is a nonselective ubiquitously expressed cation channel [108]. TRPC1 has been proposed to be involved in store-operated calcium entry in cooperation with Orai1 and STIM1 [109,110]. TRPC1 contributes to multiple important physiological processes and provides an important route for agonist-, growth factor-, and protein kinase C (PKC)-induced Ca2 + entry in a variety of cell types (see Ref. [12] for review). TRPC1 has been also shown to have a role in cancer cell migration and proliferation. Thus, the TRPC1 channel was reported to be essential for glioma cell chemotaxis in response to stimuli, such as Epidermal growth factor (EGF) [111]. Another report has shown that TRPC1 depletion induced cell growth arrest by causing incomplete cytokinesis in gliomas [112]. Further, it was suggested that extracellular signal-regulated kinases 1 and 2 and TRPC1 channels are required for calcium-sensing receptor-stimulated MCF-7 breast cancer cell proliferation [113]. The inhibitory effect of TRPC1 depletion on proliferation was also demonstrated in non-small-cell lung carcinoma cells [114]. TRPC1 has been also associated with proliferative phenotype in breast cancer and was demonstrated to be overexpressed in human breast cancer epithelial primary culture [105]. Recently, the role of TRPC1, C3, C4, and C6 channels in lung and ovarian cancers was demonstrated [115,116]. The expression of TRPC1, C3, C4, and C6 was correlated to the differentiation grade of both non-small-cell lung cancer and ovarian cancer. Moreover, siRNA-mediated knockdown of TRPC1, C3, C4, or C6 channels inhibited cell proliferation in both cancers [115,116]. Recently, a functional expression of TRPC3 has been demonstrated in MCF-7 breast cancer cells. Here, inhibition of TRPC3 by exogenous polyunsaturated fatty acids consistently attenuated breast cancer cell proliferation and migration [117]. Also, high levels of TRPC3 expression in tumor cells have been proposed as an independent predictor of a better prognosis in patients with adenocarcinoma of the lung [118]. The role of another member of TRPC subfamily, TRPC6, has been described in multiple cancers, including prostate [119,120], liver [121], renal [122], and breast cancer [123,124]. Thus, alpha1-adrenergic receptor signaling was reported to require the coupled activation of TRPC6 channels and nuclear factor of activated T-cells (NFAT) to promote proliferation of primary human prostate cancer epithelial cells, thereby suggesting TRPC6 as a novel potential therapeutic target [119]. In liver, TRPC6 expression levels were higher in tumors compared to normal hepatocytes. Moreover, TRPC6 overexpression stimulated proliferation of the human hepatoma cell line Huh-7 [121]. Expression of TRPC6 was demonstrated to be markedly increased in renal cell carcinoma specimens and was associated with renal cell carcinoma histological grade. The positive regulatory role of TRPC6 in proliferation of renal cell carcinoma ACHN cells has been also emphasized [122]. In breast carcinoma specimens, TRPC6 mRNA and protein were found to be elevated in comparison to normal breast tissue. However, no correlation was found between TRPC6 protein levels and tumor grades or metastases [124]. Moreover, activation of TRPC6 significantly reduced the growth and viability of the breast cancer cell lines [123]. Recently, it was reported that TRPC6 promotes cancer cell migration in head and neck squamous cell carcinomas (HNSCC) [125] and glioblastoma [126]. The increase in TRPC6 levels was demonstrated in HNSCC tumor samples and cancer cell lines. siRNA-mediated knockdown of TRPC6 expression in HNSCC-derived cells dramatically inhibited HNSCC-cell invasion but did not significantly alter cell proliferation [125]. In glioblastoma, TRPC6 has been shown to be required for the development of the aggressive phenotype because knockdown of TRPC6 expression inhibits glioma growth, invasion, and angiogenesis [126]. It’s now clear that TRP channels play an important role in cancer-related processes, including proliferation, differentiation, apoptosis, migration, and angiogenesis. Thus, it’s not surprising that a number of TRP channels have been proposed as potential anticancer therapeutic targets and/or diagnostic tools. Recently, synthetic vanilloid arvanil was proposed as therapeutics for high-grade astrocytoma [127]. By activating the ER-localized TRPV1 channel, which is significantly overexpressed in this type of cancer cells, it induces ER-stress thereby promoting tumor cell death [127]. In 2009, the small molecule TRPM8 agonist D-3263 was presented as a potential treatment for BPH alone or in combination with finasteride, a current treatment for BPH. Further, high-affinity TRPM8 agonists WS-12 and WS-12F (WS-12 compound with incorporated fluorine) were proposed as potential drugs for prostate cancer imaging and therapy [128]. Moreover, it has been suggested that incorporation of a radioisotope into WS-12 could allow radiodiagnostics as well as radiotherapy of prostate cancer with all the advantages of selective targeting of TRPM8-expressing cells [128]. At present, there is a huge need in selective pharmacological modulators for most of the TRP channels, and there are no doubts that design of such specific molecules will greatly advance the field toward practical clinical implications of the TRP channel-based anticancer therapies. Given that calcium overload is detrimental to all cells, prolonged stimulation or overexpression of calcium-permeable channels in cancer cells will lead to substantial increase in cytoplasmic calcium followed by apoptotic/necrotic cell death [129]. In this respect, all the calcium-permeable TRP channels could potentially represent efficient anticancer therapeutic tools, provided that specific approaches will be implemented for selective overexpression (under control of tissue/cell type specific promoter) or targeting of the channel of interest. Indeed, most of the TRP channels have broad expression patterns and thus are not cancer-specific. Therefore, selective targeting is vitally important to prevent toxicity to normal cells induced by the pharmacological impairment of channel function. Given that the expression level of certain TRP channels differs substantially between normal and cancerous tissues, these channels could be considered as potential diagnostic markers. For example, TRPV6 expression correlates with tumor grades in many tissues, which has led to the suggestion that TRPV6 represents a reliable tool to predict the clinical outcome of different cancers [100]. Nevertheless, further studies are needed to find out whether TRPV6 represents an oncogenic ion channel, or its expression is epigenetically altered during cancer progression. Furthermore, inverse correlation between TRPM1 mRNA expression and melanoma metastatic potential suggests that TRPM1 could serve as a diagnostic/prognostic marker for the development and progression of melanomas [27]. Expression levels of TRPM8 have been also reported to be useful when staging prostate cancer [70,72]. Other TRP channels, including TRPM7, TRPV1, TRPV2, TRPC1, and TRPC3, also show a great potential as diagnostic/prognostic markers in different cancers. However, it should be noted that the use of ion channels for diagnostic purposes involves tissue biopsies, as cellular ion channels normally cannot be detected in blood samples. Thus, the antibody-based approaches should be considered. Indeed, TRP channels localized on plasma membrane could be subjected to antibody-based targeting that can be particularly useful in the case of channel up-regulation in cancer. Fluorescently labeled antibodies could be used for tumor visualization, whereas radionuclide-labeled antibodies provide a powerful therapeutic tool [8]. Importantly, as the contribution of specific TRP channels to cancer-related processes could vary depending on cancer type and stage, the specific therapeutic/diagnostic approach should be implemented for each particular case. Thus, the targeting of TRP channels in cancer treatment should not be regarded as a general approach to treat cancer, but rather as a specific tool for individualized cancer therapy. Overall, TRP channels have emerged as promising anticancer therapeutic targets and diagnostic/prognostic markers. However, despite the essential progress achieved in this field, there is still a need in highly selective pharmacological modulators, novel drug delivery techniques, as well as siRNA transfection technologies to selectively target TRP channels in cancer cells without significant adverse effects.
TRP Channels in Cancer
* Corresponding author: Natacha.Prevarskaya@univ-lille1.fr
Abstract
Introduction
The Role of TRP Channels in Cancer
TRPM Channels
TRPV Channels
TRPC Channels
Clinical Implications and Concluding Remarks
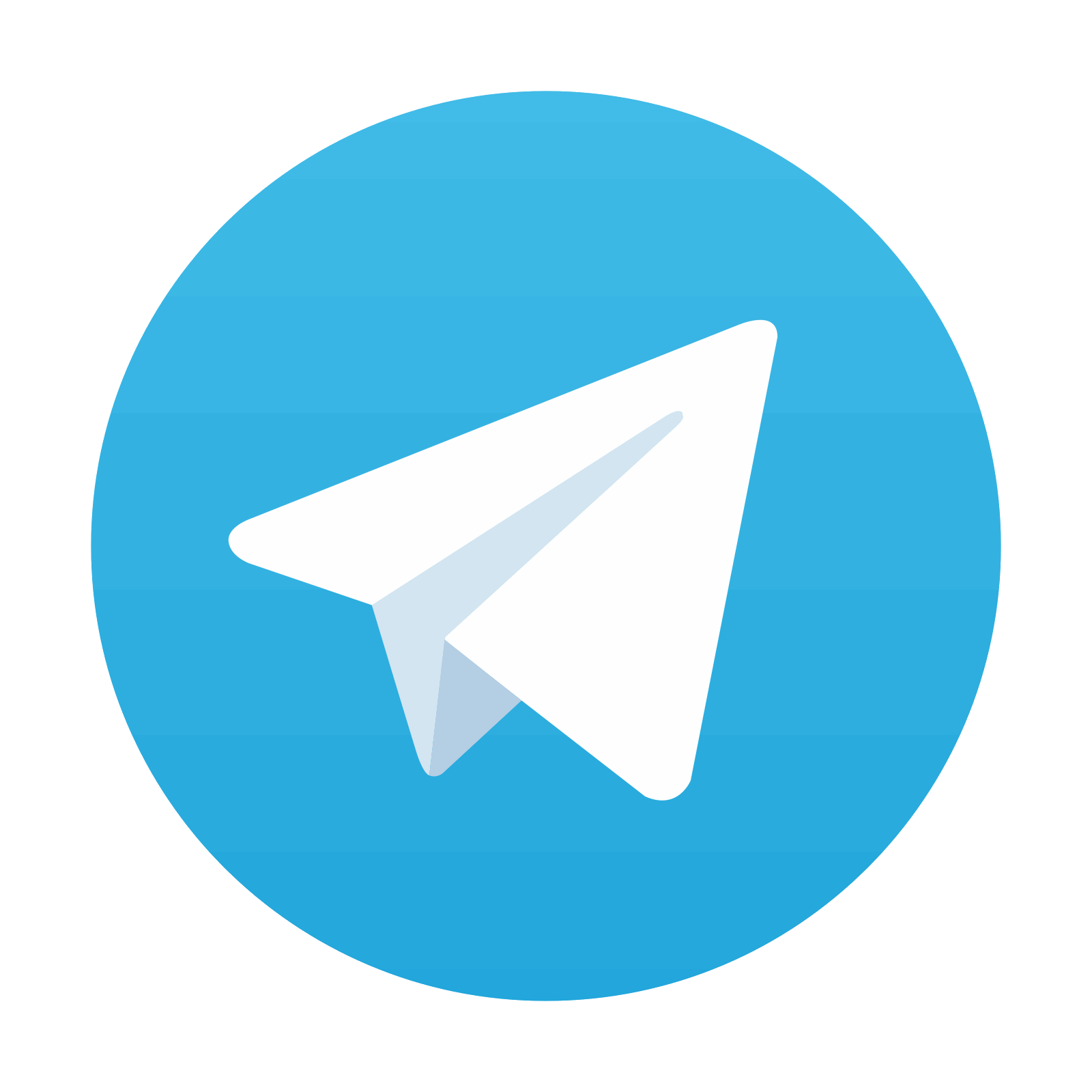
Stay updated, free articles. Join our Telegram channel

Full access? Get Clinical Tree
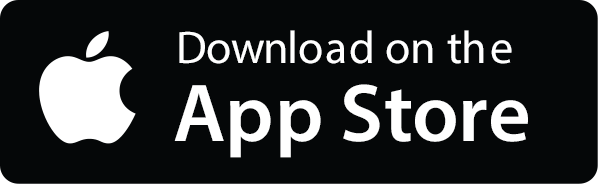
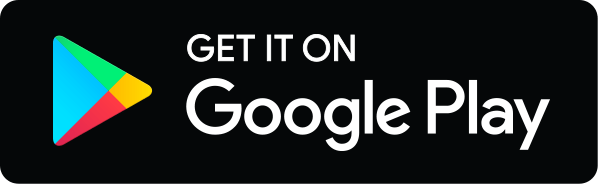